FIGURE 1 Typical visual analogue scale (VAS, 10 cm) scores of saline-induced pain in muscle, distal tendon, and proximal tendon-bone junction of the m. tibialis anterior, and in the joint (knee fat pad) and sacroiliac joint ligament. Hypertonic saline (5.8%, 0.5 – 1.0 ml) was injected in healthy subjects as bolus injections. Based on data from Gibson et al. [33], Palsson et al. [80], and Joergensen et al. [59].
Mechanical stimulation of the periosteum in humans induces an immediate sharp arresting pain with the lowest threshold as compared to the ligaments, the fascia, tendon, and muscle as the least sensitive [53]. Likewise, injecting hypertonic saline in the surface of the human periosteum elicits more pain than when injecting in muscle (Fig. 1) [39]. Recently, a novel human experimental model of bone pain was developed based on standardized mechanical impact stimulation [30].
Connective Tissue
Connective soft tissues are supplied with nociceptive afferent fibers and are probably an important source of nociceptive afferent activity during clinical pain conditions. Slowly conducting afferent fibers (group III and IV) and nerve endings, presumably nociceptive, have been observed in animal connective tissue, such as tendon and fascia [5,105]. Calcitonin-gene related peptide (CGRP) and substance P (SP) immunoreactivities have also been demonstrated in human tendon tissue [11,22] indicating a thin fiber sensory innervation most likely serving a nociceptive function. The nerve endings in tendon are mainly located in proximity to small arterioles and blood vessels in the tissue [11,22,84]. Histologically, small fiber free nerve endings have been demonstrated in human fascial tissue [109]. A quantitative evaluation of CGRP and SP-containing free nerve endings was performed in the thoracolumbar fascia illustrating that the fascia, especially the outer part, is densely innervated; in a pilot study, the human thoracolumbar fascia was further demonstrated to show similar properties [105] and may therefore be important in low back pain conditions. Recently, single fiber recordings from thin myelinated and un-myelinated afferent fibers located in fascia demonstrated nociceptive characteristics to mechanical, heat, and chemical modalities [104].
Human studies have utilized experimental pain induction techniques directed towards fascia, ligaments, and tendon tissues [34,35,53,60]. Interestingly, the experimental tendon and ligament pain models based on injection of hypertonic saline report higher pain intensity and longer pain duration compared with muscle pain models [33;53]. There is also a marked difference in the pain response following hypertonic saline injected into the deep part of the muscle and the fascia where fascia induced more pain compared with muscle belly injections [36]. This is clinically relevant as it implies that the contribution of connective tissues to the clinically prevalent entity ‘muscle pain’ needs to be carefully considered. Recently, a study compared the pain sensitivity to injections of hypertonic saline into connective tissue around the hip and reported that the gluteus medius tendon injection caused higher pain intensities than injections into the addutor longus tendon and gluteus medius muscle [55]. Similarly, Gibson et al. [33] reported higher pain intensity following hypertonic saline injected into the tendon-bone junction of the tibialis anterior muscle compared with its tendon and muscle belly site (Fig. 1). Injecting the tendon-bone junction probably stimulates the periosteal tissue which is known to be more sensitive than the muscle belly [53] thus contributing to the higher pain intensity at this site. Slater et al. [96] demonstrated a higher pain intensity following hypertonic saline injection into tendoachilles compared with the common extensor tendon. The different tendon pain profiles are likely explained by variation in nociceptor density and innervation patterns of individual tendons.
LOCALIZED HYPERSENSITIVITY
Localized Muscle Hypersensitivity
Sensitization of muscle nociceptors is normally evoked by overuse, trauma, or inflammation causing release of substances from glia cells, neural, and muscular tissues. Damaged deep-tissue release e.g. potassium ions, prostaglandin E2, bradykinin, serotonin, and the nerve ending release e.g. neuropeptides such as substance P (SP), calcitonin gene-related peptide (CGRP) or somatostatin [72]. Most of the sensitizing substances released in muscles are similar to those released in other tissues, but adenosine triphosphate (ATP) is present in muscle cells in high concentration and may be more specific for muscles. During muscle inflammation, the density of nerve endings containing SP and nerve growth factor (NGF) increases [84]. Moreover, the acid sensing ion channel 3 (ASIC3) has been shown important for the mechanical hyperalgesia in an animal inflammatory muscle pain model [99].
