KEY POINTS
While some data suggest a decrease in the number of asthmatics requiring intubation and mechanical ventilation in recent years, all aspects of the management of severe asthma should be mastered by the intensivist, including optimizing mechanical ventilation in the face of large increases in airway resistance and propensity for dynamic hyperinflation.
Severe asthma exacerbation is defined by several, but not necessarily all, of the following features: dyspnea at rest, upright positioning, inability to speak in phrases or sentences, respiratory rate >30 breaths per minute, use of accessory muscles of respiration, pulse >120 beats/min, pulsus paradoxus >25 mm Hg, peak expiratory flow rate <50% predicted or personal best, hypoxemia, and eucapnia or hypercapnia.
Altered mental status, paradoxical respirations, bradycardia, a quiet chest, and absence of pulsus paradoxus from respiratory muscle fatigue identify imminent respiratory arrest.
Airway wall inflammation, bronchospasm, and intraluminal mucus cause progressive airflow obstruction. Fewer patients develop sudden-onset asthma from a more pure form of bronchospasm.
Airflow obstruction causes ventilation-perfusion inequality, lung hyperinflation, and increased work of breathing.
Oxygen, β-agonists, and systemic corticosteroids are first-line treatments. Second-line treatments include ipratropium bromide, magnesium sulfate, leukotriene modifiers, theophylline, inhaled steroids, and heliox.
Noninvasive ventilation is potentially useful in hypercapnic patients not requiring intubation.
Postintubation hyperinflation decreases right heart preload and results in tamponade physiology. This may present as tachycardia, hypotension, and even cardiac arrest. A ventilator strategy that lowers lung volume decreases these potential complications.
Treating airflow obstruction and prolonging the expiratory time during mechanical ventilation decreases lung hyperinflation. Expiratory time is prolonged by lowering minute ventilation and increasing inspiratory flow rate.
Deep sedation allows for safe and effective mechanical ventilation in most intubated patients. Paralysis increases the risk of complications.
Patient education, environmental control measures, and use of controller agents help prevent future exacerbations.
Asthma is characterized by wheezing, dyspnea, cough, hyperreactive airways, airway remodeling, and reversible airflow obstruction.1 In the United States, it has a prevalence of just over 8.0% and is responsible for approximately 1.75 million emergency department (ED) visits, 450,000 hospitalizations and 3500 deaths.2 Underestimation of severity, poor communication between the health care provider and the patient, and failure to use a controller agent all contribute to morbidity and mortality.3-7 While some studies indicate the incidence of respiratory failure secondary to status asthmaticus requiring intubation and mechanical ventilation is falling,8 it is essential the intensivist become familiar with the full spectrum of acute asthma, be able to determine the stage and progression of this process, learn means to halt the progression of this syndrome, and to sustain patients who require mechanical ventilation safely until underlying airway disease responds to treatment.
The objective of this chapter is to review the pathophysiology, assessment, and management of patients with severe asthma exacerbation, which is signaled by many, but not necessarily all of the following features: resting dyspnea, upright positioning, monosyllabic speech, respiratory rate >30 bpm, accessory muscle use, pulse >120/min, pulsus paradoxus >25 mm Hg, peak expiratory flow rate <40% of predicted or personal best, minimal or no relief from short-acting β-agonists, hypoxemia, and eucapnia or hypercapnia.1 Altered mental status, paradoxical breathing, bradycardia, a quiet chest, and absence of pulsus paradoxus from respiratory muscle fatigue identify imminent arrest.
