Chapter 96 Wilderness Navigation Techniques and Communication Methods
For online-only figures, please go to www.expertconsult.com
Navigation With the Global Positioning System
The Navstar GPS uses satellites as predictable extraterrestrial references for the determination of terrestrial position. Calculation of position is based on circles of equal distance from the satellites. The current GPS consists of a constellation of 24 satellites arrayed in 6 orbital planes, with 4 satellites per orbital plane. The orbital planes are inclined to the earth’s equator by 55 degrees. The orbital paths of the satellites are nearly circular and have an altitude of approximately 20,000 km (12,425 miles; medium Earth orbit) with an orbital period of 11 hours and 58 minutes. At any given time, five to eight satellites are available in line of sight to a GPS receiver anywhere on the surface of the earth.13,27
The method of position determination using GPS depends on calculation of the range between the satellite and receiver. GPS signals are transmitted on two frequencies by each satellite: L1 (1575.4 MHz) and L2 (1227.6 MHz).11 Transmitted information includes the precise time as kept onboard the satellite by multiple atomic clocks, a satellite ephemeris (i.e., a catalog of predicted positions), and data concerning corrections for atmospheric propagation of radio signals and satellite clock errors. The GPS receiver decodes the positional data for each satellite and compares the timing information transmitted by the satellite with time as kept by the receiver’s onboard clock. Because distance = speed × time, the transit time of the signal allows calculation of the distance between receiver and satellite.24 At any given instant, a GPS receiver in contact with a Navstar satellite will lie on the surface of a sphere of equal distance from the satellite. The intersection of this sphere of equal distance with the surface of the earth forms a circle of equal distance. The intersection of two such circles occurs at only two points, and the intersection of three such circles occurs at a single point on the earth’s surface (Figure 96-1);13,27,28,45 this is the position of the receiver. If the intersection of the sphere of equal distance of a fourth satellite is added, the approximate altitude of the receiver can be determined. Software allows the GPS receiver to choose the optimal group of four satellites for position determination among the subset of satellites within the line of sight of the receiver.
As originally configured, two levels of service were provided by the Navstar system; the Standard Positioning Service and the Precise Positioning Service. The Standard Positioning Service provided civilian users with positional accuracy to 100 m (328 feet) 95% to 98% of the time, to 50 m (164 feet) 65% of the time, and to 40 m (131 feet) 50% of the time. The intentional inaccuracy of the Standard Positioning Service system resulted from the introduction of timing errors into the broadcast signal from the satellites on frequency L1; this degradation of precise data from the satellites is called selective availability, and is controllable from the ground. Selective availability was formally implemented 1 year after public availability of GPS was granted in 1994, and was discontinued in 1999. There are no plans for re-implementation, and the Precise Positioning Service is the level of service available to all military and civilian users at the time of this writing. The Precise Positioning Service provides positional accuracy of 15 m, velocity accuracy of 0.1 m/sec, and time accuracy of 100 nanoseconds. To comply with the requirements of safety-of-life aviation applications, the Wide Area Augmentation System (WAAS) was developed in cooperation with the Federal Aviation Administration and Department of Transportation. WAAS consists of 25 North American ground stations that monitor and correct errors in the GPS satellite signals caused by ionospheric interference, orbital drift, and clock errors. Corrections are transmitted on the L1 frequency by geostationary satellites, and can be received by enabled receivers. WAAS was implemented for general application in 2000 and for aviation safety-of-life applications in 2003. WAAS-enabled civilian GPS receivers allow less than 3-m (10-foot) horizontal and 6-m (20-foot) vertical positional accuracy 95% of the time, with 7-m (23-foot) accuracy the remainder of the time.16 Currently, WAAS is available only in the continental United States, including Alaska, border areas of Canada and Mexico, and the surrounding coastal waters.