Short lasting muscle hyperalgesia in humans can be studied after intramuscular injections of capsaicin, glutamate, or combined intramuscular injections of serotonin (5-HT) and bradykinin. Ernberg et al. [27] found that intramuscular co-injection of 5-HT and the 5-HT(3) receptor antagonist granisetron reduced the spontaneous pain evoked by injection of 5-HT and prevented hypersensitivity to mechanical pressure stimuli. Later it was demonstrated that i.m. injection of granisetron in patients with localized myalgia increased the pressure pain thresholds [18]. It is also known that the NMDA receptor is involved in glutamate evoked muscle hypersensitivity since this can be reduced by co-administration of an NMDA-antagonist [14].
Tonic muscle hyperalgesia may be induced if a muscle is eccentrically exercised; it will become sore after 1–2 days. This model of delayed onset muscle soreness (DOMS) show hypersensitivity to pressure at different sites among subjects, and these localized spots of hyperalgesia might link to the initial mechanisms for hypersensitive trigger points in myofascial pain patients. The mechanism underlying delayed onset muscle soreness is not clear, but is probably related to ultrastructural overloading resulting in release of sensitizing substances such as bradykinin and nerve growth factor [73]. The hypersensitive component in DOMS has also been suggested to be mediated by the thick myelinated afferent fibers and not exclusively by the thin un-myelinated nociceptive afferent fibers [10].
A clinical example of muscle hyperalgesia is muscle sites located in taut bands of muscle fibers with a hard and hypersensitive locus; trigger points in myofascial pain patients [93]. Recently, a novel methodology has been developed to visualize the taut band related to trigger points in muscles [17]. Trigger point assessment may involve pressure algometry to record pain thresholds [16,107] which is a more standardized approach than the manual palpation. Nonetheless, the overlap of sites with reduced pressure pain thresholds and sites palpated with tender points in healthy subjects is poor [4].
Localized Joint Hypersensitivity
In line with muscle nociceptors, the joint nociceptors have been demonstrated to be sensitized in inflammatory and degenerative animal joint models [86]. One particular example is the silent nociceptors, which do not respond to noxious mechanical stimuli under normal condition, but are active in inflammatory conditions [88]. Sluka demonstrated that muscle and joint tissue in rats show different sensory responses to experimental nociception with prolonged allodynia in joint compared to muscle tissue [97]. It would appear reasonable to presume that human muscle and other deep tissue (e.g. tendon) have a similar difference in sensory manifestations to experimental pain stimulation. The animal joint sensitization models cannot be translated into human models, but there are human studies focusing on pain sensitization related to peri-joint structures. An example is experimentally induced pain in the fat pad of the knee by injections of hypertonic saline in asymptomatic subjects. In this model hyperalgesia to pressure is found close to the injection site [59].
Recently there has been focus on the peripatellar hypersensitivity detected in OA patients compared with healthy control subjects [7,43]. Peripheral sensitization of the nociceptors is likely by e.g. inflamed synovium and damaged subchondral bone. As a sensitive marker of low grade systemic inflammation, high-sensitive C-reactive protein has been shown to correlate with pain severity [103]. Interestingly, the most sensitive site around the OA knee was located on the medial side in the patients with the highest habitual pain intensity [7] in accord with the medial compartment structures being predominately involved in the osteoarthritic process [20]. The peripatellar hyperalgesia to pressure was mainly expressed in patients with severe knee pain indicating a relationship between the tenderness in the encapsulated tissue and the intra-articular lesion in patients with knee OA [7,52].
Localized Bone Hypersensitivity
In cancer bone pain, tumor cells and associated inflammatory cells include several inflammatory mediators which may sensitize the bone nociceptors [66]. In particular the NGF has been suggested as a major player in the sensitization of nociceptors close to the tumor. Therefore, in more recent years, there has been focus on the role of NGF for nerve sprouting and formation of micro neuromas in animal bone cancer models, and hence anti-NGF compounds may be an interesting target [12]. In addition to the sensitization process, tumor-induced injury to primary afferent nerve fibers may cause a neuropathic pain component [81]. It is expected that the nociceptors are sensitized by inflammatory mediators after bone damage such as fractures [13]. A similar process although less intensive is probably also reflected in a human experimental model where impact stimuli were repeatedly applied to the tibia bone, and after one day hyperalgesia to pressure and impact stimulations were detected [30].
