Introduction
Glucocorticoids were introduced into clinical practice in 1949 with the release of a purified preparation known as cortisone. The treatment was revolutionary for patients with primary adrenal insufficiency (AI) and for the management of other acute and chronic diseases such as rheumatoid arthritis and systemic lupus erythematous. Shortly after the introduction of cortisone, two case reports were published describing surgical patients receiving long-term glucocorticoid treatment whose treatment was held in the perioperative period. The first involved a 34-year-old man who had cortisone therapy (25 mg twice daily) discontinued 48 hours before surgery. His subsequent death was attributed to acute AI caused by abrupt withdrawal of glucocorticoids. However, extenuating circumstances may have contributed to his death. The second case involved a 20-year-old woman who had been taking 62.5 to 100 mg of cortisone daily for approximately 4 months. She died less than 6 hours after surgery; autopsy findings confirmed bilateral adrenal hemorrhages and cortical atrophy indicative of AI. From these case reports came the conventional wisdom to supplement patients receiving exogenous steroids with large “stress doses” throughout the perioperative period. This practice came under scrutiny because of questions about efficacy and concern about side effects from excessive doses.
Endogenous glucocorticoids are cholesterol derivatives produced in the zona fasciculata of the adrenal cortex. Their release is controlled by a feedback mechanism known as the hypothalamic–pituitary–adrenal (HPA) axis ( Figure 26-1 ). Corticotropin-releasing hormone (CRH), released by the hypothalamus, acts on the anterior pituitary gland to initiate the production of adrenocorticotropic hormone (ACTH or corticotropin). ACTH then stimulates the adrenal glands to produce cortisol, which acts as negative feedback for CRH in the hypothalamus. Intracellular glucocorticoid receptors known as NR3C1 are ubiquitous, and glucocorticoids are integral factors in modulating normal cellular homeostasis and metabolism. Cortisol potentiates production of catecholamines and regulates the synthesis, responsiveness, coupling, and regulation of beta-adrenergic receptors. Glucocorticoids also regulate the normal metabolism of carbohydrates, proteins, and lipids. Glucocorticoid hormones modulate cardiovascular function and wound healing and have numerous other important metabolic functions.

Daily endogenous glucocorticoid secretion is estimated to be between 5 and 10 mg/m 2 . This corresponds to 5 to 7 mg/day of oral prednisone or 20 to 30 mg/day of hydrocortisone. Cortisol synthesis can increase under conditions of stress to 100 mg/m 2 /day.
Deficiencies of glucocorticoid production result in AI, which can be classified as a primary, secondary, or tertiary process with acute and chronic forms ( Table 26-1 ). Primary AI occurs in patients who have destruction of more than 90% of the adrenal glands by hemorrhage, tumor, infection, or an inflammatory process. This results in deficient production of both mineralocorticoids and glucocorticoids. Primary AI is relatively rare, most often resulting from autoimmune destruction of the adrenal glands. In developing regions of the world, it is most commonly due to tuberculous destruction of the adrenals. Patients with primary AI always require steroid replacement/supplementation with a medication(s) that include glucocorticoid and mineralocorticoid effects. Secondary AI is also relatively uncommon and results from insufficient production of ACTH resulting from destruction or dysfunction of the hypothalamus or pituitary gland. Patients with secondary AI typically require only glucocorticoid replacement and not mineralocorticoid replacement because mineralocorticoid activity is regulated primarily through the renin–angiotensin system and remains intact.
Type | Features | Incidence | Etiologies |
---|---|---|---|
Primary |
| Prevalence: 40-110 cases/million Incidence: 6 cases/million per year |
|
Secondary |
| Uncommon |
|
Tertiary |
| Most common form |
|
Tertiary, or iatrogenic, AI is the most commonly encountered type. Tertiary AI results from the suppression of the HPA axis over time, as a result of the administration of exogenous glucocorticoids. Long-term ACTH suppression from steroid treatment leads to adrenal atrophy. This can result in a potentially harmful situation if exogenous glucocorticoids are discontinued because the adrenals can no longer produce adequate cortisol.
