CHAPTER 21. Neurologic Emergencies
Katherine Logee
Competencies


1. Describe neurologic pathophysiology in terms of pressure-volume relationships and the components of the cerebral vault.
2. Perform a focused neurologic examination.
3. Describe common neurologic emergencies encountered in the transport environment in terms of pathology and management strategies.
Neurologic illness or injury is a common reason for air medical transport because of its potentially disabling or fatal consequences. The transport team is instrumental in assessing and determining the need for intervention on the basis of expert knowledge of the physiology of neurologic pathologies and how they relate to air medical transport. The two priorities of the air medical crew are effective management of those secondary injuries and rapid transport to an acute care facility.
NEUROLOGIC PATHOPHYSIOLOGY
A basic understanding of neuropathophysiology assists with the ability to critically think through management of patients with neurologic insults. We examine pressure-volume relationships and the volumetric components (cerebrospinal fluid [CSF], blood, and brain) of the neurologic system to gain an understanding of how changes in each of these components affect intracranial pressure (ICP), maintenance of ICP, alterations in ICP, and management of ICP changes.
Pressure-Volume Relationships
The main components of volume in the skull are the brain (1400 mL), blood (1400 mL), and CSF (150 mL). The latter two drain into the dural sinuses. In healthy individuals, venous pressure is the main determinant of ICP. However, any increase in the volume of one of these components without a corresponding decrease in the volume of the other two results in raised ICP. 20,27,33,39,45 Alteration in ICP is the prime concern in the care of patients with neurologic emergencies. 18,24,27,34,36
How the volumes of CSF, blood, and brain maintain a relatively constant ICP is described by the Monroe-Kellie hypothesis. The Monroe-Kellie hypothesis states that the cranial compartment is incompressible and that the volume inside the cranium is a fixed volume. The cranium and its constituents (blood, CSF, and brain tissue) create a state of volume equilibrium such that any increase in volume of one of the cranial constituents must be compensated by a decrease in volume of another (Figure 21-1).
![]() |
FIGURE 21-1 Modified Monroe-Kellie hypothesis. |
Because brain volume changes little (and when it does, it involves mainly interstitial water), most normal variations occur in the volumes of CSF and blood. 20,27,33,39
The two principle factors that affect cerebral blood flow are the cerebral perfusion pressure (CPP) and the brain’s autoregulatory system. Cerebral perfusion pressure is equal to the mean arterial pressure (MAP) minus the intercerebral pressure (ICP) or the central venous pressure, whichever is higher.


The native autoregulatory system refers to the brain’s ability to keep the cerebral blood flow at a relatively constant level over a wide range of cerebral perfusion pressure, which is accomplished by varying the resistance in the precapillary arterioles. 36 A CPP greater than or equal to 70 mm Hg ensures adequate cerebral perfusion; ICP is normally 5 to 20 cm H 2O or 3 to 15 mm Hg. Constant cerebral blood flow is obtained with MAPs from 50 to 150 mm Hg, but these values may be higher in patients with chronic hypertension. At the low end of this curve (see Figure 21-2 and 21-3), CPP drops and cerebral hypoperfusion ensues; however, at the high end of the curve, cerebral hyperperfusion with cerebral edema occurs. In disease states, the curve becomes linear and the CPP approximates the CBF because of the loss of vessel autoregulation. Elevation of ICP or decrease in MAP results in cerebral hypoperfusion. 40,45
![]() |
FIGURE 21-2 Pressure-volume curve. |
![]() |
FIGURE 21-3 Changes in cerebral blood flow (CBF) caused by independent alterations in PaCO 2, PaO 2, and mean arterial pressure (MAP). (From Piyush M, Patel PM, Drummond JC: Cerebral physiology and the effects of anesthetics and techniques. In Miller RD: Miller’s anesthesia, ed 6, Philadelphia, 2005, Churchill Livingstone.) |
To understand the ability of the intracranial components to compensate for the development of a mass (thinking of the combined volume of cerebral edema as mass is helpful), a look at the pressure-volume curve is useful (Figure 21-2). 20,27,39,41 As a change in volume of mass occurs, at first, no change in ICP occurs. This phenomenon is the result of compliance and is accomplished by reduction in volume of CSF and blood. At some point, however, compliance is lost, and additional changes in volume result in great increases in ICP. In the acute state of cerebral edema, or a rapidly developing intracranial, the total shifts in volume amount to approximately 50 to 75 mL. 20,32,39,43,44,46
Once the sutures and fontanelles close during childhood (by 18 months of age), the skull becomes a nondistensible structure. When a space-occupying lesion develops (whether that is a buildup of CSF, blood, or even a tumor), a reduction in the volumes of one or more compartments of the brain must occur for ICP to stay constant. The viscoelastic properties of the brain are such that if a mass, such as a growing tumor, exerts slowly increasing pressure, the brain may compensate through the slow loss of water or cellular elements through atrophy. However, with acute changes in the size of a mass, such as in an acute epidural hematoma, the brain is relatively noncompressible.