Localized Hypersensitivity in Connective Tissue
Tendon tissue has been shown to express sensory afferent NMDA receptors [1], and increased tissue glutamate level is associated with tendinopathy [2] implying glutamate may be important in tendon sensitization and pain. Gibson et al. [34] characterized the pain responses from asymptomatic tendons and found that glutamate and capsaicin injections to the tendon caused pain and hyperalgesia. Moreover, experimental tendon and ligament pain models based on hypertonic saline injections also includes localized hyperalgesia [33,80,96].
The delayed onset muscle soreness developing after eccentric contractions has been suggested to include sensitization of nociceptors in various tissues including epimysium/fascia rather than muscle tissue proper. Indeed increased sensitivity of mechanoreceptors was found in animal fascial/epimysium tissue 2 days after eccentric contractions in rats [36]. In humans, hypertonic saline injected into fascia during DOMS resulted in higher pain intensities compared with pre-exercise suggesting the relevance of sensitized fascia in DOMS [36]. This is in line with increased sensitivity to electrical stimulation in fascial tissue shown in humans following eccentric exercise [54]. Connective tissue may be exposed to excessive stress causing sensitization during eccentric contraction when the myofibrillar contractile mechanism becomes inefficient. Another interesting demonstration of localized sensitization in fascia tissue is seen after fascial injection of NGF into the fascia of m. erector spinae [23].
SPREADING HYPERSENSITIVITY
As outline below, musculoskeletal nociceptors are potential generators for central hyperexcitability. However, the dorsal horn neurons are also strongly controlled by the descending inhibitory pathways, and potential impairments of the descending control will give reason to similar manifestations as central hyperexcitability; e.g. reduced excitation threshold of spinal cord neurons to musculoskeletal nociceptive input, increased receptive fields, and increased on-going discharges of neurons [70,85].
Muscle as the Source for Spreading Hypersensitivity
Animal experiments suggest that expansion of receptive fields may account for spreading hypersensitivity and is working through opening of “silent” (ineffective) synapses in the spinal cord by nociceptive input from muscle [45]. Neurokinin-1 (NK-1) and N-methyl-d-aspartate (NMDA) receptors are involved in such an expansion [48]. A few hours after induction of an experimental myositis a local aesthetic block of the muscle afferents can prevent the central hyperexcitability to develop. If the block is later (2 to 4 hours after induction of the myositis) there is no influence on the development of the central hyperexcitability [49]. The contralateral spread of sensitization following repeated intramuscular injections of low pH saline [98] is another demonstration of the central hyperexcitability. New data suggest that different substances used for exciting muscle nociceptors have different influences on the dorsal horn neurons. In contrast to hypertonic saline, which gives a clear excitation of dorsal horn neurons, one injection of NGF mainly results in subthreshold potentials in the dorsal horn neurons, but still leaves the central neurons sensitized [51]. Moreover, 2 intramuscular NGF injections separated by 5 days resulted in dorsal horn neurons having more receptive fields in muscle and fascia [47] which also illustrates the central hyperexcitability.
Long lasting muscle hypersensitivity can be induced in healthy subjects by i.m. injection of NGF [32]. Interestingly, an NGF injection into the tibialis anterior muscle causes no pain, but a localized hypersensitivity develops after a few hours, and after 1 day the hypersensitivity has spread to a large portion of the muscle presumably due to central mechanisms [3]. Daily intramuscular injections of NGF results in progressive increasing muscle soreness [44]. Therefore, the NGF model may be regarded as an interesting model for spreading hypersensitivity in muscle tissue. If the original NGF injection site is anaesthetized after one day, when the spreading hypersensitivity is present, there is no immediate effect on the spreading hypersensitivity [32]. This suggests that the spreading hypersensitivity is based on a robust central mechanism independent of the peripheral drive at least in the short time frame. Interestingly, capsaicin-induced sensitization of the skin dermatome (C5, arm) evoked reduced pressure pain threshold (hyperalgesia) in the infraspinatus (C5–6) muscle [101] illustrating the segmental interaction, which may be crucial for understanding comorbid symptoms.
Fibromyalgia or whiplash associated pain is often categorized as widespread pain or hyperalgesic conditions. The more widespread a musculoskeletal pain problem becomes, the more quantitative sensory abnormalities occur [16], which may be another finding indicating central hyperexcitability. A detailed trigger point examination in fibromyalgia patients showed an increased number of trigger points in patients compared with controls [31] suggesting that more trigger points may be a result of facilitated central mechanisms.