PATHOPHYSIOLOGY
Typical asthma exacerbations often evolve over hours to days in response to infections, irritants, allergens, or air pollution.9,10 While this allows for ample time to intervene early with systemic corticosteroids, many patients rely on increasing doses of inhaled β-agonists, eventually to no avail. These patients invariably have airway inflammation and mucus plugs that can be quite striking on postmortem analysis.11 A smaller subset of patients develop sudden-onset attacks that appear to stem from a more pure form of smooth muscle–mediated bronchospasm. While these attacks can be lethal, they can also respond quickly to bronchodilators.10,12,13 Triggers of sudden attacks include allergen and irritant exposures, exercise, stress, sulfites, use of nonsteroidal anti-inflammatory agents and β-blockers in susceptible patients, and inhalation of crack cocaine or heroin.14-17 Infections are not a common trigger of sudden-onset attacks.18 However, during pandemics such as those resulting from H1N1 influenza, large numbers of patients with exacerbations of asthma may be encountered and appropriate treatment algorithms for documented pathogens should be applied.19
Airway obstruction causes ventilation-to-perfusion (˙V/˙Q) mismatch. Intrapulmonary shunting is trivial, so modest enrichment of oxygen (eg, 1-3 L/min by nasal cannula) generally corrects hypoxemia.20 Refractory hypoxemia is rare and suggests other conditions such as pneumonia, atelectasis, or pneumothorax. Hypoxemia correlates with the forced expiratory volume in 1 second (FEV1) and peak expiratory flow rate (PEFR); however, there is no cutoff value for spirometry that accurately predicts hypoxemia.21,22 Airflow rates commonly increase before oxygenation in improving patients, possibly because large airways recover quicker than smaller airways.23,24
Supplemental oxygen improves oxygen delivery to tissues, including the exercising respiratory muscles. It also protects against β-agonist–induced hypoxemia resulting from pulmonary vasodilation and increased blood flow to low ˙V/˙Q units.25,26
Respiratory alkalosis is common in early and mild attacks. If present for many hours to days, there is compensatory renal bicarbonate wasting that may subsequently manifest as a normal anion-gap metabolic acidosis (ie, posthypocapnic metabolic acidosis). As the severity of airflow obstruction increases, the partial pressure of arterial carbon dioxide (PaCO2) generally increases as well due to inadequate alveolar ventilation (reflecting a decrease in minute ventilation as the patient nears respiratory arrest) and possible elevated CO2 production from increased work of breathing. Hypercapnia usually does not occur unless the FEV1 is less than 25% of predicted.21 Increase in dead space might also occur if hyperinflated lung limits blood flow to create West’s zone 1 conditions (where alveolar pressure exceeds pulmonary capillary pressure).23 However, multiple inert gas elimination technique (MIGET) analysis demonstrates only small areas of high ˙V/˙Q and slightly increased dead space in acute asthma.20,26 Importantly, the absence of hypercapnia does not preclude a severe attack or impending arrest.22
Incomplete exhalation and the formation of positive end-expiratory alveolar pressure are hallmarks of the tachypneic patient with expiratory airflow obstruction.27 In ventilated patients, end-expiratory alveolar pressure is not reflected at the airway opening if the expiratory port of the ventilator is open (which allows airway-opening pressure to approach atmospheric pressure or the level of ventilator-applied positive end-expiratory pressure [PEEP]). If the expiratory port is closed at end expiration, central airway pressure generally equilibrates with alveolar pressure, permitting measurement of intrinsic PEEP (PEEPi), which is also referred to as auto-PEEP. This measurement is most accurate in sedated and/or paralyzed patients, since expiratory muscle contraction elevates end-expired pressure. Importantly, however, PEEPi can underestimate the degree of lung hyperinflation in patients with poorly communicating airspaces.28
The pressure-volume relationship of the lung demonstrates that lung hyperinflation decreases static compliance. However, lung compliance may be normal despite hyperinflation, suggesting a stretch-relaxation response in parenchymal tissue.29 This state is not favorable for expiratory flow, but may protect against complications of lung hyperinflation.
Circulatory abnormalities reflect a state of cardiac tamponade resulting from dynamic hyperinflation (DHI) and pleural pressure changes associated with breathing against obstructed airways. During expiration, elevated intrathoracic pressures decrease right-sided filling. Vigorous inspiration augments right ventricular filling and shifts the intraventricular septum leftward to cause a conformational change in the left ventricle (LV), diastolic dysfunction, and incomplete LV filling. Additionally, large negative pleural pressures directly impair LV emptying, which under extreme conditions can even cause pulmonary edema.30,31 Finally, lung hyperinflation increases RV afterload and may cause transient pulmonary hypertension.32 The net effect of these cyclical events is to accentuate the normal inspiratory reduction in stroke volume, a phenomenon termed pulsus paradoxus (PP). Pulsus paradoxus is a marker of asthma severity33; however, the absence of a widened PP does not ensure a mild attack.34 The PP falls in improving patients, but also in the fatiguing asthmatic no longer able to generate large swings in pleural pressure.