Cerebrospinal Fluid Volume
The volume of CSF is controlled by the rate of production and the rate of absorption of CSF. Production, except in the presence of very high ICP, remains relatively constant in the adult at about 0.35 mL/min. CSF is actively secreted by the choroid plexus in the lateral, third, and fourth ventricles. CSF leaves the ventricular system, circulates throughout the subarachnoid space, and finally reaches the subarachnoid space overlying the cerebral convexities. Here, the CSF is absorbed passively by way of the arachnoid granulations located parasagittally along the sagittal sinus. The fluid passes through the structures of the granulation into the cerebral venous system and is carried away with venous blood. 26,34
Absorption is pressure driven. The rate of CSF absorption is proportional to the ICP. CSF volume can be pathologically increased by an interference with absorption. 12,30,38 This increase can be caused by a mass or stricture in the ventricular system that prevents the CSF from exiting into the subarachnoid space. This accumulation of CSF is termed obstructive or noncommunicating hydrocephalus. 12,36
Alternatively, after the CSF leaves the ventricular system, its circulation may be disturbed so that the fluid cannot reach the arachnoid granulation, resulting in communicating or nonobstructive hydrocephalus. Given that the subarachnoid space is so large, a focal lesion such as a tumor ordinarily does not produce this type of obstruction. Instead, a widespread disorder such as inflammation of the meninges or increased CSF protein results from such an obstruction. 35
Cerebral Blood Volume
Cerebral blood volume comprises two relatively independent components: the arterial blood volume and the venous blood volume. Arterial blood volume accounts for approximately 25% of the total cerebral blood volume; venous blood volume accounts for the other 75%. 27,28,34
Arterial cerebral blood flow (and volume) under normal circumstances remains relatively independent of systemic mean arterial blood volume and pressure through a process called autoregulation. Autoregulation is influenced by pressure and biochemical parameters. 2,3,23,27 As mean systemic arterial pressure increases, cerebral arterial blood vessels constrict, preventing the increase in blood volume and flow that normally occurs. If the mean systemic arterial blood pressure decreases, the cerebral arteries dilate, increasing cerebral blood flow. Alternatively stated, the blood flow is directly proportional to the perfusion pressure and inversely proportional to the total resistance of the system. Cerebral blood flow is maintained in a constant state between a mean systemic arterial pressure of approximately 60 and 140 mm Hg. Normal blood flow to the cerebral cortex averages 50 mL of blood flow/100 g of brain tissue (expressed as mL/100 g/min). 33 At perfusion less than 20 mL/100 g/min, neuronal cell membranes become impaired, which results in neurologic dysfunction. Despite this impairment, if the blood flow is restored, this damage is reversible. At perfusion flow less than 10 mL/100 g/min, the neuronal tissue rapidly becomes irreversibly damaged. In a no-flow state, cell death occurs within minutes. 33
Arterial blood volume is also influenced by complex biochemical or metabolic action that can be summarized by the association of PaO 2, PaCO 2, and cerebral blood flow.