Joint as the Source for Spreading Hypersensitivity
Intense and prolonged nociceptive input from the joint in animals may result in central hyperexcitability manifested as e.g. expanded receptive fields [67,85]. Ongoing nociceptive activity from the joint capsule (stretching), the synovium (inflammation), and corroded subchondral bone [24] could be the peripheral generators leading to facilitated central pain mechanisms.
In the early stage of OA pain, this is recognized as a localized pain condition, but the persistent pain or hypersensitivity may result in a progression to more regional or even widespread symptoms. Hyperalgesia to pressure stimulation outside the symptomatic joint has been reported in patients with OA in either the carpo-metacarpal joints [28], knee [7], or hip [62]. Recently, widespread hyperalgesia was also demonstrated in knee OA patients with increased pain thresholds after treatment by total knee replacement [43]. Spreading hypersensitivity to the contralateral knee has also been observed [7] and is most likely due to the fact that some knee OA patients have bilateral symptoms, and in addition, contralateral subclinical changes may exist in the patients only presenting with one symptomatic knee. In chronic diseases such as knee OA it is also possible that the central neuronal systems are sensitized bilaterally. In such scenarios the clinical pain manifestations start to spread together with sensitization of central mechanisms, e.g. a joint pain patient begins complaining about pain in other regions, and the pain potentially develops into a widespread pain, and co-morbid pain conditions may develop. In low back pain (herniated disc) patients, a similar widespread hyperalgesia to pressure has been detected with reduced pressure pain threshold in the shin muscle [78]. The spreading hyperalgesia seems to depend on tonic pain for some time as 10 min of electrical stimulation on the lumbar facet joint (L3–4) did not evoke hyperalgesia outside the stimulation area [77].
The first use of cuff-algometry in patients with arthritis has demonstrated the feasibility of using cuff-algometry on the lower leg to measure hyperalgesia in knee OA patients [43]. Similar spreading hypersensitivity to those observed using a conventional pressure algometry on the tibialis anterior muscle was found with cuff pressure pain thresholds being significantly lower in OA patients compared with controls.
A higher frequency of latent myofascial trigger points was detected in the lower leg of OA patients compared with healthy controls [8], and such a co-morbid condition might be explained by a generalized reduced threshold for eliciting a painful perception due to central hyperexcitability. Interestingly, the habitual OA pain areas do not consistently include the latent myofascial trigger points. Since the aetiology of myofascial trigger points is not clear, peripheral factors contributing to the frequent development of trigger points cannot be excluded although it seems unlikely.
Bone as the Source for Spreading Hypersensitivity
Widespread hypersensitivity in non-malignant bone pain such as fractures or malignant bone pain has not been extensively described. In an animal model with tumor induced in the femur bone, it was demonstrated that non-noxious palpation of the tumor induced a nocifensive behavioral response which was not found in control animals and was correlated with the extent of bone destruction [91]. Similar responses were found after palpation of the knee which may illustrate the spreading hypersensitivity. In animal bone tumor models, it has been demonstrated that wide dynamic range dorsal horn neurons becomes hyperexcitable and the receptive field enlarges [106]. So it is likely that spreading hypersensitivity may occur due to the peripheral sensitization such as tumor growth and/or in combination with central hyperexcitability. Some bone cancer patients present with widespread bone metastases and little pain whereas others have minimal bone metastases and severe pain [79] indicating that the central mechanisms may be important.
In a recent experimental study, repeated mechanical stimulations induced hyperalgesia to pressure although this seems relatively localized [30]. In contrast, bone metastases may appear to include larger bone structures and as such larger areas with hypersensitivity is formed based on peripheral sensitization.
Connective Tissue as the Source for Spreading Hypersensitivity
Excitation of nociceptors from ligaments and fascia has demonstrated to drive hyperexcitability of dorsal horn neurons where more neurons are involved during hyperexcitability compared with control conditions [21;50;83]. Recent experimental pain induced in the human sacroiliac join ligament by hypertonic saline injections resulted in hyperalgesia distant from the injection site [80]. Likewise, capsaicin injection caused significant increases in mechanosensitivity at the tendon injection site and 2 cm proximally [34]. Clinical tendinopathy is associated with increased mechanical sensitivity in the affected tendons [63;95], but no studies have reported widespread hypersensitivity. If evoking experimental muscle pain in the symptomatic arm of lateral epicondylalgia patients, the pressure thresholds decreased in the contralateral asymptomatic arm indicating a facilitated central mechanism [95]. Using cuff algometry in lateral epicondylalgia patients compared with controls, hyperalgesia on the arm and lower leg was detected demonstrating spreading hypersensitivity [58].