Several pathophysiologic mechanisms appear to be responsible for ventilatory failure in acute asthma. Intrinsic PEEP is a threshold pressure that must be overcome before inspiratory flow occurs, increasing inspiratory work of breathing. Increased airway resistance and decreased lung compliance further increase work.
Increased mechanical loads are placed on a diaphragm that is placed in a disadvantageous position by lung hyperinflation, and at the same time circulatory abnormalities may result in hypoperfusion of the exercising respiratory muscles. In the end, strength is inadequate for load and hypercapnia ensues, which further decreases diaphragm force generation.35,36
CLINICAL PRESENTATION, DIFFERENTIAL DIAGNOSIS, AND ASSESSMENT OF SEVERITY
Multifactorial analysis including the history, physical examination, measures of airflow obstruction, response to therapy, and in selected patients arterial blood gases and chest radiography is required to assess severity and the risk for deterioration.37
Characteristics of prior exacerbations that predict a fatal or near fatal attack include intubation, hypercapnia, barotrauma, hospitalization despite corticosteroids, psychiatric illness, and medical noncompliance.1,9,38-40 Substance abuse, alcohol ingestion, and excessive, long-term use of β-agonists are also associated with mortality.16,41 Pharmacogenetic studies have suggested an association between polymorphisms of β-adrenoreceptors, the severity of asthma, and its response to therapy.42 Survivors of near-fatal asthma attacks may also have diminished ventilatory drive in the face of hypoxemic or mechanical stimuli (ie, poor perceivers of airflow obstruction).43 Other concerning features include symptoms of long duration (which suggest a substantial component of inflammation and slow recovery), late arrival for care, fatigue, altered mental status, and sleep deprivation. Deterioration despite optimal treatment, including the concurrent use of oral steroids, further identifies patients at risk.
“All that wheezes is not asthma” is a valid clinical saw to consider. The absence of a history of asthma should alert the physician to other diagnoses (although asthma can first occur at any age). A history of smoking suggests chronic obstructive pulmonary disease (COPD), which may be associated with fixed airflow obstruction and chronic respiratory acidosis. Cardiac asthma refers to the airway hyperreactivity that occurs in congestive heart failure.44 Heart failure is generally discernible by examination, but the distinction between LV dysfunction and airway obstruction can occasionally be difficult. As discussed above, severe airflow obstruction is a rare cause of pulmonary edema, and bronchodilators may improve airflow obstruction in LV failure.45 Foreign body aspiration must be considered in children and at-risk adults. Upper airway obstruction from granulation tissue, tumor, laryngeal edema, or vocal cord dysfunction is in the differential, but classic extrathoracic obstructions cause inspiratory phase (not expiratory phase) prolongation and stridor.46 In these cases, fiberoptic laryngoscopy may be indicated to confirm a laryngeal level process. For patients with tracheal stenosis (eg, from prior intubation), CT imaging and fiberoptic bronchoscopy establishes the diagnosis. Important clues to focal obstruction include localized wheeze, and rarely, asymmetric hyperinflation on the chest radiograph. Pneumonia complicating asthma is unusual but should be considered when there is fever, purulent sputum, localizing signs, and refractory hypoxemia. In large series of patients with pulmonary embolus, wheezing was not a reported sign. However, wheezing has been described anecdotally,47 and dyspnea out of proportion to measures of airflow obstruction should prompt the consideration of pulmonary embolus.
The general appearance of the patient (ie, posture, speech pattern, positioning, and mental status) allows for quick assessment of severity, response to therapy, and need for intubation. Adults with acute asthma who assume the upright position have a higher heart rate (HR), respiratory rate (RR), and PP and a significantly lower partial pressure of arterial oxygen (PaO2) and PEFR than patients who are able to lie supine.48 Diaphoresis is associated with an even lower PEFR. Accessory muscle use and PP indicate severe airflow obstruction, but the absence of either does not rule out severe obstruction.34
Examination of the head and neck should focus on identifying barotrauma and upper airway obstruction. Tracheal deviation, asymmetric breath sounds, a “mediastinal crunch,” and subcutaneous emphysema suggest pneumothorax or pneumomediastinum. The mouth and neck should be inspected for mass lesions or signs of previous surgery including tracheostomy. The lip and tongue should be inspected for angioedema.
