KEY POINTS
After acute spinal cord injury (SCI), the primary injury sets limits (that are not always initially obvious) on the potential extent of recovery and the degree of secondary injury determines the extent of the potential recovery actually achieved.
Acute SCI patients, particularly those with cervical level and severe SCI, are at risk for respiratory arrest, hypoxemia, and cardiovascular instability.
The prevention of secondary injury or “neuroprotection” consists of spine immobilization, timely surgical intervention, and early recognition and treatment of hemodynamic instability, respiratory failure, and hypoxemia.
Hemodynamic instability is common after acute SCI and may be multifactorial, including due to hypovolemia secondary to blood loss, systemic inflammation from trauma or infection (vasodilation, decreased intravascular volume), neurogenic (vasodilation, inappropriate bradycardia), arrhythmias, myocardial stunning, pneumothorax, or cardiac tamponade from associated trauma.
The term “neurogenic shock” refers to hypotension due to vasodilation that may be accompanied by absolute (HR <60) or relative bradycardia caused by the loss of outflow from the sympathetic autonomic component of the spinal cord arising from the high thoracic and cervical regions, T1-T6 level and above.
The term “spinal shock” refers to the loss of sensation accompanied by motor paralysis and depression of spinal reflexes caudal to the level of acute SCI.
Among trauma patients, the risk of VTE is likely the highest after acute spinal cord injury and LMWH should be started as soon as safely possible after primary hemostasis is achieved; until that time intermittent pneumatic compression (IPC) devices should be used.
Pulmonary embolism (PE) is the third leading cause of death after acute SCI and after any sudden hemodynamic compromise, unexplained dyspnea, or hypoxemia, PE must be considered.
Patients with high cervical injury and prolonged ventilatory failure with tracheostomy are at a higher risk of malnutrition.84 The enteral route is preferred to preserve gut integrity. The current literature does not support the maintenance of strict normoglycemia in these critically ill patients.
Following spinal decompression and/or stabilization, and resolution of potentially life-threatening cardiac and respiratory events, the goal is for rehabilitation specialists to become involved early in the management.
BACKGROUND
Acute trauma to the spine may involve the neuronal (spinal cord and nerve roots) and/or skeletal and ligamentous structures of the vertebral column that normally protect the spinal cord. Vertebral fractures or dislocations can occur without damage to the spinal cord; however, acute traumatic spinal cord injury (SCI) often involves injury to the vertebral column. The manifestations of SCI may be immediate or delayed. The key to insuring the best outcome is rapid diagnosis and prevention of secondary injuries that can further worsen ischemic neurological damage. This includes rapid recognition and treatment of unstable fractures, fracture fragments, hematomas, or other lesions that can cause impingement or laceration of the spinal cord and critical care management that emphasizes maintenance of homeostasis and the detection of new or initially unrecognized injuries.
Approximately 50% of spinal injuries occur in the cervical spine, the other half involve the thoracic, lumbar, and sacral areas.1 SCI may lead to significant neurological damage, including paraplegia, tetraplegia, or death. Patients with tetraplegia have injuries to one or more of the eight cervical segments (C1-8) of the spinal cord; those with paraplegia have lesions in the thoracic (T1-12), lumbar (L1-5), or sacral (S1-2) regions of the spinal cord (Table 119-1). The American Spinal Injury Association (ASIA) has devised a scoring system to assess SCI (Fig. 119-1). Complete spinal cord injuries result in no motor or sensory preservation below the level of injury and carry a poor prognosis for functional recovery. Partial preservation of motor and or sensory function is termed incomplete injury. An incomplete injury has the potential to regain useful function or progress to complete injury.