TEMPORAL SUMMATION OF PAIN
Repeated somatosensory stimuli with the same intensity evoke a pain perception which is progressively increasing. This phenomenon is defined as temporal summation of pain and probably parallels the initial phase of the neuronal wind-up process reported in animals [6]. Short duration intervals between sequential stimuli at constant strength (e.g. 10 stimuli and 1 s inter-stimulus interval) are needed to evoke temporal summation of pain.
Temporal Summation of Muscle Pain
In clinical and experimental studies, electrical, chemical, pressure, and focused ultrasound repeated stimulation has been used to assess the temporal summation of muscle pain [40,100,108]. Computer-controlled sequential pressure stimulation on muscle evoked temporal summation which was more efficient compared with sequential stimulation on the skin only (Fig. 2) [74]. The temporal summation of muscle pain is facilitated in fibromyalgia and OA patients, and the involvement of central hyperexcitability has been proposed as an explanation for the findings [7,100,102]. Recently, cuff algometry has been used to assess temporal summation of cuff-induced pain from the calf muscles in knee OA patients with and without clinical pain following revision total knee replacement; the temporal summation of cuff pain was significantly facilitated in the group of patients with pain [94]. Facilitated temporal summation of continuous cuff evoked pain has also been reported in lateral epicondylalgia [58].
In healthy subjects, experimental muscle soreness has also been reported to facilitate the temporal summation of pressure induced muscle pain [75] indicating that the time window with the nociceptive activity needed to cause the central hyperexcitability is relatively short. Facilitated temporal summation of the nociceptive withdrawal reflex elicited by repeated cutaneous electrical stimulation has also been used as an indicator for central hyperexcitability in fibromyalgia patients and whiplash pain patients [9]. In some patients, a minor nociceptive activity is plausible without confirmed tissue damage and facilitated temporal summation is likely to explain the perceived pain in such cases.
Temporal Summation of Joint Pain
In a recent experimental study Joergensen et al. [59] demonstrated that temporal summation elicited by sequential pressure stimuli at the fat pad of the knee and knee-related muscles was facilitated during experimental fat pad pain. Temporal summation assessed by pressure stimulation on the knee was significantly facilitated in knee OA patients compared with controls [7]. Interestingly, patients with high intensity habitual knee pain showed more temporal summation of muscle pain than OA patients less affected. The duration of OA pain was correlated to the degree of temporal summation of pain [7] suggesting that the involved plasticity is a progressive process. An intriguing finding was facilitated temporal summation assessed contralaterally to the most affected knee [7] which may be due to the fact, however, that most knee OA patients have OA symptoms in the contralateral knee although less affected. An alternative or additional explanatory component is that the same neurons sensitized by the most affected knee contribute to enhanced temporal summation on the contralateral side because some neurons have bilateral receptive fields [87].
FIGURE 2 Temporal summation following repeated pressure stimuli to muscle, tibia bone, and skin (web-space). Ten pressure stimuli were delivered with 2 sec interval by the computer-controlled pressure algometer (right). The subject scored the pain intensity on a visual analogue scale (VAS), and the ratio of increase with respect to the first stimulus is illustrated. Modified from data in Nie et al. [74].

Full access? Get Clinical Tree
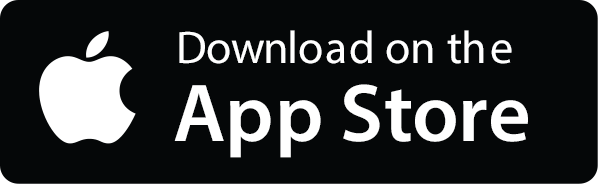
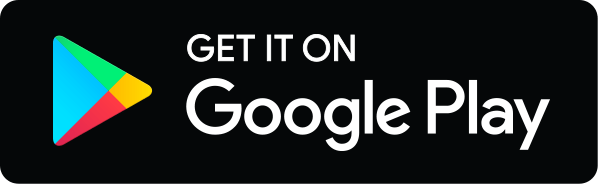