KEY POINTS
Effective preventive measures in ventilated patients include raising the head of the bed, employing measures to prevent venous thromboembolism, avoiding unnecessary changes of the ventilator circuit, reducing the amount of sedation, and providing oral care with chlorhexidine.
Even patients with normal lungs may benefit from limited tidal volumes to reduce the risk of ventilator-induced lung injury causing progression to acute respiratory distress syndrome.
Critical illness and mechanical ventilation combine to impair strength of respiratory muscles and produce atrophy. This tendency can be reduced by setting the ventilator in a way as to preserve inspiratory muscle contraction.
Whenever the adequacy of oxygen exchange is in question, the initial fraction of inspired oxygen (FiO2FiO2) should be 1.0; this will be diagnostic as well as therapeutic, since failure to achieve full arterial hemoglobin saturation identifies a significant right-to-left shunt.
The choice of ventilator mode is relatively unimportant: more relevant is to use the ventilator with full understanding of the principles of lung protection, ventilator-induced diaphragm dysfunction, autoPEEP, and patient-ventilator synchrony.
The patient with severe airflow obstruction often develops hypoperfusion after institution of positive-pressure ventilation as a result of autoPEEP; this responds to temporary cessation of ventilation and vigorous volume resuscitation, while measures are employed to reduce airflow obstruction and reduce the total minute ventilation.
The patient with acute hypoxemic respiratory failure (AHRF) resulting from pulmonary edema benefits from lung-protective ventilation (6 mL/kg ideal body weight and rate approximately 30 breaths/min). The initial FiO2 of 1.0 can be lowered to nontoxic levels by raising positive end-expiratory pressure (PEEP), guided by pulse oximetry or measures of recruitment.
Regardless of the underlying process leading to mechanical ventilation, several principles guide ventilator settings and associated management. This chapter emphasizes preventing complications by using the “ventilator bundle”; avoiding lung injury (through overdistention or autoPEEP); limiting ventilator-induced diaphragm dysfunction (VIDD); understanding cardiopulmonary interactions; choosing modes and settings in relation to the underlying cause of respiratory failure; ensuring synchrony between patient and ventilator; and responding to crises.
Other chapters of this book are complementary to the information presented here. The pathophysiology of respiratory failure is broadly reviewed in Chap. 43; monitoring respiratory system waveforms of pressure and flow is delineated in Chap. 48; noninvasive ventilation is covered in Chap. 44; ventilator-induced lung injury is discussed in Chap. 51; and finally, several chapters (eg, Chap. 52, Acute Respiratory Distress Syndrome; Chap. 54, Acute on Chronic Respiratory Failure; Chap. 55, Status Asthmaticus; Chap. 58, Restrictive Disease of the Respiratory System) discuss ventilatory support for specific problems.
PREVENTION: THE “VENTILATOR BUNDLE”
Mechanically ventilated patients are at risk for numerous complications related to the presence of the endotracheal tube, most notably ventilator-associated pneumonia (VAP), as well as adverse consequences of sedatives, paralytics, and immobilization. In response, the Institute for Healthcare Improvement promulgated the concept of “bundles,” a structured set of processes that, when performed collectively and reliably, improve outcomes (head of bed elevation; daily sedative interruption and readiness assessment; and steps to prevent venous thromboembolism and gastrointestinal hemorrhage). Ideally, these are evidence-based interventions but, in actual fact, include tactics with an uncertain impact on VAP. Effective preventive measures are more fully discussed in Chaps. 3, 4, 5, and 22, but are briefly summarized here.
Noninvasive ventilation should be used in appropriate candidates, since this reduces the risk of VAP in COPD patients1 and perhaps those in an immunocompromised state.2 For intubated patients, the head of the bed should be elevated to 30° to 45°. This intervention may3 or may not4 be effective in reducing VAP, but has the virtues of being simple, inexpensive, and logical, since much VAP is thought to arise from aspiration of gastric contents. Ventilator tubing should be changed only when the tubing is visibly soiled or malfunctioning, rather than on a time-based schedule.5 Sedative use should be limited and patients should be allowed to wake up daily, as discussed below and in Chap. 22. Ideally, the “wake-up” should be coordinated with a spontaneous breathing trial (SBT) to judge readiness for extubation.6 Prophylaxis against venous thromboembolism is indicated in most patients. Oral care with chlorhexidine is probably effective.7 There is no consensus regarding the effectiveness of selective decontamination of the digestive tract, subglottic suctioning, or avoidance of antiacid therapies for prevention of VAP.