Clinical Sequele vs Level of Spinal Cord Injury
SCI Level | Clinical Sequelae |
---|---|
C1-C2 | Tetraplegia; phrenic nerve paralysis and complete paralysis of respiratory muscles, requires permanent mechanical ventilation, diaphragmatic pacing |
C3-C4 | Tetraplegia; phrenic nerve damage and paralysis of respiratory muscles but damage at the C4 level and below allows some recovery of respiratory function |
C5 | Tetraplegia; shoulder and upper arm control preserved; loss of wrist and hand control. Diaphragmatic function preserved; paralysis of the intercostal and abdominal wall muscles supplied by the thoracic segments leads to paradoxical respiration |
C6 | Tetraplegia; wrist control preserved; loss of hand control |
C7-T1 | Tetraplegia; reduced dexterity of hands and fingers |
T1-T8 | Paraplegia; upper extremity function typically preserved; loss of abdominal muscle control |
T9-12 | Paraplegia; abdominal muscle and trunk control preserved |
L1-L5 | Paraplegia; loss of hip flexor and leg control; early but temporary loss of colonic motility; initially hypotonic bladder and external urethral sphincter; later detrusor hyperactivity with loss of external urethral sphincter control with urinary incontinence |
S1-S5 | Paraplegia; loss of foot control; chronic dysmotility; greater risks of fecal impaction; impaired motor output to bladder (S2-S4) leading to a flaccid, distended bladder |
EPIDEMIOLOGY
Acute traumatic spinal injuries occur most commonly in males (80.8%) and the average age at injury is 40.2 years, increased from 28.7 years in the 1970s mainly due to an increase in the median age of the general population.2 Excluding those who die at the accident scene, the annual incidence of (SCI) in the U.S. is approximately 12,000 new cases each year or 40 cases/million population.2 Since 2005, the most common etiologies of SCI are motor vehicle crashes (41.3%), falls (27.3%), violence (15%), and sports (7.9%). Incomplete tetraplegia occurs in approximately 38.3% of traumatic spinal injuries, followed by complete paraplegia (22.9%), incomplete paraplegia (21.5%), and complete tetraplegia (16.9%). Complete tetraplegia is a devastating injury, with a less than 1% rate of complete neurologic recovery by hospital discharge.2 Over the last 15 years, the percentage of persons with incomplete tetraplegia has increased while complete paraplegia and complete tetraplegia have decreased slightly. Intoxication is a factor in many traumatic injuries.
Respiratory complications are the leading cause of death during the first year after SCI, and the third leading cause of death thereafter.3 For patients with injury at age 20, surviving 24 hours and ventilator dependent from any level of injury, the life expectancy is only 18.1 years and at 1 year postinjury rises to 24.9 years.2 The life expectancy for older (aged 60) ventilator-dependent patients who survive the first 24 hours after SCI is 1.8 years and at 1 year after injury is only 3.6 years.2 In the past, renal failure was the leading cause of death after SCI, but due to advances in urological management, pneumonia, sepsis, and pulmonary emboli currently appear to have the greatest impact on reduced life expectancy.2
The median days spent in the acute care unit for those who immediately enter a “Model Spinal Cord Injury Unit” has declined from 24 days between 1973 and 1979 to 12 days since 2005. Shorter stays in rehabilitation, from 98 to 38 days, are also noted.2 The increasing life expectancy of spinal cord injured patients has led to an increased worldwide prevalence of SCI, now approaching 2 million.4
Intensivists working in nondesignated trauma center hospitals should consider the transfer of an acute spine injured patient to a level I trauma center as soon as possible.5 There is level II evidence that trauma centers and specialized neurocritical care have a positive impact on mortality and disability after spinal cord injury.
CLASSIFICATION OF VERTEBRAL INJURIES
The cervical spine is divided into the upper cervical spine (C1 [atlas]-C2 [odontoid or dens]) and lower (subaxial) cervical spine (C3-C7). The cervical vertebrae are smaller and very mobile. Common C2 fracture includes that of the dens and bipedicular (or hangman’s fractures). The latter are frequently caused by acute neck hyperextension. After trauma to the cervical spine, fractures may appear on x-ray, but the stability of the spine depends on the ligaments which are not visible on plain x-rays or computed tomography (CT) scans. The thoracolumbar spine (T1-12; L1-5) vertebrae are larger and less mobile.
The Denis three-column theory of spinal stability6 divides the vertebral column into anterior, middle, and posterior columns. Together, these columns form functional units that contribute to spinal stability and can explain the effect of various injuries on spinal destabilization. The anterior column contains the anterior longitudinal ligament, the anterior half of the vertebral body, intervertebral disk, and its annulus fibrous. The middle column contains the posterior longitudinal ligament, the posterior half of the vertebral body, intervertebral disk, and its annulus. The posterior column contains the bony posterior neural arch, the intervertebral articulations, the ligamentum flavum, and the interspinous and supraspinous ligaments.