A Soviet-era system called the Global Navigation Satellite System (GLONASS) also exists. In the higher northern and southern latitudes, GLONASS has marginally better satellite coverage than does GPS, and its positional accuracy is 30 m (98 feet). GLONASS receivers are not widely available, however, and the system offers no compelling advantages over GPS for the surface navigator. The European Union and European Space Agency are in the testing phase of implementation of a global satellite navigation system called Galileo. The plan is for the Galileo system to involve 30 satellites in medium Earth orbit, occupying three orbital planes with an orbital inclination of 56 degrees. Galileo is intended to become operational for general use by 2014 and will be publically available and interoperable with GLONASS and GPS. Galileo will offer 1-m (3-foot) accuracy in position and altitude for latitudes of 75 degrees north to 75 degrees south.14,15
The raw output of a GPS receiver is in latitude and longitude to the nearest second of arc or in Universal Transverse Mercator (UTM) grid coordinates to the nearest meter. This information is somewhat abstract in isolation, but software included with the receiver permits the user to understand absolute and relative position and perform sophisticated navigational feats in the absence of other navigational aids. Even without a map or compass, GPS allows the user to determine his or her current position, direction, course traveled, deviation from an intended course of travel, bearing and linear distance to predetermined targets, and velocity. Hikers with GPS devices can literally have no idea about where they are, but if they have the coordinates of where they want to go, they can find their way to safety with remarkable fidelity. Even the simplest commercially available GPS receivers render it almost impossible to become lost over distances likely to be encountered on a hike. These receivers dispense with complex displays and have only two buttons. At the start of a hike, the user turns the unit on, allows it a brief period of time to acquire a fix, then pushes a button to record the coordinates of the initial position as a waypoint. As the hike progresses, the receiver continually determines and displays the distance and direction to the waypoint, and provides an arrow that points to the waypoint (Figure 96-2, online). As long as the user follows the displayed arrow on the return hike, he or she will move toward the starting location. This type of receiver will not allow the traveler to predict or avoid impassable obstacles that may lie on the straight-line course between the current position and the goal. However, most general-purpose GPS receivers have a “moving map” feature that solves this issue, as described later in this chapter. All receivers can calculate the heading of travel and thus provide the user with a method of determining cardinal directions by walking a brief straight course.
Waypoint navigation is a feature of particular utility for wilderness travel. Waypoints can be preloaded into the GPS receiver via the keypad or downloaded from a personal computer, or they can be added on the fly with a few keystrokes as locations of interest are encountered during the hike. Most receivers have memory capacity sufficient for storage of several hundred waypoints. A group of sequential waypoints can be stored as a route, and the actual path followed between waypoints on the outbound leg of a route can be stored as tracks. Tracks are the virtual equivalent of the user’s footprints, and are displayed on the GPS screen as a string of points or a continuous meandering line. On the return leg of a route, the receiver can display the path defined by the tracks of the user, the bearing and distance to any selected waypoint, and the current course (Figure 96-3). Many receivers inform the user that a waypoint is near by sounding a proximity alarm and then automatically switch to the next waypoint in the route. Theoretically these capabilities allow a hiker to follow a route in conditions of near-zero visibility when using no references other than the display of the GPS receiver. Identification of a large number of waypoints connected by tracks on a complex route essentially allows the user to follow a “breadcrumb trail” to return to his or her objective44 (see Figure 96-3).
GPS reaches its greatest usefulness when used in conjunction with a map. Many inexpensive GPS units include low-resolution base maps with a resolution of a few hundred meters. More capable receivers often include or permit downloading of high-resolution topographic maps. Receivers with mapping capabilities display the user’s current position, with any stored waypoints, routes, or tracks superimposed on the map image (see Figure 96-3). When a topographic map is available—whether virtual or on paper—GPS allows the user to plot his or her position and route at will, determine bearings to landmarks even when the landmarks are not visible, enter the location of terrain features for use as predetermined waypoints, and precalculate the distances to be traveled during a trek. The fullest use of GPS with a map requires an understanding of two commonly used terrestrial coordinate systems: the geodetic coordinate system and the UTM coordinate system.