Normal Response to Surgical Stress
Salem and colleagues reviewed seven prospective analyses performed between 1957 and 1975 examining cortisol secretion after major surgery. Combined, the total number of subjects in these investigations was only 40. None of the patients examined were known to be adrenally insufficient or taking glucocorticoids. The reported range of 24-hour cortisol secretion was wide, varying from 60 mg/24 hr to 310 mg/24 hr. In 1972 Wise and colleagues reported 24-hour postoperative cortisol secretion to be 60 mg. The following year, Kehlet and Binder reported an immediate postoperative cortisol secretion rate of 10 mg/hr, which decreased to 5 mg/hr 24 hours after surgery. It is generally accepted, however, that most healthy, non–steroid-dependent patients will secrete somewhere between 75 and 150 mg of cortisol in the first 24 hours after major surgery or up to 100 mg/m 2 .
Integrity of the Hypothalamic–pituitary–adrenal Axis in Patients Taking Long-Term Steroids
Several studies have confirmed that patients taking small doses of steroids (≤5 mg of prednisone or its equivalent per day) retain normal HPA function. In 1973 Kehlet and Binder performed a prospective case-control study to determine whether patients receiving long-term glucocorticoid therapy could mount a physiologic response to major surgery if steroids were discontinued perioperatively. With 14 non–steroid-dependent surgical patients serving as control subjects, they prospectively followed up 74 patients on long-term glucocorticoid therapy undergoing major surgery (prednisone dose, 5 to 80 mg/day) and 30 steroid-dependent patients undergoing minor surgery (prednisone dose, 5 to 30 mg/day). Glucocorticoids were stopped 36 hours preoperatively and restarted 24 hours postoperatively. Plasma cortisol levels were measured for the first 24 hours postoperatively. Approximately 30% of the glucocorticoid-treated patients exhibited a blunted adrenocortical response to surgery, but only one patient showed any clinical signs or symptoms of AI. Interestingly, the majority of the control subjects in the minor surgery category showed little or no cortisol response to surgery. The authors concluded that subnormal adrenal stress responses were more prevalent in patients maintained on steroids at either higher doses or longer durations. Patients who received more than 12.5 mg of prednisone for more than 6 months, more than 10 mg of prednisone for more than 2 years, or more than 7.5 mg of prednisone for more than 5 years all showed an impaired adrenocortical response. The one patient who was symptomatic had no detectable plasma cortisol but was treated without resultant morbidity. Based on this report it has been hypothesized that the dose and the duration of steroid therapy influence cortisol response to stress.
In 50 patients receiving long-term low-dose prednisone (<10 mg/day for a mean duration of 41 months), La Rochelle and colleagues observed that all the patients receiving 5 mg/day or less had a normal response to a standard-dose rapid cosyntropin stimulation test. Those receiving 5.5 to 6.8 mg/day displayed an intermediate response, and those with mean doses greater than 6.8 mg/day displayed a suppressed response to ACTH stimulation.
Subsequently, Friedman and colleagues prospectively evaluated 28 patients receiving long-term glucocorticoid therapy undergoing major orthopedic surgeries. The mean duration and dose of prednisone therapy before surgery in this group was 7 years and 10 mg/day, respectively. Although patients were administered their baseline therapy up to the time of surgery, perioperative stress doses of steroids were not given. Despite this omission, no hemodynamic or biochemical changes consistent with perioperative glucocorticoid deficiency were observed.
Kenyon and Albertson performed a prospective study on 40 patients taking prednisone (doses from 5 to 10 mg/day) who were admitted to the hospital for illness, metabolic abnormalities, or surgery. No stress-dose steroids were given at any time during hospitalization. Over the first 36 hours, the authors measured serum cortisol, 24-hour urine cortisol, and ACTH levels. Once the patients’ clinical condition improved, a cosyntropin stimulation test (250 µg) was repeated. Although the response to the cosyntropin stimulation test was blunted in 63% of the subjects, 97% had normal or increased urinary cortisol concentrations. This implies that despite long-term steroid treatment, adrenal function and endogenous glucocorticoid production were sufficient to meet the stress of illness or surgery.
