KEY POINTS
Scoliotic curves greater than 100° may cause dyspnea; curves greater than 120° are associated with alveolar hypoventilation and cor pulmonale.
Biphasic positive airway pressure may be effective in patients with acute hypercapnic respiratory failure.
Low tidal volumes and high respiratory rates likely minimize the risk of barotrauma during mechanical ventilation; however, gradual institution of anti-atelectasis measures may improve gas exchange and static compliance.
Nocturnal hypoxemia is common and may contribute to cardiovascular deterioration; routine polysomnography is recommended.
Strategies for management of patients with chronic ventilatory failure include daytime intermittent positive pressure ventilation, nocturnal noninvasive ventilation, and ventilation through tracheostomy.
Acute deterioration in respiratory status can occur from disease progression, upper and lower respiratory tract infections, congestive heart failure, failure to clear secretions, atelectasis, aspiration, and pulmonary embolism.
Most patients with chest wall deformity survive their first episode of acute respiratory failure.
Patients with idiopathic pulmonary fibrosis admitted to the ICU with acute respiratory failure have an extremely poor prognosis.
If mechanical ventilation is deemed appropriate, the use of low tidal volumes and high respiratory rates during mechanical ventilation likely minimize ventilator-induced lung injury.
Idiopathic pulmonary fibrosis is typically refractory to pharmacotherapy.
Lung transplantation is a viable option in selected patients with end-stage fibrosis.
Thoracic cage deformity and pulmonary fibrosis both result in a restrictive limitation to breathing. Although relatively rare in the context of pulmonary intensive care, these disorders present unique challenges that complicate ICU management. In this chapter, we describe the pathophysiologic derangements in cardiopulmonary function associated with these disorders and how they affect management during acute illness. A primary goal of this chapter is to offer a strategy for cardiovascular management and mechanical ventilation that minimizes the risk of ventilator-induced complications and maximizes the chance for early, successful extubation. Many of these recommendations are grounded more on general precepts than on disease/disorder-specific evidence.
PATIENTS WITH THORACIC CAGE DEFORMITY
Although a number of disorders can deform and restrict the movement of the respiratory system (Table 58-1), kyphoscoliosis (KS) is the prototypical cause of severe thoracic deformity. Kyphoscoliosis is the combination of kyphosis (posterior deformity of the spine) and scoliosis (lateral deformity of the spine). It is far more common than isolated cases of kyphosis or scoliosis, placing over 200,000 people in the United States at risk of developing respiratory failure.1 Most cases are idiopathic and begin in childhood.2 Other cases result from congenital defects, connective tissue disease, poliomyelitis, thoracoplasty, syringomyelia, vertebral and spinal cord tumors, and tuberculosis.
The pathophysiologic consequences of KS correlate with the degree of spinal curvature, but there is considerable variability.1-3 Patients with severe deformity can lead long and relatively symptom-free lives,4-6 while patients with lesser degrees of curvature may develop ventilatory failure and cor pulmonale at a young age.7 The reason for this variability is not always clear. However, sleep-disordered breathing underlies clinical deterioration in some patients.8,9
The combination of a moderate kyphotic deformity and a moderate scoliotic deformity is functionally equivalent to a severe deformity of either alone.3 Of the two, however, scoliosis produces greater physiologic derangements. In KS, scoliotic curves less than 70° (Fig. 58-1) rarely cause problems, while angles greater than 70° increase the risk of respiratory failure.1,2 The earlier in life this angle is achieved, the greater the risk of eventually developing respiratory failure, as curvature angles increase by an average of 15° over 20 years from an initial angle of 70°.10-12 Angles greater than 100° can cause dyspnea; angles ≥120° can result in alveolar hypoventilation and cor pulmonale.1,7
FIGURE 58-1
Determination of the scoliotic angle by the Cobb method. The scoliotic deformity consists of a primary initiating curve and a secondary compensatory curve. The scoliotic angle is commonly determined by the intersection of lines estimating the position of the upper and lower components of the primary curve. (Reproduced with permission from Grippi MA, Fishman AP. Respiratory failure in structural and neuromuscular disorders involving the chest bellows. In: Fishman AP, ed. Pulmonary Diseases and Disorders. 2nd ed. New York, NY: McGraw-Hill; 1988.)