Wheezing correlates poorly with the degree of airflow limitation.49 Severe airflow obstruction may present with poor air movement and a silent chest; in this situation the emergence of wheezing signals improved air entry and clinical improvement. Localized wheezing or crackles should prompt consideration of atelectasis, pneumonia, pneumothorax, endobronchial lesions, or foreign body.
Sinus tachycardia is common, but supraventricular and ventricular arrhythmias occur.50 Bradycardia is an ominous sign of impending arrest.51
Clinical signs of right-sided and left-sided heart failure suggest primary cardiac disease. Yet, acute asthma alone can cause examination and electrocardiographic findings of transient right-sided strain and rarely pulmonary edema.52,53 Jugular venous distention also occurs when DHI or tension pneumothorax limit venous return to the right heart. Asthma can also cause acute coronary syndrome in at-risk patients. Large drops in intrathoracic pressure increase LV afterload and decrease coronary blood flow, which can cause an imbalance between myocardial oxygen supply and demand.54 β-agonists, theophylline, and hypoxemia may further disrupt this balance.
The degree of airflow obstruction can be determined by measuring PEFR or FEV1. A PEFR or FEV1 <40% of predicted or the patient’s personal best characterizes severe exacerbation.1 Objective measurements are generally safe to obtain except in the sickest patients, and may provide important information because physician estimates are often wrong.55 In critically ill patients, it is wise to defer measurements, which may worsen bronchospasm56 and even precipitate an arrest.57
Measurement of the change in PEFR or FEV1 helps in the assessment of treatment response. Several studies have demonstrated that failure of initial therapy to improve expiratory flow after 30 minutes predicts a refractory course and need for hospitalization or continued treatment in an ED.37,58-60 Changes in PEFR before 30 minutes of treatment have elapsed do not predict outcome.61
Pulse oximetry should be performed at the time of arrival to the ED and monitored until there is a clear response to therapy. Supplemental oxygen is recommended to maintain arterial oxygen saturation at greater than 90% (>95% in pregnant women and patients with coronary artery disease).62
When FEV1 is less than 25% predicted or of the patient’s personal best, an arterial blood gas should be considered. In early (mild) acute asthma, hypoxemia and respiratory alkalosis are common. Hypercapnia signals a severe attack, but in and of itself is not an indication for intubation.63 Conversely, hypercapnia is not always present in cases of severe obstruction and impending respiratory arrest.22
Metabolic acidosis with a normal anion gap occurs when there has been bicarbonate wasting in response to respiratory alkalosis. An elevated anion gap suggests excess serum lactate, possibly secondary to increased work of breathing, tissue hypoxia, intracellular alkalosis, or decreased lactate clearance by the liver. Lactic acidosis correlates with the severity of airflow obstruction, is more common in men, and occurs more frequently when β-agonists are administered parenterally.64
Serial blood gases are usually not necessary to determine clinical course. Physical examinations and peak flows allow for valid clinical assessments in most cases. Patients who deteriorate on clinical grounds should be considered for intubation regardless of PaCO2. Conversely, improving patients should not be intubated despite hypercapnia. Serial blood gases are helpful in intubated patients to guide ventilator management.
Chest radiography plays little role in the assessment or management of routine patients. Even in hospitalized patients, radiographic findings influence treatment in 1% to 5% of cases.65-68 In one study68 that reported major radiographic abnormalities in 34% of cases (which the authors felt impacted management), the majority of findings were classified as focal parenchymal opacities or increased interstitial markings, common indicators of atelectasis in asthma. Chest radiography should be reserved for patients suspected of having heart failure, pneumothorax, pneumonia, or atelectasis. In mechanically ventilated patients, chest radiography further identifies endotracheal tube position.
Patients demonstrating a good response to initial therapy in the ED may be discharged home with close follow-up. There should be significant improvement in breathlessness, improved air movement on physical examination, and a FEV1 or PEFR ≥70% of predicted or personal best.1 Observation for 60 minutes after the last β-agonist dose helps ensure stability prior to discharge. Written medication instructions and an asthma action plan should be provided. In general, patients should be discharged on oral corticosteroids. Inhaled corticosteroids (ICSs) should be continued (or even initiated) in the ED and follow-up appointments should be made.