Evidence That Surgery-Induced Acute Adrenal Insufficiency is Harmful
The case reports from Fraser et al and Lewis et al were sufficient to convince the medical community that acute AI from perioperative glucocorticoid withdrawal had the potential to cause serious morbidity and mortality risks. In 1976 Kehlet produced an extensive review of 57 case reports from 1952 to 1973 documenting perioperative shock or death in patients taking glucocorticoids. In all cases, adverse outcomes were suspected to be secondary to stress-induced AI. The interval between surgery and shock or death ranged from preoperatively to 48 hours postoperatively. Interestingly, only 3 cases of the 57 displayed hypotension and low plasma cortisol levels, suggesting acute AI. The remainder of the cases were inconclusive or had no evidence to link the outcomes to AI.
In contrast, two large studies support the rarity of acute AI secondary to inadequate perioperative glucocorticoid coverage. Mohler and colleagues performed a retrospective review of 6947 urologic procedures in glucocorticoid-treated patients. Only one case of perioperative AI was identified (0.01% of patients). Alford and colleagues performed a similar review of 4346 cardiothoracic surgeries and confirmed only five cases of AI (0.1% of patients). These reviews support the fact that surgically induced AI can occur, although it is a relatively rare occurrence.
One group of patients that may deserve special consideration is elderly surgical patients. To determine the incidence and outcome of AI in elderly patients having high-risk surgery, Rivers and colleagues performed a prospective, observational case study. A total of 104 consecutive adult patients (excluding patients with a history of steroid use, known adrenal dysfunction, and those administered etomidate) who required vasopressor therapy postoperatively despite adequate volume resuscitation received a cosyntropin (synthetic ACTH) stimulation test with plasma cortisol measurements at 30 and 60 minutes. Empiric hydrocortisone (100 mg intravenously [IV] for three doses) was given at the discretion of the primary team. Adrenal dysfunction (defined as a serum cortisol concentration less than 20 mg/dL with a change in cortisol concentration of less than 9 mg/dL after ACTH) or functional hypoadrenalism (serum cortisol concentration less than 30 mg/dL with a change in cortisol concentration of less than 9 mg/dL after ACTH) was found in 32.7% of patients. The mortality rate was significantly lower in the hydrocortisone-treated patients with AI (21% versus 45%, p < 0.01). This incidence of relative AI is higher than would be expected for both the general surgical population and for those receiving long-term steroid treatment.
Evidence for Perioperative Steroid Replacement
Most of the clinical data on adrenal replacement therapy in the perioperative period are based on case series or drawn from clinical experience. There are few well-designed, prospective, randomized, blinded clinical trials investigating optimal perioperative steroid supplementation. Nonetheless, because of the potential for morbidity and mortality related to acute AI, it is generally agreed that certain patient populations should receive empiric perioperative steroid supplementation. The difficulty lies in determining the dose and duration of treatment.
For many years, all surgical patients taking glucocorticoids were given a standardized dose of supplementary steroid throughout the perioperative period. This method eventually came under question because of the deleterious effects of large doses of steroids, including poor wound healing, inadequate glucose control, fluid retention, hypertension, electrolyte imbalances, immunosuppression, gastrointestinal bleeding, and untoward psychological effects.
In 1975, Kehlet suggested that procedures be divided into “major” and “minor” categories. For major surgeries (e.g., intrathoracic, major vascular, or major abdominal operations), the recommendation was for 25 mg IV hydrocortisone at induction, followed by 100 mg IV hydrocortisone every 24 hours until the patient was able to resume oral steroid therapy. The goal of this approach was to adequately replace the increased cortisol requirements of 75 to 150 mg in the first 24 hours. For minor surgeries (surgeries taking less than 1 hour and those performed under local anesthetic), Kehlet suggested 25 mg IV hydrocortisone at the start of surgery and the resumption of oral therapy postoperatively. This recommendation was based on a study showing that healthy subjects often do not mount a stress response to minor surgery and, at most, secrete 50 mg/day of cortisol.