VENTILATOR-INDUCED LUNG INJURY
When the lungs of patients with the acute respiratory distress syndrome (ARDS) are distended excessively, through high tidal volumes or high positive end-expiratory pressure (PEEP), injury follows. Local pulmonary inflammation ensues, including areas of previously healthy lung, and systemic inflammation is seen,8 potentially causing distant organ failures. Increasing evidence suggests that ventilated patients with normal lungs (having central nervous system failure, eg, or undergoing surgery) may also be at risk when large (12 mL/kg) tidal volumes are used9,10 (see Chap. 51). In light of these findings, tidal volume should probably be limited also in these patients as discussed more fully below.
VENTILATOR-INDUCED DIAPHRAGM DYSFUNCTION
Like skeletal muscles, the diaphragm suffers atrophy and contractile dysfunction during critical illness and mechanical ventilation, termed VIDD.11,12 This occurs acutely, worsens progressively, and is associated with prolonged ventilation and risk of death.13 Muscle protein synthesis is inhibited and multiple pathways of self-destruction are up-regulated.14 Also like in peripheral muscle, active contraction (ie, active breathing) can effectively modify the degree of catabolism, helping to maintain contractile function. This has potentially important implications for selection of mode, adjustment of settings based on patient-ventilator interaction, role for sedation and SBTs, and endpoints for ventilator settings. For example, these findings regarding VIDD suggest that the ventilator should generally be set so as to encourage patient triggering, rather than passivity (unless profound shock or hypoxemia prevents this). Further, adjusting ventilator settings to achieve a modest degree of patient effort should perhaps override alternative strategies, such as those based on standard protocols or relying on arterial blood gas analysis. It is also interesting to speculate to what degree daily sedative interruption, spontaneous breathing trials, and noninvasive ventilation exert their beneficial effects through reductions in VIDD.
CARDIOPULMONARY INTERACTIONS
Cardiopulmonary interactions describe the complex, and mutually important, relationships between respiration (and mechanical ventilation) and the circulation, largely because these systems are deeply intertwined within the thorax. Circulatory abnormalities and treatments have implications for lung function. At the same time, lung injury, ventilation, and PEEP can support or cripple the circulation.
Mechanical ventilation affects the circulation through cyclic changes in the pleural pressure (Ppl), direct effects on the pulmonary circulation and right ventricular afterload, and indirect consequences of altered gas exchange and work of breathing. In contrast to spontaneous breathing when the Ppl falls during inspiration, mechanical ventilation tends to make Ppl rise. Following institution of or changes in mechanical ventilation, the increment in Ppl relates to patient effort (both inspiratory and expiratory), tidal volume, chest wall compliance, and the magnitude of any alveolar recruitment. Higher tidal volumes and a stiffer chest wall produce greater changes in Ppl and accordingly greater effects on the circulation. PEEP has a similar impact, except that the degree of Ppl augmentation depends on the magnitude of PEEP, the lung and chest wall compliances, and whether lung is recruited or not: more recruitment causes a greater change in Ppl. The dominant circulatory impact of ventilation tends to be mediated through changes in Ppl (since this largely determines the juxtacardiac pressure), most notably by reducing right ventricular preload. Impaired right heart filling accounts for much of the hemodynamic depression of positive pressure ventilation and PEEP, although right ventricular afterload plays a role in some, especially those with severe ARDS.15 Modes of ventilation that preserve spontaneous breathing dampen the rise in average pleural pressure and may be associated with less circulatory depression.
Ventilation can support the circulation, too, by raising left ventricular preload, reducing afterload, and easing the work of breathing. Similarly, withdrawing ventilation or PEEP can challenge the circulation, especially in those with severe left ventricular dysfunction, impeding liberation from the ventilator.16
CHOOSING A VENTILATOR MODE
Technological innovations have provided a plethora of modes by which a patient can be mechanically ventilated. These have been developed with the hope of improving gas exchange, patient comfort, or speed of return to spontaneous ventilation. Aside from minor subtleties, however, nearly all modes allow full rest of the patient, on the one hand, or substantial exercise, on the other, while providing a suitable foundation for maintaining gas exchange and protecting the lung. Thus, in the great majority of patients, choice of mode is merely a matter of patient or physician preference. Noninvasive ventilation should be considered before intubation and ventilation in many patients who are hemodynamically stable and do not require an artificial airway, especially those with acute-on-chronic respiratory failure, postoperative respiratory failure, and cardiogenic pulmonary edema. Management of the patient ventilated noninvasively is discussed thoroughly in Chap. 44.