The Geodetic Coordinate System
The angular measurement between the plane of the equator, as measured north or south from the center of the earth to a point on the surface, is the latitude (L) of that point (Figure 96-4). All points at the same latitude form what is called a parallel of latitude. Latitude is measured in degrees, minutes, and seconds of arc from 0 degrees through 90 degrees north or south. As such, the latitude of the equator is 0 degrees, whereas that of each pole is 90 degrees north or south. Every point on the surface of the earth is defined by a specific longitude and latitude.
Latitude and longitude appear along the margins of topographic maps distributed by the United States Geological Survey (USGS) and by other national governmental organizations responsible for cartography. At each map corner, the latitude and longitude of the point defined by the intersection of the horizontal and vertical map margins is recorded in degrees, minutes, and seconds (Figure 96-5, online). On standard USGS 7.5- and 15-minute maps, latitude and longitude notations appear on the margins at intervals of 2.5 minutes of arc, and are marked with black tick marks on the inside edges of each margin.
The Universal Transverse Mercator Coordinate System
The UTM and Military Grid Reference System grids are metric, make use of the transverse Mercator map projection in common with maps distributed by the USGS, and require no conversion between distances expressed as angles and linear measures of distance expressed in meters (m) or kilometers (km). UTM divides the earth’s surface from 80 degrees south latitude to 84 degrees north latitude into 60 south- to north-running zones arrayed around the surface of the planet like narrow slices of an orange. Each zone is 6 degrees wide in longitude. The zones are consecutively numbered from 1 through 60 beginning at an index line, the International Date Line, and progressing eastward. Each zone is subdivided from south to north in 8-degree increments of latitude (except the northernmost zone, which encompasses 12 degrees of latitude [i.e., 72 degrees north to 84 degrees north]). The south-to-north divisions of each zone are labeled consecutively and alphabetically from C through X, excluding the letters I and O to avoid confusion with the numbers 1 and 0 (Figure 96-6, online). In UTM, terrestrial coordinates are expressed in meters east of a false origin and north of a latitude index line. The false origin for any zone is an arbitrarily assigned south–north line 500,000 m (1,640,420 feet) to the west of the central meridian of the UTM zone of interest. Progress to the east of the false origin is termed false easting or simply easting. In the northern hemisphere, the latitude index is the equator. In the southern hemisphere, the latitude index is the southern limit of strip C (i.e., 80 degrees south latitude). Progress to the north of the latitude index is termed northing.27,38,60
UTM coordinates are printed on all USGS maps produced during the past several decades. These appear as numbered blue tick marks occurring at 1-km intervals along the horizontal and vertical map margins. The numbers express UTM eastings and northings and thus increase from left to right and from bottom to top (i.e., to the right and up). By convention, the central meridian of each of the 60 UTM zones is assigned an easting value of 500000 m E (500,000 m east of the false origin of the zone). Easting values less than 500,000 thus lie west of the central meridian for the applicable UTM zone, and easting values of more than 500,000 lie east of the central meridian for the UTM zone in question. Slight overlap (i.e., 80 km) between adjacent zones prevents negative easting values from occurring. Similarly, easting values of more than 1,000,000 are never encountered; they would lie beyond the overlap into the next zone to the east. For the northern hemisphere, northing values are expressed as meters north of the equator, and range from zero to 10000000 m N (10,000,000 m north of the equator) (Figure 96-7). Negative values for northing are avoided for the southern hemisphere by labeling the equator as 10000000 m N and counting upward toward this number as one moves northward from the southern latitude index (i.e., 80 degrees south latitude).

(Redrawn from Cole WP: Using the UTM grid system to record historic sites. http://www.cr.nps.gov/nr/publications/bulletins/nrb28/.)