The spine can be damaged by blunt or penetrating trauma and the forces of flexion, distraction (extension), and rotation. Fractures of the spine can be classified based on the pattern of injury and the forces. The types of fractures include compression (wedge) fractures, caused by flexion and compression in the anterior column with variable involvement of the middle and posterior column,1 burst (or crush) fractures involving the anterior and middle columns characterized by loss of height of the vertebral body, caused by axial compression forces associated with high energy trauma (eg, MVA, falls from a heights, and sports-related trauma), most commonly found at the thoracolumbar junction between levels T10 and L2; seatbelt or Chance thoracolumbar spine fractures are the result of flexion and distraction forces and involve middle and posterior columns with injury to ligamentous components, bony components, or both; and dislocations that involve all three columns (Figs. 119-2 to 119-4). Chance fractures are often associated with intra-abdominal injuries. Lateral flexion and rotation (with or without anterior -posteriorly directed force) result in rotational fracture-dislocations. The posterior and middle columns are damaged with varying degrees of anterior column insult. The rotational forces disrupt the posterior ligaments and facet joints. With sufficient rotational force, the upper vertebral body rotates and carries the superior portion of the lower vertebral body along with it causing a radiographic “slice” appearance sometimes seen with these types of injuries.1
FIGURE 119-2
Sagittal reconstructed CT image: three-column thoracic spine injury in a 32 year-old female with poly substance abuse who jumped from a second story window. This patient has wedge and superior endplate fractures with bony avulsions of T7, T8, and T9. There are also mild compression deformities at T10 and T12. Avulsion spinous process injuries are seen at T6 and T8. At the top of the image is a stable C7 spinous process fracture. There was no spinal canal compromise and the patient was neurologically intact. This patient also had rib head fractures at T8 and T9, rendering this injury highly unstable, requiring surgical stabilization.
FIGURE 119-3
Intraoperative fluoroscopy images in lateral (A) and AP (B) projections showing the construct of pedicle screw fixation for the thoracic spine injury in Figure 119-2. Given destruction of the T8 vertebral body and pedicles, no screws were able to be placed at that level. This patient had significant pain preoperatively and was remanded to bed rest with log-roll precautions. Her pain quickly resolved after stabilization.
FIGURE 119-4
A, B. Sagittal and axial CT imaging of an L3 burst fracture in patient who fell off a ladder while intoxicated. These burst fractures are caused by direct axial force, such as landing on both feet. Transitional junctions, such as the cervicothoracic and thoracolumbar regions are particularly vulnerable to this axial loading force. In this fracture there is approximately 85% spinal canal compromise due to retropulsed bone fragments, especially on the right side. Burst fractures at the cauda equina level are more forgiving in terms of neurological function and this patient was neurologically intact. Patients presenting with neurological deficits or bladder dysfunction and diminished rectal tone are candidates for early decompression. Treatment for thoracolumbar burst fractures (operative vs nonoperative) remains controversial in neurologically intact patients. This patient underwent surgical decompression and stabilization.
Other types of stable fractures include spinous process and transverse process fractures, osteophyte fractures, avulsion fractures, and injury to trabecular bone (Fig. 119-2).
The grading of the stability of vertebral fractures is based on biomechanical stability, fracture morphology, osteoligamentous integrity, spinal canal or neural foramina deformity, and neurological impairment. Disruption of two or more columns results in a potentially unstable spine, therefore burst fractures, Chance fractures, and dislocations are potentially unstable but compression fractures are stable. For example, compression fractures do not lead to neurological lesions and are stable; burst fractures are potentially unstable but in the presence of neurological signs related to migration of the vertebral body, they are unstable (Fig. 119-4A, B). The stability of seat belt fractures depends on the displacement of the posterior arch, but they usually do not cause neurological lesions. Dislocations are always unstable as they are fracture-dislocations and almost always associated with neurological lesions.
The spinal canal may be narrowed from intrusion of vertebral body fragments. Displacement of bone fragments into the spinal canal may cause compression of the spinal cord or nerve roots, as well as vascular injury.1 Posterior element displacement, vertebral body or facet dislocation, or subluxation are found in unstable fractures and are more likely to involve spinal cord compression.