Increased PaCO 2 or decreased PaO 2 results in dilation of the blood vessel, presumably in response to greater cerebral metabolic needs. 27,29,46 As PaCO 2 decreases, blood volume and flow are reduced. Thus, this component of autoregulation may be influenced by respiratory control, with hyperventilation resulting in decreased cerebral blood flow and hypoventilation resulting in increased cerebral blood flow. 1,2,27 Venous blood volume is passively influenced by the delivery of blood from the arterial side and the ability of the cerebral venous system to drain from the head. This drainage depends on two influences: hydrostatic pressure and central venous pressure. Elevation of the head increases hydrostatic pressure on the venous side, permitting more rapid drainage from the cerebral venous system, mainly through the internal jugular veins bilaterally. Increased central venous pressure, whether caused by increased intrathoracic pressure or by right heart failure, decreases cerebral venous return. 2,13,27
Respiratory management can greatly influence the volume of cerebral venous blood. If intrathoracic pressure is increasing because, for example, the patient is straining on an endotracheal tube that is blocked, a decrease in cerebral venous drainage (and thus an increase in cerebral venous blood volume) occurs. 27
Brain Volume
Brain volume, except for a relatively insignificant alteration in interstitial water, does not change under ordinary circumstances. However, with injury, cerebral brain water may accumulate in the form of cerebral edema. An accumulation of water can be found in the intracellular space, extracellular space, or both. The three types of cerebral edema are: vasogenic, cytotoxic, and interstitial edema.
Vasogenic edema is an extracellular edema of the white matter that results from increased capillary permeability as a result of the widening of tight junctions and increases in pinocytotic vesicles at the level blood-brain barrier. 21 Vasogenic edema is seen locally around brain tumors, although it can develop around a cerebral infarct or a cerebral abscess as well. Generalized vasogenic edema occurs with cerebral trauma or meningitis. 16,21
Cytotoxic edema is an increase in fluid in the neurons, glia, and endothelial cells as a result of ATP-dependent sodium-potassium pump failure so that fluid and sodium accumulate within the cell, leading to diffuse brain swelling. Development of cytotoxic edema is associated with a hypoxic or anoxic episode, such as a cardiac arrest or asphyxiation. It is also seen with hypoosmolarity conditions, such as water intoxication, hyponatremia, and the syndrome of inappropriate secretion of antidiuretic hormone. 21
Interstitial edema occurs with hydrocephalus. The edema is found in the periventricular white matter when the intraventricular pressure is greater than the ability of the ependymal cells to contain the CSF within the ventricle. 21
NEUROLOGIC EXAMINATION
A focused comprehensive neurologic assessment of the patient to be transported is important. After completion of a primary assessment, chief symptoms, history of present illness (including onset, duration, and sequence of symptoms), and medical history should be obtained. Box 21-1 contains a useful mnemonic for the differential diagnosis of an unconscious patient. Each of these items should be considered when identifying the etiology of an unconscious state. The examination itself should be conducted in a systematic, hierarchic, stepwise approach that proceeds from the highest level of function (cerebral cortex) to the lowest level of function (reflexes). The cranial nerves, motor system, and reflexes should be assessed as well. In many cases, serial focused neurologic examinations are necessary for appropriate and timely interventions.
