KEY POINTS
The neuroanatomy of coma can be divided into three major categories: diffuse brain dysfunction or bithalamic injury, primary brain stem disorders, and secondary brain stem compression from supratentorial and infratentorial mass lesions.
Most cases of coma are due to metabolic disorders or exogenous drug intoxication.
Patient evaluation must follow an orderly sequence, beginning with vital signs, general physical examination, and neurologic examination.
The neurologic examination of the patient in coma is brief and focuses on (1) level of consciousness, (2) pupils, (3) eye movements, (4) motor responses, and (5) respiratory pattern.
Computed tomographic (CT) scanning of the brain is the most valuable acute test to rule out structural causes of coma.
Hypoxic-ischemic encephalopathy after cardiopulmonary arrest may be ameliorated by targeted hypothermia and supportive measures.
Serial neurologic examination over the first 72 hours is most helpful to determine the prognosis for patients with atraumatic coma; for anoxic brain injury, failure to recover pupillary responses or corneal reflexes in the first 72 hours is a poor prognostic sign.
As therapies aimed at cerebral resuscitation and preservation following acute injury are developed and proved efficacious, prior guidelines for determining prognosis will require redefinition and reconfirmation.
The Uniform Determination of Death Act states that, “An individual who has sustained either (1) irreversible cessation of circulatory and respiratory functions, or (2) irreversible cessation of all functions of the entire brain, including the brain stem, is dead.”
The determination of death by brain criteria is based on clinical examination, and in most cases does not require confirmatory tests. However, the cause of coma must be known, and the cause must be sufficient to explain irreversible cessation of whole brain function. The new AAN guidelines recommend a single clinical neurological examination.
NORMAL AND IMPAIRED CONSCIOUSNESS: A CONCEPTUAL APPROACH
Consciousness is a difficult term to define, and even more complicating is the fact that many different meanings and classification systems exist for the various states of decreased level of consciousness, such as drowsiness, stupor, and coma. For practical reasons, however, in the evaluation of consciousness most clinicians give greater weight to the patient’s responses and behavior than to what the patient says. Hence, consciousness can be defined in its simplest form as the patient’s awareness of self and environment and the responsiveness to his or her needs and external stimulation. The level of consciousness used in clinical practice refers to the state of arousal and should be separated from the content of consciousness, which describes various forms of cognitive behaviors and thinking. An awake person is fully responsive (alert) to stimuli and is able to specify their extent of awareness of self and environment.
Impaired consciousness is generally categorized by the level of responsiveness to external and internal stimuli (Table 88-1). Sleep and pathologic states of consciousness undeniably share some common features; for example, the sleeping person is not aware of himself or herself and in this respect is unconscious. Of course, the important difference is that a sleeping person can be aroused to full consciousness. Furthermore, electrodiagnostic evaluation differs in the two conditions (see below) and cerebral glucose uptake does not decrease during sleep but does so in coma. Drowsiness (“lethargy”) is a state of reduced spontaneous physical and mental activity. A drowsy person cannot sustain wakefulness without external stimulation. It is similar in appearance to light sleep and almost always accompanied by reduced attention, concentration, and mild confusion.
A stuporous patient appears asleep and requires repeated, stronger stimuli to arouse, yet may not achieve full state of arousal and quickly drifts back to persistent inactivity when the stimulus is withdrawn. When aroused, this patient may or may not open their eyes and partially respond to requests. At times, restlessness and motor stereotypes are observed. Sopor, the Latin word for deep sleep and a term used in some European countries, denotes an intermediate state between stupor and coma.
Delirium is characterized as a reduction in clarity of mentation accompanied by cognitive changes such as memory deficit, altered speech, or orientation. Its duration of onset is hours or days and there is fluctuation in severity during its course. Disorientation affects time followed by place and lastly people in the environment. There may be an associated anxiety state or agitation. Delirium typically implies diffuse impairment of bilateral cortical structures usually by a toxic-metabolic process and it is typically reversible.
Dementia is a chronic condition in which content of consciousness is affected without effect on level of arousal, except in severe terminal cases. It is generally progressive and affects memory and at least two cognitive domains such as language, executive function, planning motor tasks, and recognition. Dementia is caused by damage to the cerebral hemispheres by degenerative diseases, traumatic brain injury, hydrocephalus, etc.