In order to decrease respiratory work, patients with severe deformity and low respiratory system compliance take rapid and shallow breaths. Thoracic deformity with loss of height and asymmetric chest wall excursions often dominates the physical exam findings. Chest auscultation may reveal crackles or coarse wheezes from atelectasis and failure to clear secretions. Cardiac examination may demonstrate a loud P2, right ventricular heave, or jugular venous pressure elevation, indicating the presence of pulmonary hypertension.3
Kyphoscoliosis reduces total lung capacity (TLC) and functional residual capacity (FRC) (Fig. 58-2). Residual volume (RV) may be normal or decreased to a lesser extent than FRC. Vital capacity (VC), inspiratory capacity (IC = TLC − FRC), and expiratory reserve volume (ERV = FRC − RV) are all decreased.13 Interestingly, pulmonary function in adolescents is only weakly related to the angle of scoliosis.6 In these patients, VC is also influenced by the degree of thoracic kyphosis, location of the curve, and number of vertebral bodies involved.14 Furthermore, spinal column rotation, respiratory muscle strength, and duration of the curve are not clearly related to pulmonary function in these patients. It does appear that age-related decreases in chest wall compliance increase the risk of developing ventilatory failure.12,15
FIGURE 58-2
Schematic drawing of the abnormalities of lung volumes in common restrictive diseases. By contrast with normal subdivisions (left trace) of plethysmographic gas volumes (TLC, FRC, and RV) and spirometric volumes (IC, VC, and ERV), kyphoscoliosis and pulmonary fibrosis reduce VC and TLC by restricting IC, with lesser reductions in FRC (traces 2 and 3). Ankylosing spondylitis (like neuromuscular diseases of the chest wall) limits IC and ERV excursions around a normal FRC, so TLC is reduced and RV is increased, causing a large decrease in VC (trace 4). Obesity greatly reduces FRC to eliminate ERV without much change in TLC or RV, so VC is normal and IC is increased (trace 5, far right).
Patients with fibrothorax or thoracoplasty have similar abnormalities.1,16-18 By contrast, obesity mainly reduces FRC and ERV and lesser changes in RV, VC, or TLC.19-21 In patients with ankylosing spondylitis, ERV and IC excursions are restricted around a normal FRC, such that RV increases and TLC decreases to reduce VC, a pattern similar to that seen in neuromuscular diseases of the chest wall.22-27
In each of these disorders, it is the chest wall that limits the excursion of the respiratory system; the lungs and respiratory muscles are affected secondarily and to a lesser degree. In health, TLC is largely determined by the pressure-volume (P-V) curve of the lung, but in KS the P-V curve of the noncompliant chest wall dominates, lowering TLC and FRC while RV is relatively spared (Fig. 58-3). Note that the P-V curve of the respiratory system is shifted downward and to the right, requiring patients to work harder for each tidal breath. Normal lung compliance and respiratory muscle strength are assumed in Figure 58-3, although reductions in both contribute to low lung volumes in selected patients with either parenchymal lung disease or neuromuscular dysfunction. Indeed, in four patients with severe KS requiring mechanical ventilation for acute respiratory failure, both lung and chest wall compliance were decreased.28 Decreased lung compliance may occur as a result of infection, edema, atelectasis, or abnormalities in alveolar surface tension and may respond to positive-pressure ventilation (see below).29
FIGURE 58-3
Pressure-volume curves of the chest wall, lung, and respiratory system in scoliosis. The P-V curve is shifted downward and to the right, requiring patients to generate large transpulmonary pressures for small amounts of air. CW, chest wall; NL, normal lung; RS, respiratory system. (Reproduced with permission from Bergofsky EH. Respiratory failure in disorders of the thoracic cage. Am Rev Respir Dis. April 1979;119(4):643-669.)