On the horizontal margins of the map in the left upper and right lower corners, one of the last blue tick marks is labeled with a six-digit number in mixed large and small numerals (i.e., 716000 m E in Figure 96-5, online). This refers to a line that is 716,000 m east of the false origin or 216,000 m east of the central meridian of the applicable UTM zone, because the false easting value is more than 500,000 (the central meridian in this example is in UTM Zone 16 at 87 degrees west longitude). Similarly, one of the blue tick marks at the right lower or left upper vertical margins of the map image is labeled with a multidigit number (i.e., 4374000 m N for the left upper border), indicating a line 4,374,000 m north of the equator in UTM row S. Note that, within the six-digit false easting value and seven-digit northing value, the numerals in larger type represent thousands of meters or kilometers. UTM tick marks on a map margin between corners are labeled with three- or four-digit numbers. The last two digits, which are in large numerals, represent whole kilometers in relation to the UTM reference value given near the corner of the map. Thus, for practical purposes, when navigating within an area of several dozens to several hundreds of square kilometers, the UTM margin tick marks on any large-scale USGS map can be thought of as a simple kilometer scale. The long and intimidating numeric labels on the tick marks can be ignored (except to recognize that they indicate 1-km increments on the map margin).
Every point on the earth’s surface can be described by a unique false easting and northing value; this is described as a grid reference. Use of the UTM grid system for plotting position based on a map is actually much simpler than its conceptual framework would suggest. Position plotting is greatly assisted by using a specialized ruler called a roamer scale that is compatible with common USGS map scales (see Figure 96-5, online). Roamer scales are included on many baseplate compasses, can be easily made from a scrap of paper, or can be printed or purchased from Internet sources. The large graduations on a roamer scale generally denote 100-m increments, whereas the smaller divisions may represent a 10- or 20-m span. The steps for determining UTM coordinates for any point on a compatible map are:
This indicates that the location is in UTM Grid Zone 16S (Zone 16, Row S). The meridians bounding Zone 16 are 90 degrees west longitude on the west and 84 degrees west longitude on the east. Row S is bounded by 32 degrees north latitude to the south and 40 degrees north latitude to the north. The latitude and longitude boundaries of the identified zone and row include southwestern Ohio. The numbers specifying the unique location within the zone and row must be read, per UTM convention, to the right (easting) and then up (northing). The first seven-digit number indicates that the site of interest has a false easting value of 716469 m E (i.e., 716,469 m east of the central meridian for the zone) or 469 m east of the 16-km tick mark on the horizontal margin of the relevant USGS map (see Figure 96-5, online). The second number indicates that the location has a northing value of 4346677 m N (i.e., 4,346,677 m north of the equator) or 677 m north of the 46-km tick mark on the vertical map margin.
For navigation in the majority of wilderness activities, the area of interest fits within a single USGS 7.5-minute square, and accuracy to within 100 m is adequate. As such, the UTM coordinates used to describe a location can be abbreviated for simplicity, including only the whole kilometers (i.e., the larger two-digit numerals) and the small digit to their immediate right (representing hundreds of meters). Do not round the last digit upward. In the prior example, the location of interest would be described in abbreviated UTM notation as easting 164, northing 466 (i.e., about 400 m east of the 16 tick mark and about 600 m north of the 46 tick mark).8 Map usage involving the UTM coordinate system is greatly facilitated by drawing a 1-km by 1-km grid on the map using the UTM tick marks to define the whole-kilometer spacing of the grid lines.
Compass Navigation
The directional properties of lodestone (magnetite) were recognized by a variety of civilizations during ancient times. References to the use of a directional magnetized needle at sea appear in Chinese literature dating from the 12th century CE. Descriptions of the magnetic compass in European writings followed during the 13th century, by which time it was noted that a needle stroked on lodestone pointed to the vicinity of the North Star.6,7,12 Discovery of the magnetic compass was a seminal event in the exploration of the planet; the compass allowed reasonably accurate steering in all weather and provided the directional reference that permitted development of the process of dead reckoning.