PRIMARY VERSUS SECONDARY INJURY
Neurological injury is conceptualized into primary and secondary injury. Since neurons cannot regenerate, this concept has important implications for preventive management and treatment. Primary injury occurs at the time of the initial traumatic insult (eg, spinal cord compression, laceration, transection, intramedullary and extramedullary hematoma formation, foreign bodies) and results in neurons that are dead or irreversibly damaged (ie, necrosis, apoptosis), injured (eg, potentially reversible ischemia), or intact (uninjured). In SCI, the compressive force is typically caused by bone or disc material that enters the spinal canal as a consequence of vertebral fracture or dislocation. Although primary preventive efforts aim to reduce the incidence of primary injuries, little can be done from a therapeutic standpoint to repair this component of the injury once it has occurred.4 Secondary injury is any insult that occurs subsequent to the primary injury (eg, continued compression, expansion of hematomas, unstable spine or fragment movement, decreased spinal cord perfusion due to hemodynamic instability, cardiac arrest, respiratory arrest, and molecular events triggered by ischemia and inflammatory pathways) and results in additional neuronal damage to previously uninjured neurons as well as the particularly susceptible primarily injured neurons.
Cellular processes involved in secondary injury include proinflammatory cytokine release, free radical formation, release of excitotoxic amino acids (eg, glutamate), ischemia-reperfusion injury, activation of macrophages, vasospasm, and cytotoxic edema.4,7-9 Biomarkers for the early detection of spinal cord ischemia, including rapidly induced heat shock proteins, are under investigation.10 The damage from SCI can spread superiorly and inferiorly from the initial site. Research on the immunological consequences of SCI suggests that cytokines (eg, tumor necrosis factor alpha [TNFa]) and other immune mediators, such as nitric oxide (NO) may have the capability to induce complete, but reversible, conduction failure.11 To the extent that associated traumatic injuries and medical complications such as sepsis influence endogenous mediator production, they may contribute to reversible neurologic deficits, either early onset or later. Therefore, the primary injury sets limits (that are not always initially obvious) on the potential extent of recovery and the degree of secondary injury determines the extent of the potential recovery actually achieved. Secondary injury if limited or prevented can potentially lead to reduced spinal cord damage and improved neurological outcomes. The prevention of secondary injury is main focus of surgical and critical care management as well as in the development of potential therapeutic agents.
GENERAL MANAGEMENT ISSUES AFTER ACUTE SCI
Patients with acute SCI are managed in a multidisciplinary fashion from the accident scene by emergency response personnel, to triage and stabilization in the emergency department by trauma or general surgeons and neurosurgeons or orthopedic spine surgeons, followed by transport to radiology for diagnostic imaging or interventional procedures, operating room, or ICU, the order of which is determined by the nature of the acute injuries.
The initial trauma assessment (ATLS—acute trauma life support) protocol for life-threatening injuries is followed utilizing the “A-B-C-D-E” approach consisting of Airway maintenance, with immobilization of the spine with a rigid cervical collar and strapped to a backboard to secure the entire spine; Breathing and ventilation; Circulation with control of bleeding; Disability: rapid neurologic evaluation; and Exposure: removal from harmful environment and protection from hypothermia. At all times during the acute management, the principles of primary survey (immediate management of acute life-threatening problems), secondary survey (identification of remaining major injuries and setting management priorities in hemodynamically stable patients), and tertiary survey (identify occult injuries) are followed.
Upon admission to the ICU, a tertiary head-to-toe examination is performed to completely identify potential multiple injuries.12 The key neurological exam points include level of consciousness, cranial nerve function, movement in the extremities, and sensation in determining a spinal cord level of injury.
Basic ICU monitoring consists of continuous ECG, pulse oximeter, and blood pressure via arterial line, with additional hemodynamic monitoring if needed. Central venous access, nasogastric tube insertion, and Foley catheter placement are recommended if there are no contraindications. Care must be taken to insure spine immobilization until definitive treatment. When repositioning or preparing for transport, the patient should be log-rolled as a unit. SCI patients are more prone to develop skin breakdown and decubitus ulcers and transfer from the backboard onto a firm cushioned surface should be accomplished as early as possible. When prolonged immobilization is anticipated, a specialized immobilization bed should be ordered.
A review of the diagnostic imaging, laboratory results, and surgical procedures performed thus far, and communication with the surgeon (neuro or orthopedic) regarding anticipated diagnostic imaging, surgical interventions, and ICU monitoring and management is critical. Ordering laboratory studies, either as initial or follow-up, including arterial blood gases, electrolytes, complete blood count, coagulation profile, type and cross-match packed red blood cells, lactate, and liver function tests is indicated. If appropriate and omitted thus far, a toxicology screen should be ordered. Any loss of consciousness may be a sign of traumatic brain injury (TBI) that may not have been evident on initial head CT. Healthcare proxy or available family or friends should be asked to provide pre-accident and accident history as well as advanced directives.