Coma (from the Greek komas, or deep sleep) is a state of unresponsiveness in which the patient is incapable of arousing to external or internal stimuli (lack of alertness). The degree of coma can vary from lighter stages (also denoted as semicoma) with observed changes in autonomic function or brief moaning to strong stimulation, to the deepest stage with absence of any brain stem responses (eg, pupillary and corneal reflexes), cyclic autonomic activity, and motor tone. For in-depth discussions of this topic, the reader is referred to the major textbooks in neurology and to the seminal work by Posner et al.1
A vegetative state can follow coma and identifies a state in which the brainstem and diencephalic (thalamic) activity is present to a degree that clinical signs of spontaneous arousal are observed; however, clinical evidence of awareness of self and environment is lacking.2 The patients often show blink responses to light; intermittent eye movements (sometimes erroneously interpreted as following objects or looking at family members); stimulus-sensitive automatisms such as swallowing, bruxism, and moaning, as well as primitive motor responses and cycles of sleeping and waking. If this state lasts longer than 30 days, it is referred to as persistent vegetative state (PVS) and is used as a descriptive clinical syndrome rather than a disease-specific entity. The most common causes include cardiac arrest, head trauma, severe brain infections, and various causes of thalamic necrosis. Vegetative states can also be seen in the terminal phase of degenerative illnesses such as Alzheimer disease. Ambiguous terms for PVS such as apallic syndrome and neocortical death should be avoided.3,4
Minimal conscious state can be diagnosed in patients displaying inconsistent behavioral evidence of awareness of the environment, but they cannot communicate and are unable to follow instructions reliably5 (Table 88-2). It describes a large group of patients who are different from vegetative patients in that they demonstrate some signs of awareness of their selves and their surroundings albeit inconsistently. The inconsistency may be very subtle or more pronounced wherein unless observed for a long periods of time, it is almost impossible for a clinician to determine otherwise.
Characteristics of the Persistent Vegetative State (PVS) and Related Conditions
Condition | Self-Awareness | Sleep-Wake Cycles | Motor Function | Experience Suffering | EEG Activity |
---|---|---|---|---|---|
PVS | Absent | Intact | No purposeful movement | No | Polymorphic delta or theta, sometimes slow alfa |
Coma | Absent | Absent | No purposeful movement | No | Polymorphic delta or theta |
Brain death | Absent | Absent | None or only spinal reflex movements | No | Electrocerebral silence |
Locked-in syndrome | Present | Intact | Quadriplegia and pseudobulbar palsy; eye movements preserved | Yes | Normal or minimally abnormal |
Akinetic mutism | Present | Intact | Paucity of movement | Yes | Nonspecific slowing |
Akinetic mutism is a manifestation of hypothalamic or basal forebrain injury, which manifests as apparent depressed levels of consciousness in a patient with well-formed sleep-wake cycles, with no external evidence of awareness or spontaneous motor activity. It is imperative in such instances to have a rigorous neurological examination as well as a careful review of neuroimaging and EEG. Abulia is a state in which the patient is awake, has normal sleep wake cycle, and is very slow to respond to stimuli. Mental function is usually normal when tested with sufficient stimulation. It is secondary to bilateral frontal lobe disease and in severe instances may mimic or progress to akinetic mutism.
Clinical practice teaches that consciousness should be viewed as a continuum between different pathological conditions and not as an all-or-none phenomenon, and that it is frequently difficult to identify definite and consistent signs of conscious perception of environment and self in patients with severe brain injuries.1 The latter limits the diagnostic certainty of remaining brain function on clinical grounds since the identification of consciousness relies purely on the deduction whether consciousness is present or absent in a particular patient.2 To this end the assessment is further complicated by the fact that responses sought along different domains of awareness are summarized by the patient’s motor manifestations.