Inspiratory muscle dysfunction occurs when the deformed thorax places inspiratory muscles at a mechanical disadvantage or there is respiratory muscle fatigue.30,31 When KS is a manifestation of neuromuscular disease (eg, postpolio syndrome), inspiratory muscles may be affected directly by the neuromuscular disease.
Significant daytime hypoxemia rarely occurs until the development of daytime hypercapnia.1 However, nocturnal hypercapnia with hypoxemia occurs early, particularly during rapid eye movement (REM) sleep, and may underlie cardiovascular deterioration in some patients.8,9
The alveolar-arterial gradient [(a-a)DO2] on room air is usually ≤25 mm Hg, even in late stages of KS.1 This modest increase in (a-a)DO2 results primarily from ventilation-perfusion (˙V/˙Q) inequality caused by atelectasis or underventilation of one hemithorax.32˙V/˙Q inequality further contributes to a low diffusing capacity as does failure of the vascular bed to grow normally in a distorted chest.
Alveolar hypoventilation results in part from an increase in the dead space to tidal volume ratio (Vds/VT). Vds/VT is increased because VT is reduced in hypercapnic patients; anatomic and alveolar dead space are usually normal.1 Minute ventilation is often normal but maintained by higher respiratory rates. The use of small VT minimizes work of breathing and is a sign of inspiratory muscle dysfunction.30,33 As inspiratory muscle strength falls, the partial pressure of arterial carbon dioxide (PaCO2) rises and further impairs diaphragm function.29,34
Ventilatory response to high concentrations of inspired CO2 is normal in normocapnic patients with KS. However, in hypercapnic patients the response is blunted by buffering from elevated cerebrospinal fluid bicarbonate or by a derangement in the central drive to breathe.33
A further consequence of severe KS is pulmonary hypertension and cor pulmonale.7 Left untreated, patients with cor pulmonale typically die within 1 year.3 Initially, pulmonary hypertension occurs only with exercise, but over time it occurs at rest as well. Pulmonary hypertension is usually caused by increased pulmonary vascular resistance (PVR) and not left atrial hypertension.1 Thus, there is an increased gradient between the pulmonary artery diastolic pressure and the pulmonary capillary wedge pressure. Identifying and treating reversible conditions such as pulmonary embolism, hypoxemia, and sleep-disordered breathing lowers pulmonary artery pressure and delays the onset of right ventricular failure.36-39 However, there also may be irreversible changes associated with proliferation of the media in smaller, pre-capillary pulmonary vessels.3,32 The mechanism by which this occurs is not known, but blood flow through vessels narrowed by low lung volumes, blood flow through fewer vessels, and the vascular effects of chronic alveolar hypoxia and hypercapnia are likely important. Kinking of larger vessels as they travel through deformed lung may further increase pulmonary artery pressures in some cases.1 Prostacyclin analogues, endothelin receptor antagonists, and phosphodiesterase inhibitors have not been rigorously studied for use in pulmonary hypertension associated with chest wall deformities and should be used at the discretion of the clinician.
ACUTE CARDIOPULMONARY FAILURE
In a paper published nearly 30 years ago, clinical features of 20 patients in acute respiratory failure (ARF) for the first time were reported. Mean deformity for the group was 113° and admission blood gases showed severe arterial hypoxemia (partial pressure of arterial oxygen [PaO2] of 35 ± 7 mm Hg), acute-on-chronic hypercapnia (PaCO2 of 63 ± 9 mm Hg), and mild arterial acidemia (pH of 7.34 ± 0.08).40 Cor pulmonale was present in 60% of patients. Seven (35%) patients required intubation and mechanical ventilation, while the remaining patients were managed successfully without mechanical ventilation in an age when noninvasive positive-pressure ventilation (NIV) was not routinely available.36 There were no statistical differences in admission blood gases, cause of respiratory failure, age, or degree of spinal curvature between patients who required mechanical ventilation and those who did not. Outcome was surprisingly good. All patients survived their initial episode of ARF and subsequently experienced 2.4 episodes of ARF each during the follow-up period (median of 6 years). Median survival after the first episode of ARF was 9 years. On discharge, mean PaO2 was 63 mm Hg and mean PaCO2 was 55 mm Hg. This study performed in the era before noninvasive positive pressure ventilation demonstrates the utility of aggressive management of ARF, even in cases of severe deformity. More recent data are not available.