In 1978, Gran and Pahle recommended depot-betamethasone acetate/phosphate as a single intramuscular (IM) injection in perioperative patients receiving glucocorticoids. In a prospective cohort study on 1461 surgical patients receiving long-term steroid therapy, patients were given depot-betamethasone before surgery: 2 mg for major procedures and 1 mg for minor procedures. There were no reports of AI, delayed healing, or gastrointestinal bleeding. The authors contend that ease of administration is a major benefit of this regimen.
Salem and colleagues advised that perioperative supplementation should be individualized and based on prior steroid dose, duration, and degree of anticipated surgical stress. For minor surgeries, 25 mg hydrocortisone or an equivalent dose (oral prednisone or a parenteral equivalent) was suggested, and the long-term dose should be resumed the day after surgery. For procedures of perceived moderate stress, such as an open cholecystectomy or segmental colon resection, 50 to 75 mg/day of hydrocortisone or an equivalent dose (oral or parenteral) with a rapid taper over 1 to 2 days was recommended. For major surgery, such as cardiac surgery involving bypass, a target of 100 to 150 mg of hydrocortisone (or equivalent) per day with a rapid taper over 2 to 3 days was advised (see Table 26-3 later in this chapter).
Most recently, Zaghiyan et al reported no significant benefit to perioperative glucocorticoid administration (100 mg hydrocortisone IV at the time of surgery, then 100 mg hydrocortisone every 8 hours for 24 hours, then 20 mg oral prednisone tapered over 3 days) compared with no perioperative steroids among patients with inflammatory bowel disease who had received corticosteroids any time during the year preceding colon surgery. None of the patients was receiving steroids at the time of surgery. Thirty-eight patients underwent 49 surgical procedures. Perioperative glucocorticoids were administered and not administered for 11 and 38 procedures, respectively. No differences in postoperative surgical morbidity or mortality were identified between groups, although the group receiving steroid treatment had more tachycardia.
Areas of Evolving Interest and Ongoing Controversy
There are several areas of specific interest in the therapeutic administration of glucocorticoids in critically ill patients. These include treatment of patients with severe sepsis and septic shock, acute respiratory distress syndrome (ARDS), community-acquired pneumonia (CAP), meningitis, traumatic brain injury (TBI), and acute spinal cord injury (SCI). The use of etomidate in critically ill patients, a topic of renewed interest, is also reviewed.
Severe Sepsis and Septic Shock
A myriad of host regulatory responses are elicited in response to severe infection, manifesting anywhere along the spectrum of sepsis to septic shock. The result may be proinflammatory. Under normal conditions glucocorticoid production is increased to prevent the inflammatory state from overwhelming the host. However, during severe infection, the body’s ability to regulate the inflammatory state by increasing glucocorticoid production can be overwhelmed—a condition termed critical illness–related corticosteroid insufficiency (CIRCI). The recognition of this phenomenon formed the basis for several seminal studies performed in the 1970s and 1980s, in which supraphysiologic doses of corticosteroids (e.g., 30 mg/kg methylprednisolone) up to several times per day were administered to patients with septic shock. However, no survival benefit was realized, and in some instances morbidity was increased as a result of an increased incidence of secondary infectious complications. More recently, Annane and colleagues showed a survival benefit in septic patients receiving low- to moderate-dose (“physiologic”) glucocorticoids. In a prospective, randomized, placebo-controlled trial (RCT) of low-dose corticosteroids, 300 patients with septic shock refractory to fluid resuscitation and vasopressors were randomly assigned to receive 50 mg IV hydrocortisone every 6 hours plus 50 µg oral fludrocortisone daily for 7 days versus placebo. All underwent cosyntropin stimulation testing. In the 229 patients who were nonresponders to ACTH testing (76%), there was a significant reduction in the risk of death in the steroid versus placebo group (53% versus 63%, p = 0.02). In addition, the duration of vasopressor therapy was significantly shorter in patients treated with steroids. There were no significant differences in adverse events between groups. This influential study led to renewed interest and widespread clinical use of physiologic supplementation (200-300 mg/day of hydrocortisone or its equivalent) of glucocorticoids in the treatment of septic shock and sepsis-induced hypotension.