Extensive and severe neuronal damage is causally related to loss of consciousness in patients with PVS, a finding supported by a study identifying decreased cortical radiolabeled flumazenil uptake (a benzodiazepine antagonist and neuronal marker).6 In corroboration, several studies consistently identified decreased cerebral glucose metabolism and blood flow.7,8 However, brain regions with the most consistent decrease in cerebral glucose consumption in patients in PVS are the polymodal association areas of the frontal, temporal, and parietal lobes9; interestingly, normal subjects display higher brain metabolism during wakefulness than in sleep in similar regions.10 Furthermore, thalamocortical disconnections can be identified using auditory and sensory external stimulations.11
Some carefully performed studies identified patients with PVS who activate primary and associative cortex, depending on the complexity and familiarity of the test stimuli.12,13 Recent data obtained in patients with PVS reveal functional magnetic resonance imaging (fMRI) activation of the supplementary motor area (SMA) and parahippocampal areas in motor planning and visuospatial task stimulation paradigms, respectively.14 It is, however, unclear whether the identified activations represent consciousness since no conclusions to the connectivity of thalamocortical brain regions and larger neuronal networks can be drawn. Similarly, brain plasticity with recovery of functional thalamocortical connections and reestablishment of neuronal networks may allow certain patients to regain consciousness after severe brain injury and PVS.9 In recent years, we have gained much insight into which brain areas seem necessary for conscious experience; however, future research should broaden our knowledge about what form of brain activity in these areas confers consciousness.
IMPAIRED CONSCIOUSNESS: AN ANATOMIC APPROACH
Because coma is a sleep-like state, it is not surprising to find that the neuroanatomy of coma is closely related to brain stem centers that regulate daily cycles of wakefulness and sleep: the reticular activating system (RAS). In animals the RAS lies within the center of the brain stem, extending from the midbrain into the hypothalamus and thalamus.15,16 Lesions in the pathways of the brain stem reticular formation or RAS have the greatest impact on changes in consciousness.
The RAS is a loosely organized core of polysynaptic neurons reaching in the brain stem from the lower medulla through the paramedian pons to central midbrain. From here, the RAS projects into the diencephalon to several functionally related nuclei in the thalami (especially the medial thalamus). Further cerebral projections are prominent to the inferomedial frontal lobes, but reach almost all parts of the cerebral cortex. The essential role of the RAS is arousal and maintenance of wakefulness; injury leads to reduction or failure of arousal. As the brain stem RAS receives direct spinothalamic information, incoming sensory stimulations are not only projected to the sensory cortices, but are also needed to activate the brain stem RAS for the maintenance of consciousness. Within the brain stem and thalamus, the RAS is confined to rather small anatomic areas; therefore, even small lesions can severely impair arousal and consciousness. In contrast, RAS fibers are sparse and spread out as they move toward the cerebral hemispheres, hence only larger cortical lesions will lead to impaired consciousness at that level.
Lesions that affect consciousness therefore can be grouped as supratentorial lesions that compress or injure cortical projections in either hemispheres or deep diencephalic nuclei, and infratentorial lesions that affect the RAS fibers in the brainstem. The clues to the location of a structural lesion are presented by the focal deficit that may be produced by injury to immediate structures in the vicinity of RAS fibers. Supratentorial lesions involve focal motor/sensory systems, whereas infratentorial lesions are accompanied by cranial nerve palsies as well as motor deficits.
Lesions in the lower pons and medulla need to be quite large to induce significant coma (loss of arousal) since the RAS is rather thinned in these regions. More commonly, lesions in the ventral pons (such as basilar artery occlusions) lead to severe motor pathway injury sparing somatosensory and ascending RAS (arousal) systems. This state is referred to as locked-in syndrome or de-efferented state, as the patient has preserved consciousness. However, the patient cannot speak or respond and is unable to move cranial, trunk, or extremity muscles, but retains the ability for vertical gaze and eye blinking. In contrast, vascular occlusions of the top of the basilar artery or the posterior cerebral arteries result in injuries to the midbrain or medial thalami, which lead to significant impairment of consciousness but none or only minimal focal neurologic findings. Traumatic brain injury with diffuse axonal injury can lead to petechial hemorrhages and ischemia or inflammatory to necrotizing lesions of the midbrain, resulting in impaired consciousness.
Cerebral masses can produce either direct or indirect (uncal or tentorial herniation) displacement and torsions of the midbrain and reduced alertness. Sudden injury to either or both cerebral hemispheres may produce impaired consciousness, indicating that wakefulness has no hemispheric dominance and requires some cerebral function. Bilateral, extensive acute or subacute damage to the cortex and white matter, for example, due to trauma, hypoxia, or infection, impairs activation of the upper RAS, leading to impaired consciousness. However, focal large cortical (lobar) areas can be injured and initially not affect consciousness at all until secondary injury from swelling and bleeding occurs, as evidenced by patients with penetrating wounds of the cerebral hemispheres who remain fully awake. Similarly, degenerative disorders such as Alzheimer disease generally do not or only minimally affect the RAS and these patients remain fully awake. Hypersomnia refers to a condition of excessive drowsiness and sleep. It may occur in the setting of narcolepsy, hypothalamic disorders, sleep disorders, or psychiatric illness.