The etiology of ARF may be obvious, but it is also important to note that the trigger may be minor and even obscure in patients with minimal respiratory muscle reserve. ARF is most commonly precipitated by pneumonia, upper respiratory tract infection, or congestive heart failure.40 Aspiration should be considered in the differential diagnosis of ARF because chest wall deformity may affect the swallowing mechanism. Risk factors for clotting (pulmonary hypertension and decreased mobility) mandate consideration of pulmonary embolism. Finally, identifying and treating airflow obstruction when possible can help restore the delicate balance between strength and respiratory system load. Limited data suggest that airway resistance is increased in mechanically ventilated patients with KS (∼20 cm H2O/L/s) and refractory to bronchodilators.28 This may stem from torsion or narrowing of the central airways, which may be determined by bronchoscopic examination.41
A primary goal is to correct hypoxemia by increasing the fraction of inspired oxygen (FiO2) until an oxyhemoglobin saturation of 90% to 92% is achieved.35 Adequate saturation by pulse oximetry should be confirmed by arterial blood gas analysis, which also helps establish the acid-base status. If adequate oxygenation cannot be achieved with face-mask oxygen, NIV should be initiated unless there are indications for intubation (see below).42,43
Hypoxemia causes pulmonary vasoconstriction and may precipitate right ventricular failure in patients with preexisting right heart disease. Its causes include alveolar hypoventilation, ˙V/˙Q inequality, and intrapulmonary shunt. Right-to-left intracardiac shunts have also been reported in the setting of thoracic deformity.44 Low mixed venous PaO2 (PˉvO2), a frequent finding in patients with pulmonary hypertension and low cardiac output, further lowers arterial oxygenation in the setting of ˙V/˙Q inequality.
Evaluation of shock in patients with KS is similar to that described elsewhere in this text (see Chaps. 31 and 33). Hypotensive patients not responding to an initial volume challenge should be considered for central venous catheter placement (and rarely right heart catheterization), measurement of the central (or mixed) venous oxyhemoglobin saturation, lactate, and/or bedside echocardiography to further direct therapy. Mechanical ventilation is indicated for nearly all patients with persistent shock, in part to redirect blood flow from the muscles of respiration, which can account for as much as 25% of the cardiac output.45,46 Mechanical ventilation and sedation decrease oxygen consumption (and thus supplemental oxygen requirement) and lactate generation.