Beyond the initial mortality risks secondary to comorbid multitrauma including head injury, acute SCI patients, particularly those with cervical level and severe SCI, are at risk for respiratory arrest, hypoxemia, and cardiovascular instability. Failures of the cardiovascular system and respiratory system are more strongly correlated with the severity of injury (ASIA motor score) than the anatomic level of injury.13 These complications may manifest on presentation or be transient and episodic, and usually occur within the first 7 to 14 days after the initial SCI.14
DIAGNOSTIC IMAGING
After initial resuscitation and cardiopulmonary stabilization, the goal is rapid and accurate assessment of the spinal column to guide potential surgical decompression and stabilization.1
Plain x-ray films have been replaced with multidetector computed tomography (MDCT) and magnetic resonance imaging (MRI) in high risk patients with blunt cervical spine injury as well as thoracic and lumbar spine injury. In the cervical spine for example, plain x-ray films detect only 60% to 80% of fractures; a significant number of fractures are not visible, even when three views of the spine are obtained.1 In the cervical spine, MDCT detects 97% to 100% of fractures, provides finer anatomical delineation of the bony spinal canal, and also depicts epidural hemorrhages, significant soft tissue abnormalities such as traumatic disk herniation (Fig. 119-5) or hematomas, and abnormalities distant to the spine such as in the chest, abdomen, and head. MDCT is also more accurate than plain x-rays in the diagnosis of cervicothoracic junction and thoracolumbar spine fractures, and shortens the screening time to removal of spine precautions, creating the opportunity for earlier mobilization.
In spine fractures, the spinal canal is often narrowed from translation and intrusion of vertebral body fragments. In addition to axial images, multiplanar formatting of volumetric CT data allows image orientation into sagittal and coronal image planes helpful for demonstrating abnormalities in alignment, clarifying the nature of fractures, and measuring the anterior–posterior spinal canal diameter.1
The main limitation of MDCT is the inability to rule out SCI or adequately assess the soft-tissue structures or ligaments critical for maintaining spinal stability such as the disco-ligamentous complex in the cervical spine and the posterior ligamentous complex in the thoracolumbar spine. MRI is the favored technique for the detection of soft tissue injuries15 and SCI and plays an important role in the assessment of acute SCI patients.1 In crush fractures and other unstable injuries, MDCT is useful for assessing bone fragments, whereas MR imaging is superior for demonstrating SCI and paraspinal hematomas. Any patient with presumed SCI should undergo an MR imaging examination as soon as possible. In patients with SCI, MR imaging is able to reveal the location, severity, and cause of spinal cord compression (Fig. 119-6A, B).1 This is especially useful in the management of patients with incomplete SCI, for whom surgical intervention may prevent further deterioration.
FIGURE 119-6
A. Sagittal CT image of a patient who fell down a manhole. He presented with dense quadraparesis consistent with central cord syndrome. Scan shows signs of ankylosing spondylitis. He has ossification of the posterior longitudinal ligament, most severe at C3-C4 (see thick arrow) which was considered the possible culprit for the spinal injury. The CT also reveals a fracture of the C6 vertebral body (see thin arrow). B. Sagittal T2-weighted MRI image revealed a spinal cord contusion (see arrow) at C6 with signal changes within the disc space indicating disruption but no herniation. While there was, indeed, significant cord compression at C3-C4, the C6 injury was, in fact, responsible for the spinal cord injury. The patient underwent early posterior decompression and stabilization.
MR imaging is the modality of choice for assessing extrinsic compression of the spinal cord by bone fragments or a traumatic disk herniation, lesions involving the intervertebral disks and spinal ligaments, and to identify spinal cord lesions such as spinal cord contusion/edema, intramedullary hemorrhage, and complete transsection of the cord.1,16 The exam protocol for detecting spinal ligamentous injury includes sagittal T1, sagittal gradient recalled T2, and sagittal STIR (short TI inversion recovery—suppresses signal from fat) images, and axial imaging. Edema in the interspinous or supraspinous ligaments is prominently demonstrated on STIR images (Fig. 119-7A, B). In cervical spine trauma, MR imaging is highly sensitive for injury to intervertebral disk (93%), posterior longitudinal ligament (93%), and interspinous soft tissues (100%), but less sensitive in detecting anterior longitudinal (71%) and flavum (67%) ligament injury.17 MRI can also demonstrate bone contusions within vertebrae adjacent to the site of trauma (Fig. 119-8).