Patients with severe airflow obstruction demonstrating a poor response to initial therapy and patients who deteriorate despite therapy should be admitted to an ICU. Other indications for ICU admission include respiratory arrest, altered mental status, hypercapnia, arrhythmias, acute coronary syndrome, and need for frequent inhaler treatments.
An incomplete response to treatment is defined as the persistence of mild wheezing or dyspnea and a PEFR or FEV1 between 50% and 69% of predicted.1 Patients in this group should be assessed individually, and while selected low-risk patients may be safely discharged from the ED, others require ongoing treatment in either the ED or the medical ward. Extended ED evaluation allows for assessment of the initial response to systemic corticosteroids, and for those who are discharged home decreases the risk of relapse and return to the ED.34 If the patient demonstrates a good response to treatment over that period of time and close follow-up can be arranged, discharge home may be appropriate. Again, these patients have better outcomes if they receive a course of oral steroids.69 Patients who continue to have an incomplete response should be admitted to a medical ward. Admission is also recommended when there is a harmful home environment or medical noncompliance.
THERAPY PRIOR TO INTUBATION
β-Agonists: Inhaled short-acting β-agonists (SABAs) are the cornerstone of treatment of smooth muscle–mediated bronchoconstriction and should be given immediately regardless of prior use (Table 55-1).70 Albuterol is the most widely used SABA, but others are available including levalbuterol, bitolterol, and pirbuterol. Levalbuterol in one-half the milligram dose of albuterol provides comparable efficacy and safety by metered dose inhaler (MDI), but has not been studied by continuous administration.62
Drugs Used in the Initial Treatment of Acute Severe Asthma
Albuterol: 2.5 mg by nebulization every 20 minutes or four to eight puffs by MDI with spacer every 20 minutes; for intubated patients titrate to physiologic effect. Alternative: levalbuterol (see text) |
Epinephrine: 0.3 mL of a 1:1000 solution subcutaneously every 20 minutes × 3. Terbutaline is favored in pregnancy when parenteral therapy is indicated. Use with caution in patients >age 40 and in the presence of cardiac disease |
Ipratropium bromide: 0.5 mg combined with albuterol by nebulization every 20 minutes or four to eight puffs by MDI with spacer combined with albuterol every 20 minutes |
Corticosteroids: Methylprednisolone 40 mg IV every 12 hours or prednisone PO 40 mg every 12 hours |
Magnesium sulfate: 2 g IV over 20 minutes, repeat in 20 minutes if clinically indicated (total 4 g unless hypomagnesemic) |
Leukotriene modifiers: Consider montelukast 10 mg PO daily |
Guidelines recommend repetitive or continuous administration depending on clinical response and side effects. A common strategy is to give albuterol 2.5 mg by nebulization every 20 minutes during the first hour of ED management.62 Continuous administration (at the same total dose) may be slightly superior to repetitive dosing in patients with severe exacerbations, although there is little difference between these two strategies in most cases.71-74 Albuterol can also be delivered effectively by MDI with a spacer; four to eight puffs of albuterol by MDI with spacer is equivalent to a 2.5-mg nebulizer treatment.75,76 MDIs with spacers are less expensive and faster, but handheld nebulizers require less supervision and coordination. Fortunately, frequent doses of β-agonists are generally well tolerated. This may relate to the fact that in the most severely obstructed patients who would be receiving higher doses of β-agonists, drug delivery is most impaired resulting in minimal systemic absorption and effect. In one study, albuterol delivered by MDI with spacer to a total dose of 1600 µg over 90 minutes was not associated with increased cardiovascular morbidity in well-oxygenated patients.77 After the first hour, dosing depends on clinical response and side effects.
Long-acting β-agonists (LABAs) are not recommended for treatment of acute asthma, although limited data do show that formoterol (which has quick onset of action) is effective and safe in this setting. Combination therapy with a LABA and an ICS may be initiated or continued in hospitalized patients receiving rescue therapy,78 and may be required to achieve adequate outpatient control and decrease the risk of future exacerbations.