BOX 21-1
Mnemonic for the Differential Diagnosis of Coma
U Units of insulin
N Narcotics
C Convulsions
O Oxygen
N Nonorganic
S Stroke
C Cocktail
I ICP
O Organism
U Urea
S Shock
The focused neurologic assessment begins with obtaining vital signs and performing a general inspection of the patient. A rapid assessment of the patient’s mental status should be made. Is the patient awake? Can the patient talk? Can the patient answer questions appropriately? Can the patient protect the airway? A complete mental status examination focuses on the following areas: awareness and mental function; and reception and interpretation of sensory stimuli, including an awareness and responsiveness to self, to the environment, and to the impressions made by the senses, and of cognitive function. 21
There are 12 pairs of cranial nerves (a pair consists of a right and left nerve). Each nerve (right and left) must be evaluated separately to completely identify the extent of dysfunction that may be unilateral. Findings are compared for symmetry. As a brief review, cranial nerve (CN) I is the olfactory nerve. Testing of this nerve is often times deferred, unless an anterior fossa mass is suspected. CN II is the only nerve that can be examined directly. Testing encompasses an evaluation of visual acuity, visual fields, and an ophthalmoscopic examination. An ophthalmoscopic examination is rarely completed in the transport environment because of the need to balance a comprehensive examination with a rapid transport and relatively short bedside time. CNs III, IV, and VI are all tested together because all three supply the extraocular eye muscles. CN V is composed of both sensory and motor components. Sensation on the face is tested here as is masseter and temporal muscle strength (clench/open jaw). CN VII is the facial nerve. This nerve also has both sensory and motor components. The sensory component includes the sense of taste on the anterior two thirds of the tongue. The motor component is tested by observing symmetry of the face at rest and during deliberate facial movements. CN VIII, also called the acoustic nerve, is a pure sensory nerve. Testing of this nerve involves the use of a tuning fork to test air and bone conduction. CNs IV and X are tested together because of their intimate association of function in the pharynx. Having the patient open the mouth and say “ah” allows visualization of the uvula for midline location and voice quality analysis and also allows for the testing for the presence of a gag reflex. CN XI is the spinal accessory nerve and is tested by having the patient shrug the shoulders against resistance and by having the patient turn the head to the side against resistance. Finally, CN XII is the hypoglossal nerve. Testing this nerve involves having the patient protrude the tongue and move it tongue from side to side. Clinical judgment must be used when deciding whether or not a judicious use of time involves performing a complete or partial cranial nerve assessment. This decision is based on the type of patient being transported and the agency’s standing medical orders.
The next part of the neurologic examination is the motor system examination. The neck, upper limbs, trunk, and finally, the lower extremities should be examined (in that order). Generally speaking, muscle size, tone, and strength are examined.
The sensory system is examined next and is done with the patient’s eyes closed. This portion of the examination is not generally included in a standard neurologic examination unless numbness, pain, trophic changes, or other sensory abnormalities are present. 21 Superficial, deep, and discriminative sensations are tested. 37
The cerebellar system is tested by beginning with a finger-to-nose test. If this test is completed successfully, a further examination of upper extremity coordination is not necessary. Lower extremities are tested by having the patient draw a figure 8 on the opposite leg. Balance is also tested as part of the cerebellar assessment. This test is rarely indicated (or even advised) in the transport environment.
< div class='tao-gold-member'>
Only gold members can continue reading. Log In or Register a > to continue

Full access? Get Clinical Tree
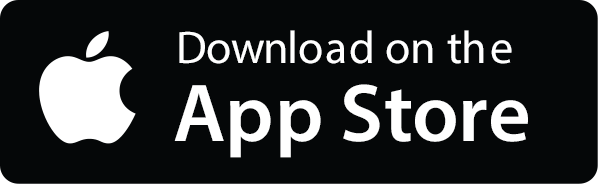
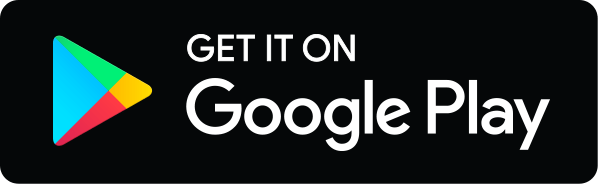