Choosing a ventilatory mode and settings appropriate for each individual patient depends not only on the physician’s goals (rest vs exercise; lung protection; limitation of autoPEEP; gas exchange), but also on knowledge of the mechanical properties of the patient’s respiratory system. Determining respiratory system mechanics is an integral part of ventilator management and a routine component of examination of the critically ill patient, discussed fully in Chap. 48 (Ventilator Waveforms). The intensivist can combine clinical information, chest radiography, lung ultrasound, and respiratory system mechanical properties to categorize the patient into one of four prototypes: (1) normal gas exchange and mechanics; (2) significant airflow obstruction (as in status asthmaticus or acute exacerbations of COPD); (3) ARDS; and (4) restriction of lung or chest wall. Appropriate initial ventilator settings and subsequent adjustments for each of these four states are discussed later in this chapter.
If full rest of the respiratory muscles is desired, it is incumbent on the physician to ensure that this is indeed achieved. Although some patients are fully passive while being ventilated (those with deep sedation, some forms of coma, metabolic alkalosis, sleep-disordered breathing), most patients will make active respiratory efforts, even on volume assist-control ventilation (VACV),17 at times performing extraordinary amounts of work. Unintended patient effort can be difficult to recognize but, aside from obvious patient effort, may be signaled by an inspiratory fall in intrathoracic pressure (as noted on a central venous or pulmonary artery pressure tracings or with an esophageal balloon), by triggering of the ventilator, or by a careful analysis of real-time flow and pressure waveforms, discussed more fully in Chap. 48. When there is evidence of unwanted patient effort, ventilator adjustments, psychological measures, pharmacologic sedation, and therapeutic paralysis can be useful. Ventilator strategies to reduce the patient’s work of breathing include increasing the minute ventilation to reduce PaCO2 (although this may run counter to other goals of ventilation, especially in patients with ARDS or severe obstruction), increasing the inspiratory flow rate, and changing the mode to pressure-targeted ventilation, as in pressure-support ventilation (PSV) mode or pressure assist-control ventilation (PACV).
For most patients, however, some degree of triggering and work are desired because this is likely to reduce the degree of VIDD as described above. If some work of breathing on the patient’s part is desired, this can be achieved through any of the existing ventilatory modes. The amount of work done may be highly variable, however, and depends on the specific mode, the settings chosen within each mode, and the interaction between the patient and the ventilator. A recurring question during the time when a patient can carry some of the work of breathing is, “Can this patient breathe without ventilatory assistance?” This issue is more fully developed in Chap. 60, Liberation From Mechanical Ventilation.
The terminology describing modes of ventilation can be very confusing and may vary from one company’s ventilator to another. In this chapter, we refer to volume-targeted modes as those in which the physician sets a desired tidal volume that the ventilator delivers, using whatever pressure is required, and pressure-targeted modes, in which the physician sets a desired pressure that the ventilator maintains, delivering a volume that depends on the settings, respiratory mechanics, and patient effort. Some modern modes (dual control modes, see below) attempt to blend pressure and volume targets. Few studies have compared modes directly except with respect to comfort, a measure generally favoring pressure-targeted modes. At the same time, comparative trials are plagued by the details of settings and these are often dissimilar between modes (biasing the study) or are not sufficiently specified in the methods. For this reason, modes are often chosen based on preference, personal experience, or institutional practice, rather than on evidence relating to meaningful outcomes.
The differences between volume-targeted and pressure-targeted modes are fewer than often appreciated, since volume and pressure are related through the mechanical properties of the respiratory system, most notably the respiratory system compliance (Crs). For example, a passive patient with a static Crs of 50 mL/cm H2O ventilated on VACV at a tidal volume of 500 cc with no PEEP (or autoPEEP) will have a plateau airway pressure (Pplat; see Chap. 48) of about 10 cm H2O, whereas the same patient ventilated on PACV at 10 cm H2O can be expected to have a tidal volume (VT) close to 500 cc. The difference to the patient between these modes may be quite trivial, often amounting to small differences in inspiratory flow profile. Thus, while physicians’ comfort level with volume- targeted and pressure-targeted modes may be very different, the modes can be similar because they are tied to each other through the patient’s Crs.