FIGURE 119-8
Sagittal MRI T2-weighted images of the lumbar spine. This patient suffered an L1 burst fracture. There is increased signal intensity within the L1 body indicating contusion/edema. In addition, higher signal intensities (see arrow) can also be found in the L2, L3, and L4 vertebral bodies indicating injury to those vertebrae that may or may not manifest on bone CT imaging.
Sagittal T2-weighted MRI is most effective at evaluating spinal cord compression and the combination of sagittal CT and T1-weighted MRI are most effective at evaluating spinal canal compromise by comparing the AP canal and AP cord diameters at the level of maximal injury versus at the nearest adjacent normal levels.4 The presence of >25% canal compromise on sagittal CT predicted the MRI presence of cord compression in 100% of cases; however, in those with <25% canal compromise on CT, the majority continued to exhibit MRI evidence of cord compression illustrating the poor specificity of CT in ruling out spinal cord compression.4 CT angiography of the head and neck is used to evaluate for carotid or vertebral dissection18 in patients with cervical spine fracture and neurologic deficits suspicious for stroke.
MRI is helpful prognostically. Patients with intramedullary hemorrhage or cord transsection are unlikely to regain any significant neurological function, whereas patients with cord edema or contusion may significantly recover neurological function.19 MRI may provide prognostic information about recovery from spinal cord injury without radiological abnormality (SCIWORA) defined as neurological symptoms of SCI without abnormalities on x-ray or MDCT.4,20
ICU MANAGEMENT
Patients with acute SCI, particularly those with severe cervical level injuries or those with any respiratory or hemodynamic instability, should be monitored and managed in the ICU.14
AIRWAY, BREATHING, AND RESPIRATORY ISSUES
After acute spine injury, neuromuscular dysfunction, altered mental status, inability to handle secretions which may be copious, bronchoconstriction, and acute lung injury (noncardiogenic pulmonary edema) are common. Retained secretions can occur insidiously resulting in acute deterioration and lead to the need for aggressive pulmonary toilet with frequent repeated suctioning and bronchoscopy that should be performed with low a threshold.
Due to the greater effects on respiratory function, more rostral and complete cervical SCI patients usually acutely require intubation and mechanical ventilation. Regardless of the level of injury, early intubation under controlled circumstances is preferred to avoid secondary injuries resulting from hypoxemia or respiratory system-induced hemodynamic failure. Evidence of respiratory failure includes altered mental status, hypoxemia, rapid, shallow, or irregular breathing with associated respiratory alkalosis (early) or elevated [Math Processing Error] (late) or a progressive decline in serial vital capacity (VC), or VC <1.0 L.
In critically ill patients in general, and trauma and SCI patients in particular, tracheal intubation is significantly more difficult due to factors such as the need for precautionary neck stabilization, bleeding, vomiting, oropharyngeal secretions, respiratory dysfunction, airway edema, hemodynamic instability, and encephalopathy. Halo traction devices are not readily removable and also limit the ability to position the airway for intubation, increasing difficulties. Bradycardia and hypotension during endotracheal intubation are more common in the tetraplegic patient. Complications such as hypoxemia, aspiration, bradycardia, and cardiac arrest increase significantly21 as the number of laryngoscopic intubation attempts increases. A first year anesthesia resident performing direct laryngoscopy in the relatively controlled setting of the operating room requires about 47 tracheal intubations to achieve a 90% probability of a success.22 In addition to direct laryngoscopy devices, it is important to have additional devices prepared and ready to aid with intubation such as intubation bronchoscopes and video laryngoscopic devices. Indirect optical laryngoscopy does not require aligning the head and neck axis and provides better visualization of the vocal cords facilitating faster, less traumatic intubation that requires less sedation. It appears that the success rate, even in previously untrained operators, with optical laryngoscopy is much higher than with traditional direct laryngoscopy using the Macintosh blade.23 In patients with spinal immobilization, video laryngoscopy appears to improve the success rate, especially in less experienced operators.24
The stabilization of neck injuries often involves external fixation with cervical collars, vests and halo traction devices as well as surgical stabilization. Cervical collars may be removed temporarily while maintaining in-line positioning during tracheal intubation or to place central lines.