In contrast, the 499-patient Corticosteroid Therapy of Septic Shock (CORTICUS) trial reported no benefit of corticosteroid supplementation on overall survival or reversal of shock. The largest multicenter RCT to date, this study randomly assigned patients with septic shock unresponsive to fluids and vasopressors to receive steroids (50 µg hydrocortisone every 6 hours for 5 days, followed by a 6-day taper) or placebo. All underwent cosyntropin stimulation testing before treatment. In a departure from the study by Annane, there was no difference in mortality rate between the hydrocortisone and placebo groups in those unresponsive to cosyntropin stimulation. In patients whose shock was reversible, reversal occurred more quickly in the hydrocortisone group, although there were more superinfections in the treatment arm. Other side effects noted were hyperglycemia and hypernatremia.
Evidence on the administration of steroids in the treatment of sepsis is continually evolving. The 2008 Surviving Sepsis Campaign international guidelines for the management of severe sepsis and septic shock recommend that stress-dose steroid therapy only be given after conventional treatment with fluids and vasopressors has failed to restore adequate perfusion. The guidelines also suggest that cosyntropin stimulation testing not be used to identify those with septic shock who will receive hydrocortisone treatment.
Acute Respiratory Distress Syndrome and Community-Acquired Pneumonia
Corticosteroids, in doses of 1 mg/kg/day methylprednisolone or equivalent, have been reported to lead to improvement in clearing lung inflammation and lung physiologic parameters. A single-center randomized trial involving 24 patients in the fibroproliferative phase of ARDS (at 7 days after onset) reported improved lung function and survival with moderate-dose, prolonged corticosteroid administration.
However, the ARDSnet Clinical Trial Group study, a 180-patient multicenter RCT of steroids in persistent ARDS, did not report a survival benefit with steroid treatment. In this study, methylprednisolone (2 mg/kg for one dose, then 0.5 mg/kg every 6 hours for 14 days with an extended taper) was associated with reductions in shock symptoms and ventilator days, improved respiratory system compliance, and reduction in the need for vasopressor therapy but not with an improved survival rate. In addition, significantly increased 60- and 180-day mortality rates were identified in steroid-treated patients enrolled greater than 14 days after disease onset. Infectious complications were not increased, but the incidence of neuromuscular weakness was higher in the methylprednisolone-treated patients.
Conversely, another prospective RCT that administered methylprednisolone by continuous infusion in 91 patients with early ARDS (onset less than 72 hours) reported improvements in lung function and extrapulmonary organ function and reductions in both duration of mechanical ventilation and intensive care unit (ICU) length of stay. It should be noted that strict infection surveillance, tight glucose control, and avoidance of neuromuscular blocking drugs were integral parts of the protocol.
Glucocorticoid supplementation has also been recently advocated as an adjunct to antibiotics in the treatment of CAP. Despite an initial negative trial of hydrocortisone versus placebo in treatment of CAP, subsequent trials reported more promising results, which have included improved gas exchange (as evidenced by PaO 2 to FiO 2 ratio), a decreased length of ICU stay, and a lower occurrence of progression to septic shock. The largest of these trials enrolled 304 patients with severe CAP and prospectively randomized them to receive either 5 mg daily dexamethasone or placebo for 4 days. No survival benefit was seen, but the median hospital length of stay was 1 day shorter in those receiving steroids.
Given the inconsistencies in clinical evidence, the precise role of corticosteroids in ARDS and pneumonia remains elusive and requires further study before definitive recommendations can be made.
Meningitis, Traumatic Brain Injury, and Acute Spinal Cord Injury
One RCT indicates that dexamethasone, administered in conjunction with the first antibiotic dose, significantly reduces mortality rate, severe hearing loss, and neurologic sequelae in adults with community-acquired bacterial meningitis. A 2010 meta-analysis supports the reduction in hearing loss and neurologic sequelae but failed to find a survival benefit.
Despite a significant incidence of hypoadrenalism (25%) soon after TBI, there is strong evidence against routine corticosteroid treatment in head-injured patients. In a large multicenter study the risk of death from all causes within 14 days was higher in those patients with TBI who received a 48-hour infusion of corticosteroids when compared with those administered placebo. Furthermore, at 6 months, the relative risk of death or severe disability favored the placebo group.