Subcutaneous β-agonists are not recommended unless the patient is unable to comply with inhaled therapy (such as those with an altered mental status or impending cardiopulmonary arrest). They are no more effective in the initial management of acutely ill asthmatics and are associated with greater toxicity.79-81 However, subcutaneous epinephrine may benefit some patients not responding to several hours of an inhaled β-agonist.82 Known cardiac disease and age >40 years are relative contraindications to parenteral therapy.83 Intravenous infusions of β-agonists are not recommended because they are more toxic and less efficacious than inhaled treatments.84-88
Approximately two-thirds of patients respond to inhaled albuterol in a convincing dose-dependent fashion, generally allowing discharge home from the ED.89 In these patients, 1.2 to 2.4 mg albuterol delivered by MDI and spacer or 5 to 7.5 mg by nebulizer in the first hour is effective. In the remaining one-third of patients albuterol has minimal effect, presumably because airway inflammation and mucus plugging adversely affects the dose response relationship.
Ipratropium Bromide: Overall, the data suggest an advantage in maximal bronchodilation response when ipratropium bromide and albuterol are combined in the initial emergency treatment of asthma.90-100 However, several studies that generally used small doses of ipratropium bromide showed little or no benefit to this combination,101-105 and the addition of ipratropium bromide has not been shown to provide further benefit once the patient is hospitalized.62
Combination therapy is recommended for patients critically ill on first presentation or not responding quickly (eg, within 30 minutes) to albuterol alone. The Expert Panel of the NIH recommends adding 0.5 mg of ipratropium bromide to 2.5 mg of albuterol by nebulizer every 20 minutes for three doses, then as needed. Alternatively combination of four to eight puffs of ipratropium bromide MDI and four to eight puffs of albuterol (or eight puffs of a combination albuterol/ipratropium bromide inhaler) can be given every 20 minutes for the first 1 to 3 hours as guided by clinical response and toxicity.62
Corticosteroids: Systemic corticosteroids should be administered quickly to patients not responding in an immediate, marked, and durable manner to initial bronchodilator therapy, particularly since benefits are not immediately evident. Indeed McFadden’s group demonstrated no differences in physiologic or clinical variables in the first 6 hours in 38 patients receiving hydrocortisone.105 Rodrigo and Rodrigo similarly showed that early administration of steroids did not improve spirometry in the first 6 hours.106 However, Littenberg and Gluck demonstrated that methylprednisolone 125 mg IV on arrival decreased admission rates compared to placebo,107 and Lin and colleagues demonstrated improved peak flows after 1 and 2 hours of methylprednisolone.108 A systematic analysis for the Cochrane Review demonstrated that corticosteroids within 1 hour of arrival in the ED reduced admissions.109,110 Systemic steroids reduce the number of relapses and the risk of death.110-113 In hospitalized patients, they speed the rate of improvement.114 Oral and intravenous routes are equally effective,115 but oral steroids should be avoided if there is concern regarding the need for intubation.
There is no clear dose-response relationship to steroids in acute asthma.116,117 In one meta-analysis by Manser and colleagues, there was no difference in clinical outcomes between low-dose corticosteroids (≤80 mg/d methylprednisolone or ≤400 mg/d hydrocortisone) and higher doses in the initial management of hospitalized asthmatics.118 Haskell and colleagues demonstrated that 125 mg IV methylprednisolone every 6 hours resulted in faster improvement compared to 40 mg every 6 hours, but there was no difference in peak improvement.119 Both doses were superior to 15 mg every 6 hours in terms of rate and absolute response. Emerman and Cydulka compared 500 mg and 100 mg of methylprednisolone and found no benefit to the higher dose.120
The Expert Panel from the National Institutes of Health (NIH) recommends 40 to 80 mg/d of prednisone or methylprednisolone in one or two divided doses for all patients with moderate to severe exacerbations until PEFR reaches 70% predicted or personal best.62 Prednisone is tapered at variable rates depending on a number of factors, including PEFR, the duration of high-dose therapy required to treat the acute exacerbation, and whether oral steroids had been used for maintenance therapy. Automatic tapering schedules are not recommended because patients may taper prematurely.