Several categories of consciousness-impairing mechanisms and lesions can be defined. First, one cause is an easily identifiable mass lesion compressing the upper RAS either directly or indirectly (such as tumor, abscess, meningitis, or hemorrhage); second, discrete lesions of the upper brain stem (examples are outlined above) may be the cause; and third, a larger group of patients includes those in whom suppression of the RAS is induced by metabolic derangements, toxic states, or seizures. Such functional causes of coma may be reversible by correcting the underlying metabolic derangement or removing the offending drug. “Metabolic” coma is likely the most common etiologic category resulting in impaired consciousness in medical critical care units.
EXAMINATION OF THE COMATOSE PATIENT: A CLINICAL APPROACH
Acute depression in level of consciousness is a critical, life-threatening emergency that requires a systematic approach for evaluation of etiology. The causes of coma are myriad. Therefore, a reliable history should be obtained from family, witnesses, or medical personnel, and examination should seek representative localizing neurological and general physical findings.
Clues can be ascertained from the onset of coma. An acute onset in a previously healthy individual may indicate a cerebral vascular etiology (ie, subarachnoid hemorrhage, intracerebral hemorrhage, or hemispheric or brain stem stroke), generalized epileptic activity, traumatic brain injury, or drug overdose. Likewise, a subacute deterioration may point to systemic illness, evolving intracranial mass, or a degenerative, infectious, or paraneoplastic neurologic disorder. Moreover, the duration of a comatose state should be documented because it may have predictive value for prognosis in certain causes.
Careful clinical examination is irreplaceable by any investigation. While the assessment may take some period of time, a quick assessment of ABCs should be carried out in addition to basic resuscitation (Tables 88-3 and 88-4). A subsequent careful assessment should confirm and evaluate the presence and extent of impairment of consciousness and awareness. Core body temperature should be determined as hypothermia can be seen in drug overdose, brain death, or acute spinal cord transection. Moreover, hyperthermia can be seen in infection; traumatic brain injury; subarachnoid, intracerebral, or pontine hemorrhage; and hypothalamic dysfunction. If there is no evidence of neck trauma, signs of meningismus must be elicited.
Useful Physical Examination Findings in Comatose Patients
Exam Focus | Specific Features | Suggested Condition |
---|---|---|
Skin | Petechiae, splinter hemorrhage | Coagulopathy; SBE |
Icteric | Hepatic encephalopathy | |
Needle tracks | Drug overdose or withdrawal | |
Cyanotic | Hypoxemia | |
Lymph nodes | Adenopathy | Infectious etiologies; immunocompromised hosts |
Head | Contusion; postauricular ecchymosis (Battle sign) | Trauma |
VP shunt | Hydrocephalus; shunt malfunction | |
Eyes | Periorbital ecchymosis (raccoon eyes) | Trauma |
Papilledema | Increased intracranial pressure | |
Ears | Hemotympanum | Trauma |
Nose | Excessive discharge | Trauma |
Neck | Stiff | Subarachnoid hemorrhage, infection |
Enlarged thyroid | Dysthyroidism | |
Cardiovascular | Arrhythmia, etc | Hypoxic/ischemic encephalopathy |
Abdomen | Small hard liver | Hepatic encephalopathy |
Miscellaneous | Acetone, alcohol breath | Ketoacidosis; alcohol intoxication |
Fever | Infection | |
Tongue laceration; incontinence | Postictal state |
Neurologic Assessment of the Comatose Patient
Level of consciousness |
Arousability |
Content |
Brain stem function |
Respiratory rate and pattern |
Blood pressure and heart rate |
Pupil size and reactivity |
Eye position and movements |
Doll’s eyes maneuver |
Cold caloric testing |
Corneal reflexes |
Facial symmetry |
Motor function |
Posturing |
Tone |
Spontaneous movements |
Withdrawal to noxious stimulus |
Deep tendon reflexes |
Frequently the etiology of acute depression in consciousness in the hospitalized patient includes sepsis, acid-base, fluid, and electrolyte disorders, or hepatic, renal, or cardiac failure or seizures. Therefore, laboratory studies should be obtained to exclude metabolic and endocrine causes.