Magnetic Dip, Deviation, and Declination
The directional properties of the compass result from interactions between magnetized iron in the compass needle and magnetic lines of force generated by metals in the earth’s core. These lines of force have both a vertical and horizontal component. The vertical component is termed magnetic inclination or dip. Dip causes a compass needle to incline downward from horizontal, potentially to a degree that interferes with the ability of the needle or card to pivot freely. Dip is 90 degrees at the magnetic poles and 0 degrees at the magnetic equator. Most modern compasses are manufactured to compensate for the average dip that is likely to be encountered in the region of intended use. Others allow a small weight to be moved along the indicator needle to compensate for dip in any region of use.55
Direction in compass navigation is expressed in three ways: (1) true direction or direction measured in reference to the earth’s meridians and geographic poles; (2) magnetic direction or direction measured in reference to the earth’s magnetic poles; and (3) compass direction or direction measured by the magnetic compass. Magnetic direction varies from true direction by the sum of declination and deviation. Compass direction varies from magnetic direction by the quantity of deviation.10,43 The definitions of magnetic and compass direction point out the necessity of minimizing preventable sources of compass deviation when taking bearings. For practical purposes, when preventable sources of deviation are minimized and the compass is used with caution, magnetic direction and compass direction can be considered to be equivalent.
Wandering lines of points with equal magnetic declination can be graphed on maps and charts; these are called isogonic lines. Lines representing points on the surface of the earth where the magnetic declination is zero, and where magnetic north and true north are aligned, are termed agonic lines. In the Americas, an agonic line follows a relatively straight and slanting course extending from the east coast of Victoria Island in north-central Canada through western Lake Superior, along the west coast of Florida, and traversing South America from the Gulf of Venezuela to the southeastern coast of Brazil. At locations east of the agonic line, the compass needle declines to the west (counterclockwise) of true north; at points west of the agonic line, the compass needle declines to the east (clockwise) of true north. By convention, magnetic declination is given a positive sign when east and a negative sign when west (Figure 96-8). Declination is quantified as the angle between true and magnetic north.
The mnemonic “Declination east, compass bearing least; declination west, compass bearing greatest” may be helpful for converting magnetic direction to true direction when taking a bearing. In other words, to convert from magnetic to true while taking a bearing from the compass, add east declination to the compass bearing, or subtract west declination from the compass bearing. When taking a true bearing from a map and converting it to a compass bearing to follow in the environment, subtract east declination from the true bearing, or add west declination to the true bearing. Interconversion between magnetic and true bearings is an essential skill for compass navigation. Failure to recognize this relationship will result in significant errors when following a map route by compass, because directional references on the map are based on true direction. At a location where the magnetic declination is 10 degrees, travel over a straight course derived from a map and guided by a compass will result in a 0.18-mile error for each 1 mile traveled if declination is not considered.26
Magnetic declination for any location can be determined by referencing the Isogonic Chart for Magnetic Declination, produced every 5 years by the USGS. In the United States, magnetic declination varies from 23 degrees East in Washington State to 22 degrees West in Maine (see Figure 96-8).12 On standard USGS 7.5-minute and 15-minute squares, magnetic declination is indicated by a pointer next to the indicator for true north at the bottom of the map. If one does not have access to an isogonic chart, declination for any location in the northern hemisphere can be empirically determined with reasonable accuracy by comparing the magnetic bearing of north with the true bearing as indicated by the direction to the star Polaris. Polaris lies up to 45 minutes of arc (i.e., 0.75 degrees) away from true north at some times of day, but this offset is negligible for wilderness navigation situations. Declination at any location can also be determined by comparing the magnetic bearing of a prominent landmark with the true bearing between the observer’s known location and the location of the landmark as read from a map.7
Compass Types