Although data demonstrate efficacy of ICSs in the treatment of acute asthma,121 there is no established role for their use in most patients.62,122 However, ICSs play a pivotal role in achieving outpatient asthma control and are generally underused for this purpose. Patients discharged from the ED or hospital after an asthma attack should be considered for an ICS-based treatment program, combined with optimal education regarding ICS use.
Aminophylline: There is no benefit to adding aminophylline to inhaled β-agonists in the initial treatment of acute asthma.123 In a meta-analysis by Parameswaran and colleagues there was a trend toward higher PEFR at 12 and 24 hours, but at the cost of arrhythmias and vomiting.124 Others have reported a delayed benefit.125 Nonbronchodilating properties of aminophylline may be useful in refractory cases; indeed anti-inflammatory effects and enhanced diaphragm function may explain one report that ED administration of aminophylline decreased hospitalizations, even when airflow rates were no different than placebo.126
Aminophylline should be used sparingly in refractory patients; however, it is reasonable to continue its use in the rare patient taking theophylline as an outpatient after confirming a nontoxic serum concentration. This approach is safe if attention is paid to serum drug levels and to factors that increase levels, such as congestive heart failure, ciprofloxacin, macrolide antibiotics, and cimetidine, and if the drug is discontinued for signs and symptoms of toxicity.
Magnesium Sulfate: Three early prospective trials failed to confirm a benefit to administering magnesium sulfate (MgSO4) to asthmatics in the ED.127-129 In 135 asthmatics randomized to 2 g MgSO4 IV or placebo after 30 minutes and followed for 4 hours, admission rates and FEV1 were no different between magnesium-treated patients and controls.129 However, subgroup analysis revealed MgSO4 decreased admission rates and improved FEV1 in subjects with FEV1 <25% of predicted. Subsequently, a placebo-controlled, double-blind, randomized trial in 248 patients with FEV1 ≤30% showed a small but statistically significant increase in FEV1 after 240 minutes in the magnesium group, but no difference in hospitalization rates.130 Subsequent meta-analysis of 7 trials (5 adult, 2 pediatric) and 665 patients did not support the routine use of IV magnesium in all ED patients, but did demonstrate that magnesium was safe and beneficial in patients with severe attacks.131 A similar conclusion was reached by the authors of a systematic review that included 10 randomized trials.132 Additional evidence supporting benefit in severe disease comes from an uncontrolled study of five intubated asthmatics given magnesium.133 In this study, there was a fall in peak airway pressure (43-32 cm H2O) after high doses of MgSO4 (10-20 g) were administered over 1 hour. Other investigators have suggested that gender may play a role in magnesium responsiveness, since estrogen augments the bronchodilator effect of magnesium.134,135
Magnesium sulfate can also be administered by inhalation. Nannini and colleagues studied the effects of MgSO4 (225 mg) versus saline as the vehicle for nebulized albuterol in a randomized, double-blind fashion.136 At 20 minutes, patients treated with MgSO4 and albuterol had a greater PEFR compared to the saline-albuterol group (134 ± 70 L/min vs 86 ± 64 L/min). Hughes and colleagues published similar data.137 To the contrary, Aggarwal and colleagues reported no therapeutic benefit to adding MgSO4 to albuterol nebulization in their randomized trial of acute severe asthmatics.138 One systematic review has reported inhaled magnesium improves lung function in patients with severe attacks,139 whereas a more recent systematic review states the data are insufficient to draw strong conclusions.132 A recent Cochrane review also concluded that inhaled MgSO4

Full access? Get Clinical Tree
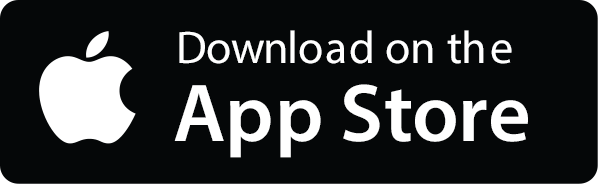
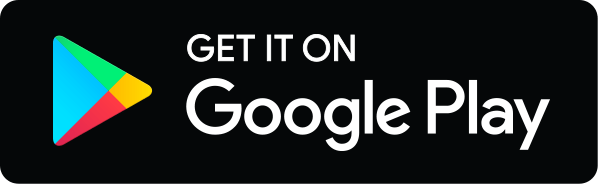