Two large series have demonstrated the safety of orotracheal intubation with manual in-line stabilization of the neck in the setting of acute cervical injury. If possible, 8.0 or 9.0 mm internal diameter sized endotracheal tubes should be placed on initial intubation in anticipation of the need for pulmonary toilet and bronchoscopy with larger bore suction channels. Aggressive suctioning, chest physiotherapy, pulmonary toilet including flexible bronchoscopy at signs of collapse, thick and copious secretions, or hypoxemia is helpful in treating and limiting the extent of atelectasis.
Rapid-sequence intubation is preferred in the setting of acute head or spine trauma; however, succinylcholine, a paralytic agent used due to its rapid onset of action and short half-life, may precipitate hyperkalemia in the setting of subacute SCI, that is, 4 to 5 days after acute SCI.25
The development of respiratory failure and the length of time of ventilator dependence after spinal cord injury, apart from additional traumatic injuries, depend on the degree and level of SCI. More complete and rostral levels of SCI lead to more significant reductions in pulmonary function with a greater incidence of prolonged respiratory failure, separately from additional traumatic injuries.
Arterial hypoxemia is common in the acute stage after SCI, even in patients with adequate ventilatory ability and normocarbia.26 In the first week following cervical cord injury, individuals with an FVC of less than 25% of predicted are likely to develop respiratory failure requiring ventilator support.27 In patients not requiring immediate intubation, close attention to signs of respiratory impairment supplemented by arterial blood gas and pulmonary function (vital capacity or FEV1) trends is recommended at regular intervals until the patient is stable.
The effects of acute SCI, particularly cervical injury, on the respiratory system are related to reduced inspiratory and expiratory respiratory muscle strength leading to hypercarbic respiratory failure, noncardiogenic pulmonary edema (acute respiratory distress syndrome [ARDS]) leading to hypoxemic respiratory failure, and decreased sympathetic tone (with increased relative parasympathetic tone) leading to bronchoconstriction and increased airway secretions. Sympathetic (bronchodilatory) innervation of the lungs arises from the T1-T6 level so that tetraplegic (ie, cervical) SCI leads to loss of sympathetic innervation to the lungs and unopposed or increased parasympathetic vagal activity that can result in decreased baseline airway caliber.27 The use of anticholinergic bronchodilators such as ipratropium may be considered28; however, it is not known if the baseline bronchoconstriction in tetraplegia contributes to respiratory symptoms.27 Aggressive suctioning of secretions can result in bronchial stimulation and increased parasympathetic output resulting in bradyarrhythmia or conduction blocks.
Cervical and upper thoracic cord injury disrupts neuronal output to the diaphragm, intercostal muscles, accessory respiratory muscles, and abdominal muscles (Fig. 119-9) causing reduction in spirometric and lung volume parameters.27 The phrenic nerves innervating the diaphragm exit the spinal cord from levels C3-C5. Spinal cord injury above C3 leads to apnea, the need for immediate ventilatory support, and permanent ventilator dependence as well as tetraplegia. Damage at the C4 level and below allows some recovery of respiratory function. With acute injury at the C5 level and below, diaphragmatic function is preserved, but paralysis of the intercostal and abdominal wall muscles supplied by the thoracic segments leads to paradoxical chest wall contraction with abdominal expansion as the diaphragm contracts and descends.

Full access? Get Clinical Tree
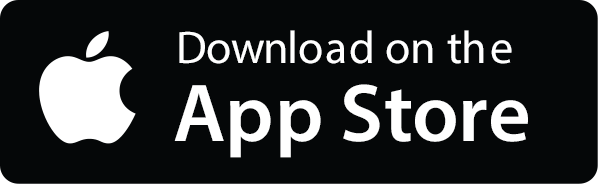
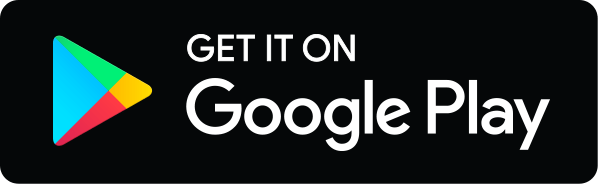