Neurologic Examination: The neurologic examination in a patient with depressed level of consciousness can be a valuable tool to localize the etiology. The important neurologic features include (1) level of consciousness, (2) respiratory pattern, (3) pupillary size and reactivity, (4) eye position and movements, (5) corneal reflexes, and (6) motor function.
Assessment of Consciousness The determination of the level of consciousness depends on analyzing arousal and content (see Table 88-1). Initially, one should observe whether the patient appears asleep or wakeful with spontaneous eye opening. In a sleeping patient, one should quantify how much stimulation (verbal, tactile, or noxious) is required to arouse the patient. Attempts should be made to elicit a behavioral response by verbal command alone. If no response is obtained, then physical stimulation should be used, first by shaking the patient. Then noxious stimulation can be applied by digital pressure to the supraorbital nerves or nail beds of the fingers or toes. Care should always be taken not to use stimuli severe enough to cause bruising. Purposeful attempts by the patient to remove the offending stimulus indicate preservation of brain stem function and intact connections to the cerebral hemispheres. Eye opening, either spontaneous or in response to stimulation, indicates preserved function of the RAS in the upper brain stem and hypothalamus. Once aroused, the patient’s ability to remain wakeful and respond coherently is determined.
Lethargy (or drowsiness), stupor, and coma represent different points on a continuum of decreasing levels of consciousness. Patients in these states appear to be sleeping with eyes closed. In contrast, patients with akinetic mutism and locked-in syndrome appear to be awake with eyes opened.
The Glasgow Coma Scale (Table 88-5) is used to assign a numerical description of consciousness. The scale was devised to evaluate patients with head injury and is most reliable and reproducible in trauma patients.17,18 Its application in nontraumatic conditions is less reliable, but it is still the most widely used clinical scale to evaluate the level of consciousness. Furthermore, it provides a reproducible tool to monitor progression. Another scale has recently been adopted by neurointensive care units across the country. The FOUR score includes more neurological details and has higher predictability for in-hospital mortality (Table 88-6).19
The Glasgow Coma Scalea
Response | Points |
---|---|
Eye opening | |
Spontaneously | 4 |
To speech | 3 |
To pain | 2 |
Never | 1 |
Best verbal response | |
Oriented | 5 |
Confused | 4 |
Inappropriate | 3 |
Garbled | 2 |
None | 1 |
Best motor response | |
Obeys commands | 6 |
Localizes pain | 5 |
Withdrawal | 4 |
Abnormal flexion | 3 |
Extension | 2 |
None | 1 |
15 |
Pupillary Changes in Coma
Size | Reactivity | Comments |
---|---|---|
Bilateral | ||
Normal or small | Normal | Toxic-metabolic disturbance |
Midposition (3-5 mm) | Poor | Midbrain dysfunction; drugs (glutethimide [Doriden]) |
Small (pinpoint) | Poor | Pontine dysfunction; drugs (narcotics) |
Large | Poor | Toxic-metabolic disturbance (anoxia); drugs (anticholinergics) |
Unilateral | ||
Large | Unreactive | Ipsilateral midbrain pathology or compression of ipsilateral CN III: uncal herniation, posterior communicating artery aneurysm |
Small | Minimal | Ipsilateral sympathetic dysfunction |
Respiratory Control The ventral respiratory group of neurons in the medulla generates the intrinsic respiratory rhythm with the dorsal group of neurons controlling the airway and respiratory reflexes. The respiratory motor control is also influenced by prefrontal cortex, which modulates continuous pattern; by parabrachial nucleus in the pons, which integrates respiratory movements with reflexes such as coughing and swallowing; and by the hypothalamus, which modulates respiration in relation to behavioral state. The cerebral cortex and forebrain are important in the control of regular respiration. Patients with isolated brain injury uncomplicated by other critical medical illnesses may have characteristic breathing patterns that aid in neuroanatomical localization (Fig. 88-1). However, these patterns are not reliable in patients with multiple organ system failure who are receiving mechanical ventilation. Nevertheless, a discussion is warranted.