When sepsis causes shock, patients with chest wall deformity and pulmonary hypertension may not mount a hyperdynamic response. When right ventricular failure causes shock, a vicious cycle ensues. As the right ventricle fails, cardiac output and systemic blood pressure fall, limiting perfusion to the right ventricle from the aortic root. Right ventricular end-diastolic volume increases and shifts the interventricular septum to the left, decreasing left ventricular compliance and further reducing cardiac output and systemic blood pressure. Ensuring an adequate circulating volume and correcting hypoxemia to reduce pulmonary vasoconstriction are the first goals of therapy. Increasing systemic blood pressure with norepinephrine may increase perfusion pressure to the right ventricle.47
We consider lower extremity Doppler exams to evaluate for venous thromboembolism; however, a single lower extremity Doppler exam is insufficiently sensitive to rule out venous thromboembolism as a cause of clinical decompensation. Serially negative Doppler exams provide an added sense of security as does a negative D-dimer. Computed tomographic (CT) pulmonary angiography is preferable to ventilation-perfusion imaging in chest wall deformity, and may provide additional clues regarding the etiology of ARF. In the absence of venous thromboembolism, preventive therapy with prophylactic doses of unfractionated heparin is indicated. The use of low molecular weight heparin as a prophylactic intervention does not appear to be superior to unfractionated heparin.48
Decreased pulmonary compliance lowers lung volume, which in turn limits cough efficiency and mucus clearance.49 To improve compliance and treat atelectasis, short periods (15-20 minutes) of intermittent positive-pressure ventilation (IPPV) delivered by mouthpiece 4 to 6 times daily using inflation pressures between 20 and 30 cm H2O have been recommended.29 IPPV increases lung compliance by 70% for up to 3 hours in acutely ill patients, suggesting that IPPV lowers surface tension by altering the surfactant lining layer. Mechanical insufflator-exsufflator devices used with pressures of negative 20 to 30 cm H2O and positive 20 to 30 cm H2O may also be beneficial to clear secretions. Alternatively, a volume-preset, time-cycled device may be used.50
In patients with acute hypercapnic respiratory failure, NIV by full face mask or nasal mask should be considered first-line therapy (see Chap. 44). Advantages of NIV over invasive ventilation in general include decreased need for sedation and paralysis, decreased incidence of nosocomial pneumonia, decreased incidence of otitis and sinusitis, and improved patient comfort. Disadvantages include increased risk of aspiration and skin necrosis, and less control of the patient’s ventilatory status compared with invasive ventilation.36,51
Although nocturnal NIV is firmly established in the management of KS patients with chronic respiratory failure,52,53 limited data are available regarding its efficacy in acutely ill patients.54-57 In one report of the use of noninvasive ventilation in 164 patients with heterogeneous forms of ARF, only five patients had restrictive lung disease.56 All five patients improved on noninvasive ventilation, although one subsequently required intubation. Noninvasive ventilation was also helpful in four patients with KS and ARF failing conventional medical therapy.57
Following the guidelines of Meduri and colleagues,56 we initiate NIV using a loose-fitting full face mask. We start with 0 cm H2O continuous positive airway pressure (CPAP) and 8 to 10 cm H2O pressure support, and increase CPAP to 3 to 5 cm H2O and pressure support to the level required to achieve an exhaled tidal volume ≥7 mL/kg and a respiratory rate ≤25/min, adequate gas exchange, and improved patient comfort.
Noninvasive negative pressure ventilators are not feasible in most acute situations because they generally require patients to lie flat and coordinate their breaths with the ventilator. Difficulties with fit and applying the device adequately to the distorted chest wall further complicate their use. Still, negative pressure ventilators have averted intubation in rare cases40 and have been used successfully in the long-term management of patients with KS.58
Intubation is indicated for cardiopulmonary arrest, impending arrest, refractory hypoxemia or hypercapnia, mental status changes, and shock. Intubation can be difficult because of spinal curvature and tracheal distortion, and because patients with small lung volumes desaturate quickly. Airway visualization with fiberoptic bronchoscopy may be useful in some cases. During the peri-intubation period, an FiO2 of 1.0 is desirable, although it should be decreased to nontoxic levels as tolerated once the patient has been stabilized on the ventilator. Decreasing oxygen consumption with sedatives, use of positive end-expiratory pressure (PEEP), and increasing Pˉv

Full access? Get Clinical Tree
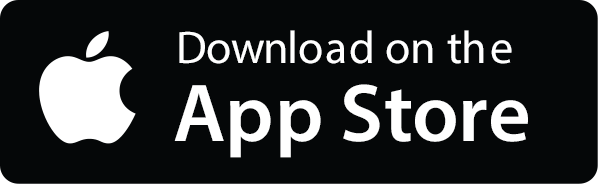
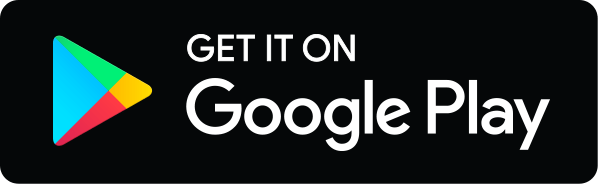