In the following descriptions, each mode is first illustrated for a passive patient, such as following muscle paralysis, and then for the more common situation in which the patient plays an active role in ventilation. On some ventilators tidal volume (VT) can be selected by the physician or respiratory therapist, whereas on others a minute ventilation and respiratory rate (f) are chosen, secondarily determining the VT. Similarly, on some machines an inspiratory flow rate (˙V) is selected, whereas on others ˙V depends on the ratio of inspiratory time to total respiratory cycle time (TI/TT) and f; on inspiratory-expiratory (I:E) ratio and f; or on rise-time and other parameters.
Pressure-Targeted Modes: In pressure-targeted modes, a fixed inspiratory pressure (PI) is applied to the patient, whatever the resulting VT. Depending on the particular ventilator, the physician may have to specify the actual level of PI or, alternatively, the increment in pressure over the expiratory pressure (PEEP). Ventilators designed primarily for noninvasive ventilation often require setting PI and PEEP independently, while most ICU ventilators require setting the PEEP and an inspiratory pressure increment. For example, the following settings are identical: 1. PI = 20 cm H2O; PEEP = 5 cm H2O (noninvasive ventilator); or 2. Pressure increment (eg, “pressure-support” or “pressure-control”) = 15 cm H2O; PEEP = 5 cm H2O. In this chapter, we will specify inspiratory (PI) and expiratory (PEEP) pressures to avoid confusion.
In pressure-targeted modes, the VT is predictable (again, passive patient) when the Crs is known:
assuming time for equilibration between PI and alveolar pressure (Palv) and the absence of autoPEEP (both of these assumptions are often not true in patients in the ICU; see below).
Compared with volume-targeted modes, a potential advantage of pressure-targeted ventilation is greater physician control over the maximal alveolar pressure (PI) in passive patients, although it should be emphasized that a “safe” maximal alveolar pressure is not known. Further, when patients are active, PI does not represent the transpulmonary pressure, meaning that gross overdistention of lung is possible on pressure-targeted modes despite modest ventilator pressures. In addition, the same reduction in maximal alveolar pressure can be achieved using volume-targeted modes, simply by limiting tidal volume, as has been shown in ALI/ARDS patients.18 Nevertheless, pressure-targeted modes make such a lung protection strategy easier to carry out by dispensing with the need to repeatedly determine Pplat and periodically adjust the VT.
Pressure-targeted modes also allow the patient greater control over inspiratory flow rate and therefore potentially increased comfort. On the other hand, during lung protective ventilation, pressure modes (including pressure-regulated volume control, see below) did not reduce work of breathing compared to volume assist-control and did not allow precise control of tidal volume.19 A disadvantage of pressure-targeted modes is that changes in respiratory system mechanics (eg, increased airflow resistance or lung stiffness) or patient effort may decrease the minute ventilation, necessitating alarms for adequate ventilation. Also, the mechanics cannot be determined readily and partitioned as described in Chap. 48 without switching modes, inserting an esophageal balloon, or using more complex algorithms.20
Pressure Assist-Control Ventilation (PACV) In the passive patient, ventilation is determined by f, the inspiratory pressure increment (PI − PEEP), inspiratory to expiratory (I:E) ratio, and Crs. In patients without severe obstruction given a sufficiently long TI, there is equilibration between the ventilator-determined PI and Palv so that inspiratory flow ceases (Fig. 49-1A). In this situation, tidal volume is highly predictable, based on PI (= Palv) and the mechanical properties of the respiratory system (Crs). In the presence of severe obstruction or if TI is too short to allow equilibration between ventilator and alveoli, VT will fall below that predicted based on PI and Crs (see Fig. 49-1A). One of the advantages of PACV is that it may facilitate ventilation with a lung protective strategy. For example, alveolar overdistention can be prevented by ensuring that Palv never exceeds some threshold value (this is often taken to be 30 cm H2O, but a truly safe level is unknown) by simply setting PI (alternatively, PEEP + PSV) to the desired upper limit. Inspiratory activity can raise the transpulmonary pressure well above a safe level, despite a modest PI, threatening lung protection. During PACV, TI and f are set by the physician and may not approximate the patient’s desired TI and f.