The treatment of acute SCI with steroids is controversial. Evidence from the National Acute Spinal Cord Injury Studies (NASCIS) in the early 1990s supported high-dose methylprednisolone (30 mg/kg with infusion of 5.4 mg/kg/hr for 24 hours) after acute SCI, ideally administered within 8 hours of injury. Based on these initial studies, the treatment was widely adopted and became a standard of care. However, there has been much criticism of the study design and statistical analysis, and other conflicting clinical evidence has emerged, causing some clinicians to abandon use because of an unacceptable risk–benefit ratio. However, a 2012 Cochrane review also supports methylprednisolone use in SCI to improve both motor and sensory outcomes. The review recommends the same dosing as the NASCIS study but also recommends that therapy duration be extended to 48 hours if treatment is initiated between 3 and 8 hours after injury. In an investigation by Leypold and colleagues comparing acute SCI lesions by magnetic resonance imaging characteristics, patients who received methylprednisolone had significantly less intramedullary spinal cord hemorrhage than those who were not treated.
Indicative of the situation is a survey of 305 spine surgeons that found 90% would initiate methylprednisolone, especially if within the 8-hour window. Interestingly, many cited institutional protocols and medicolegal reasons as justification for use; only 24% used steroid treatment because of a belief in improved outcomes. An area of ongoing debate, high-dose methylprednisolone use may be effective in promoting some degree of neurologic improvement if given early after injury, although more well-designed RCTs are necessary.
Etomidate
Interest has been increasing recently in the use of the induction agent etomidate in critically ill patients, in particular for facilitation of intubation. An imidazole derivative, etomidate is often a first-line agent for endotracheal intubation or procedural sedation in the critically ill because of its minimal hemodynamic side effects. However, it is known to inhibit the 11beta-hydroxylase enzyme responsible for converting 11beta-deoxycortisol into cortisol within the adrenal gland. The potent suppression of adrenal steroidogenesis by etomidate was first described in 1984 by Wagner and White and has been shown to occur after even a single dose of etomidate. A systematic review of etomidate use in the critically ill found not only a significant increase in AI but also more ventilator-dependent days, longer hospital and ICU lengths of stay, and an increased mortality rate (relative risk for mortality versus patients not receiving etomidate, 1.19; confidence interval, 1.10 to 1.30). The risk of harm was greatest in the subset of patients with a diagnosis of sepsis. Conversely, in a retrospective review of septic patients who received either etomidate or an alternate agent to facilitate intubation, no significant outcome differences between groups were found. In an RCT in which patients without sepsis who received etomidate were randomly assigned to either receive daily hydrocortisone (200 mg IV continuous infusion) or placebo, the patients receiving steroid supplementation required vasopressor therapy for a shorter duration. However, there was no difference in length of stay, duration of ventilator use, or 28-day mortality. The results of these studies highlight the need for further investigation, as the clinical relevance of the effect of etomidate on adrenal function remains open for debate. Until further evidence is available, some authors recommend that etomidate be used judiciously in the critically ill, whereas others recommend discontinuing its use altogether, particularly in patients with severe sepsis or septic shock. In response to these concerns, an etomidate derivative, carboetomidate, has been developed. It is reported to be a less potent inhibitor of in vitro cortisol synthesis by three orders of magnitude and, in rats, caused minimal hemodynamic changes without suppressing adrenocortical function. However, human testing has yet to be performed.

Full access? Get Clinical Tree
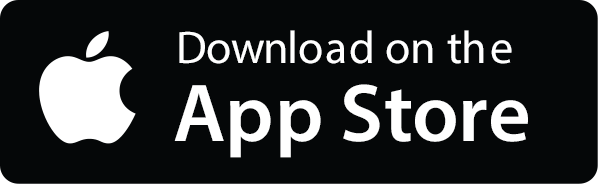
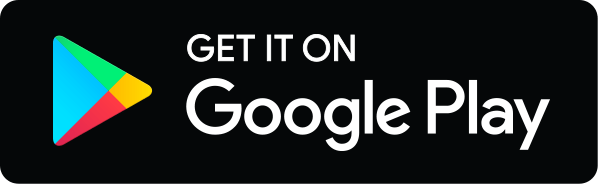