The three compass types used in land navigation are the fixed-dial compass, magnetic card compass, and baseplate compass (Figure 96-9, online). The simplest compass is the fixed-dial, which uses a magnetized needle that is balanced on a pivot and enclosed in a case and that is graduated around its periphery into 360 degrees. The magnetic card compass uses a magnetized needle or wire fixed to a circular card that is graduated around its periphery from 0 degrees to 360 degrees. The housing of the compass is marked with a line called the lubber line that allows magnetic bearings to be determined when the line is pointed at an object of interest. The lensatic compass used by the military, which has a lens for magnification of the compass card and sights for alignment to distant objects, is a typical magnetic card compass. The most useful compass for land navigation is the baseplate compass,26,35,46,57 which consists of a fixed-dial compass (or capsule) mounted to a baseplate in a manner that allows the capsule to rotate in relation to the baseplate. The baseplate is marked with a line used to indicate the direction of travel. This line functions in a manner identical to the lubber line of the magnetic card compass. The capsule of the compass has an orienting arrow inscribed on its lower surface that points to the graduation denoting north on the capsule rim. On different models, this graduation may be labeled “0°,” “360°,” or “N.” Rotation of the capsule such that the compass needle is superimposed on the orienting arrow and points to “N” on the capsule rim allows the user to easily read the magnetic bearing indicated by the direction-of-travel line. As long as the direction-of-travel line is followed and the needle remains superimposed on the orienting arrow, the user is assured of maintaining the desired magnetic bearing during travel.
Many baseplate compasses allow the orienting arrow to be adjusted relative to the rim of the capsule to compensate for magnetic declination. When the orienting arrow of such a compass is adjusted to point to the bearing of magnetic north, the “N” graduation on the capsule rim will indicate true north when the compass needle aligns with the orienting arrow. All bearings as read on the capsule rim will now represent true—rather than magnetic—direction. Baseplate compasses have other features particularly suited for use with a map, including plotting scales, a straightedge, and often a protractor and magnifier.57
Compass Use
Use of a compass in this manner permits the user to return easily to the desired direction of travel if an obstacle to the intended route is encountered. The course around the obstacle is recorded as a series of legs of known direction and estimated (by stride) length. When permitted by the terrain, right-angle detours are the simplest to follow. The user returns to the intended route by traveling the reciprocal of the course of the detour for the same distance as that required for bypass of the obstacle10 (Figure 96-10). Return to the intended course is greatly augmented by using natural ranges. A natural range is formed by two landmarks that lie along the same bearing line, with one end indicated by a landmark of intermediate distance from the viewer and one at a greater distance (e.g., a large tree or rock formation several kilometers from the viewer and the silhouette of a hill or mountain on the horizon). As the traveler deviates from the intended course, the near and far landmarks will fall out of line. When the traveler returns to the intended route, the objects forming the natural range will return to alignment.
Use of a compass with a map allows the user to orient the map to the environment and relate bearings taken from the map to bearings measured with the compass. Correction for declination is essential when the compass is used for this task if true bearings are to be used when plotting a route. However, there is no absolute need for the use of true bearings in navigation; it is important only that the map and compass agree. Agreement can be accomplished either by correcting the compass to the map or by correcting the map to the compass. If a baseplate compass with declination adjustment is used, it is relatively simple to correct the compass to the map and use true bearings for all subsequent travel. With all types of compasses, however, it is easiest to use magnetic bearings exclusively. If the declination is known or can be determined observationally, magnetic meridians can be drawn on the map to be used in place of the true meridians represented by the map margins (Figure 96-11, online). These magnetic meridians will form an angle with the true meridians equal to the declination angle. A map modified in this manner permits magnetic bearings (rather than true) to be taken from the map for use when following a course. The internal consistency of this method is always much less confusing than the method requiring conversion between true bearings and compass bearings. Because of the ease with which bearings may be taken from the map when it is marked with magnetic meridians, maps for use in navigational sports (e.g., orienteering) are prepared exclusively with magnetic meridians. Choice between the use of true versus magnetic bearings should be made in advance of travel to permit modification of the map. Plotting magnetic meridians on a map requires a pencil, straightedge, flat surface, and protractor; these items are unlikely to be available during a field emergency.55,57
To orient a map that has been modified with magnetic meridians using an uncompensated baseplate compass, the compass capsule is rotated until “N” on the capsule rim aligns with the direction-of-travel arrow. An edge of the compass parallel to the direction-of-travel arrow is placed on one of the magnetic meridians plotted on the map. The map, with the compass in place, is rotated until the compass needle is superimposed on the orienting arrow. True north on the map now corresponds with true north in the surrounding landscape.26,46,55,57 If an uncompensated compass of another type is used, the north–south line of the compass face or lubber line is superimposed on a magnetic meridian, and the map and compass are rotated in concert until the indicator needle points to “N.”