FIGURE 49-1
A. Pressure-control ventilation of a muscle-relaxed patient showing the effects of changed inspiratory resistance. The left-hand panel shows a pressure-control breath with normal resistance, during which PI equilibrates with Palv before the inspiratory cycle is terminated (left arrow), flow ceases, and tidal volume can be predicted from the PI and Cst (VT = Cst × PI − PEEP). In the right-hand panel, inspiratory resistance is elevated. Note that at the same PI, inspiratory flow is reduced, the tidal volume is not reached until the inspiratory phase is terminated (right arrow), and the tidal volume (solid line) falls below that predicted by Cst and PI − PEEP (dotted line). Pao is airway opening pressure; is flow; V is volume. B. Pressure-support ventilation. When a breath is triggered Pao rises to the set level (PI) with flow and VT depending on the PI − PEEP, respiratory system mechanics, and patient effort. The first breath shown represents a patient who triggers the ventilator and then remains fully passive (a hypothetical circumstance used here for contrast with the usual patient efforts shown in the next two breaths). As long as there is no significant airflow obstruction, VT nearly reaches the volume that would be predicted based on the compliance of the respiratory system (VT = Crs * PI − PEEP). During the middle breath shown, the patient makes a moderate but prolonged inspiratory effort. The Pao remains at the set inspiratory level as long as patient effort maintains flow, and a much longer TI and VT result. In the final breath, a more powerful but briefer inspiratory effort is made, shortening the TI but generating a larger VT than during the passive breath. Pao is airway opening pressure;
is flow; and V is volume. C. Airway opening pressure (Pao) and lung volume (V) during VACV ventilation of a patient who is periodically triggering the ventilator. The second breath was set to be delivered at the time marked by the second arrow; instead, the patient lowers the Pao, triggering the ventilator at the time marked by the first arrow, thereby increasing the respiratory rate above the default value, decreasing the expiratory time (TE), and increasing the I:E ratio. D. Airway opening pressure (Pao), flow (
) and lung volume (V) during SIMV. Breath 1 (a mandatory breath) is not triggered by the patient, who remains fully passive. V and
are determined by the ventilator, while the Pao reflects the passive mechanical characteristics of the respiratory system. The shaded rectangle near the second breath denotes the interval during which the ventilator is programmed to synchronize with the patient’s inspiratory effort, delivering the mandatory breath slightly ahead of schedule. At the end of this time interval (arrow), a mandatory breath would have been delivered (dotted tracing) if the patient had not triggered the ventilator. The synchronized breath (breath 2) has the same volume and flow as a mandatory breath. The Pao may not be the same as during a passive breath because of continued patient effort throughout inspiration. The third breath (3) is initiated before the synchronization interval at x and is therefore not assisted. Flow and tidal volume are totally determined by the patient’s effort and mechanics. These breaths are typically shorter and smaller (as indicated) than the mandatory breaths. When the patient fails to trigger another breath within the next synchronization window, another mandatory breath (4) is delivered.
When the patient is active, the tidal volume reflects patient effort and the patient may trigger additional breaths. When the patient makes inspiratory efforts synchronized with machine inspiration, the tidal volume is generally greater than that predicted from the Crs and PI and may exceed targets for lung-protection. However, dyssynchrony or expiratory effort during machine inspiration may reduce VT below that otherwise expected. Special care must be taken to adjust TI to the individual patient (Fig. 49-2); otherwise, heavy sedation is typically needed. When unphysiologic settings are intentionally chosen, as when the physician desires an unusually long TI (TI longer than TE results in inverse ratio ventilation, IRV), deep sedation or therapeutic paralysis is often given.
FIGURE 49-2
The effect of changing TI on ventilation in the passive patient during PACV. As TI is raised from an initial value of 0.5 second (first two breaths) to 1.0 second (middle breaths) to 1.5 seconds (last breaths) tidal volume increases; TE falls so much that autoPEEP is created (once TI reaches 1.0 second); and end-inspiratory Palv rises (signaled by the lower flow at end-inspiration—essentially zero at TI 1.5 seconds).