Cheyne-Stokes respiration is a periodic breathing pattern in which periods of hyperpnea regularly alternate with apnea in a smooth crescendo-decrescendo pattern. This neurogenic respiratory alteration occurs with damage to the cortex and forebrain bilaterally, or secondary to cardiac or respiratory failure. It is the result of the loss of frontal lobe control over respiratory patterns with excessive dependence on blood CO2/pH levels to trigger brain stem respiratory centers.
Midbrain and upper pontine lesions may cause a central neurogenic hyperventilation syndrome with persistent deep hyperventilation. It can only be diagnosed with arterial blood gas measurements, since hyperventilation also occurs secondary to hypoxemia and acidemia. Likewise, metabolic disorders, especially the early stages of hepatic coma, cause central neurogenic hyperventilation.
Lesions of the middle or lower pons are characterized by deep prolonged inspiration followed by a long pause referred to as apneustic breathing. Most patients with this respiratory pattern require early intubation and mechanical ventilation.
Ataxic and irregular periodic breathing occurs with lesions in the dorsomedial medulla and may be accompanied by hypersensitivity to respiratory depressants. These patterns are not compatible with sustained life.
When assessing a comatose patient, the rate and pattern of respiration should be observed. In addition, vomiting and hiccups should be noted because they may result from intrinsic brain stem pathology or transmitted pressure on the brain stem. Furthermore, spontaneous yawning may occur in comatose patients. The neurogenic networks for this complex respiratory response are integrated in the lower brain stem.
Pupillary Size and Reactivity In one study of 346 comatose patients, the pupillary reflex was shown to be the strongest prognostic variable for awakening when compared with evoked-potential studies.20 Pupillary size is controlled by the autonomic nervous system and is dictated by the balance between sympathetic and parasympathetic input to the pupillary dilators and constrictors, respectively. The parasympathetic efferents to the pupil originate from the Edinger-Westphal nucleus in the upper midbrain and travel with the ipsilateral third cranial nerve (oculomotor). Dysfunction within this pathway will produce unopposed sympathetic input to the pupil and relative pupillary dilation ipsilateral to the lesion. The sympathetic efferents to the pupil originate in the hypothalamus, descend through the brain stem and cervical spinal cord, and exit the upper thoracic spinal cord (T1-T3 levels). From this point, they ascend the carotid sheath and follow the vasculature to the pupil. Any disruption of the sympathetic fibers along this loop can lead to unopposed parasympathetic pupillary activity and subsequently an ipsilateral small (miotic) pupil.
Pupillary reflex is examined using a light stimulus to one eye, which produces constriction of the ipsilateral pupil (direct response) and contralateral pupil (consensual response), through a network of connections. Table 88-6 summarizes the pupillary changes commonly seen in coma and their significance.
Small reactive pupils may be due to a toxic-metabolic disturbance. Very small pupils (pinpoint) that react to naloxone are characteristic of an opiate overdose. Pinpoint pupils that are poorly reactive are characteristic of pontine dysfunction. Lesions rostral or caudal to the midbrain may disrupt descending sympathetics and produce small pupils.
Bilateral, widely dilated pupils are due to sympathetic overactivity from an endogenous cause (seizures or severe anoxic ischemia) or exogenous catecholamines (dopamine or norepinephrine) or atropine-like drugs.
Since the midbrain is the one location in the brain stem where parasympathetic and sympathetic pupillary fibers are adjacent, a midbrain lesion classically results in intermediate pupil size. Such pupils are seen in severe midbrain injuries and herniation.

Full access? Get Clinical Tree
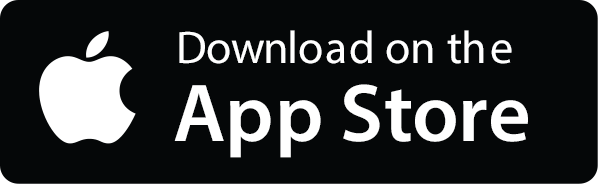
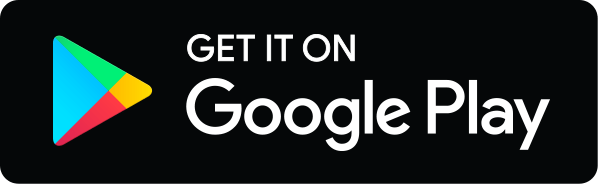