To plot and follow a bearing using a baseplate compass and map, the map is held horizontally, and a straightedge of the baseplate parallel to the direction-of-travel arrow is placed on a line connecting the starting and ending points of the leg. The compass capsule is then rotated until the orienting arrow points to north as indicated on the map. The map and compass are held together and rotated until the compass needle is superimposed on the orienting arrow. The intersection of the direction-of-travel arrow and the capsule rim now indicates the bearing of the leg, and the direction-of-travel arrow points to the objective (Figure 96-12). To follow the bearing, the user walks in the direction indicated by the direction-of-travel arrow while keeping the compass needle and orienting arrow in alignment.
When a map is oriented to the environment, back bearings from landmarks that are visible both on the map and in the landscape can be used to obtain a positional fix by resection or triangulation.46 Each back bearing from a landmark represents an LOP that can be plotted on the map. The point of crossing of two or more LOPs fixes the position of the observer (Figure 96-13). Alternatively, the intersection between the LOP represented by a bearing line and a shoreline, riverbank, road, firebreak, or ridgeline can be used to fix position on a map.
Makeshift Compasses
A field-expedient compass can be fabricated with relative ease. Items containing iron, nickel, or cobalt are suitable for use as an indicator needle. Iron in the form of a steel needle, pin, wire, staple, or paper clip is most commonly available. Most of these items are magnetized as purchased. If not, they can be magnetized by stroking them on a magnet salvaged from an electric motor or radio speaker, on a magnetized screwdriver or similar item, or on a piece of silk. A dry cell also can be used to magnetize a needle by wrapping an insulated wire tightly around the needle and connecting the ends of the wire to the battery terminals for several minutes. There is a fire risk associated with shorting the battery terminals in this manner, and sparks and heat should be expected. Trial and error will often yield a suitable magnetizer. The indicator needle is floated in water by placing it on a wood chip, leaf, slip of paper, or small piece of cork or closed-cell foam. The container, which may be the cupped palm of the hand or a puddle, should be protected from the wind (Figure 96-14). A compass that has been so constructed will reliably indicate a magnetic north–south line. The absolute determination of direction may require external cues, such as the general direction of sunrise, sunset, or the position of the sun at midday.

Full access? Get Clinical Tree
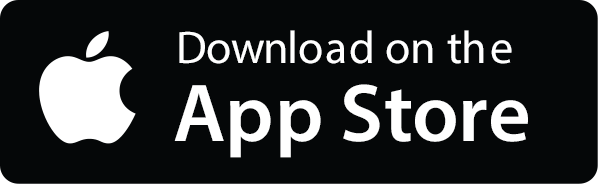
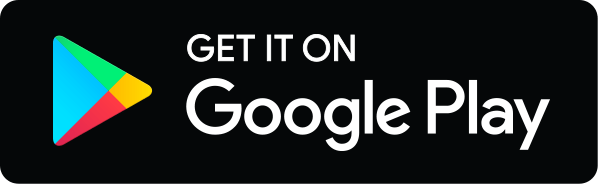