Pressure-Support Ventilation (PSV) The patient must trigger the ventilator in order to activate this mode, so PSV is not applied to passive patients. Ventilation is determined by PI − PEEP, patient-determined f, patient effort, and the patient’s mechanics. Once a breath is triggered, the ventilator attempts to maintain PI using whatever flow is necessary to achieve this. Eventually, flow begins to fall due to cessation of the patient’s inspiratory effort combined with increasing elastic recoil of the respiratory system as lung volume rises. The ventilator maintains PI until inspiratory flow falls an arbitrary amount (eg, to 20% of initial flow) or below an absolute flow rate (set by default or user-configured).
It is useful to first consider what happens if the patient were to trigger the ventilator and then remain passive (an artificial situation). Tidal volume would be determined by PI and the (largely static) mechanical properties of the respiratory system, as during PACV (see Fig. 49-1B). More typically, the patient makes an effort throughout inspiration, in which case VT is determined, in part, by the degree of effort (see Fig. 49-1B). At a constant minute ventilation the patient’s work of breathing can be increased by lowering PI and can increase inadvertently if respiratory system mechanics change despite no change in ventilator settings. Respiratory system mechanical parameters cannot be determined readily on this mode because the ventilator and patient contributions to VT and ˙V are not distinguishable from analysis of the ventilator airway opening pressure (Pao); accordingly, the important measurements of Pplat, Ppk minus Pplat, and autoPEEP are measured during a brief daily switch from pressure-support to VACV at the corresponding values of VT, ˙V, and I:E observed during PSV.
A potential advantage of PSV is improved patient comfort. An important caution about PSV is that it can account for a large fraction of total minute ventilation, even when set at rather low levels, as in patients with normal respiratory system mechanics. For example, in a patient with myasthenia gravis, 10 cm H2O of PSV may represent full mechanical ventilation. A “successful” spontaneous breathing trial on these settings should not be used to judge the patient’s readiness for extubation.
Volume-Targeted Modes: During volume-targeted ventilation, a volume is delivered to the patient whatever the pressure required (within the limits of the high pressure alarm). The physician generally also sets an inspiratory flow rate (indirectly determining the TI) as well as f. In volume-targeted modes, the Pplat is predictable (again, passive patient) when the Crs is known:
where PEEP includes also autoPEEP.
Compared with pressure-targeted modes, a potential advantage of volume-targeted ventilation is greater control over the total minute ventilation, since VT does not depend on potentially changing patient effort or respiratory system mechanical properties. Also, it is easy to characterize the respiratory system mechanics by measuring Ppk and Pplat, thereby helping to follow the patient’s progress or response to therapies.
Volume Assist-Control Ventilation (VACV) The set parameters of the volume assist-control mode are the inspiratory flow rate (˙V), frequency (f), and tidal volume (VT). (On some ventilators, one must set the total minute ventilation and rate, thereby determining tidal volume and indirectly determining ˙V). In the passive patient, the ventilator delivers f [f = 60 s/(TI + TE)] equal breaths per minute, each of VT volume. VT and ˙V determine the inspiratory time (TI), expiratory time (TE), and the inspiratory-expiratory (I:E) ratio. Pplat is related to the VT and the compliance of the respiratory system, whereas Ppk-Pplat includes contributions from ˙V and inspiratory resistance (see Fig. 49-1C).
The active patient can trigger extra breaths by exerting an inspiratory effort exceeding the preset trigger sensitivity, each at the set VT and ˙V, and thereby change TI, TE, and I:E ratio and (potentially) create or increase autoPEEP. Typically, each patient will display a preferred rate for a given VT

Full access? Get Clinical Tree
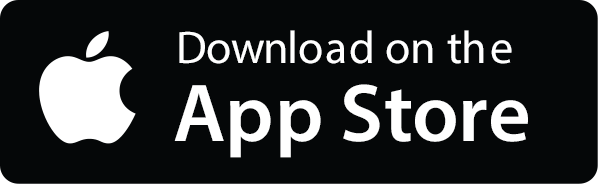
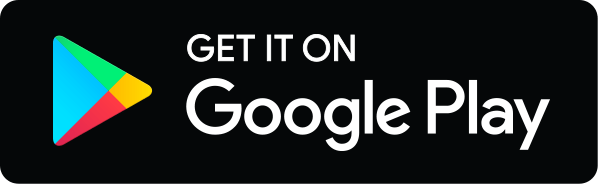
