The annual prevalence of sepsis, the systemic inflammatory response to infection, is estimated at 19 million cases worldwide. Over the last 30 years, reported mortality rates in severe sepsis, defined as sepsis plus organ dysfunction, have dropped from over 80% to 20% to 30% because of advances in training, better surveillance and monitoring, prompt initiation of therapy, and organ support.
The timely and correct identification of infection followed by appropriate treatment with antibiotics is crucial to the management of critically ill and injured patients. Antibiotic therapy is founded on principles of appropriate drug selection based on (suspected) susceptibility patterns of the causative pathogen. The goal of antimicrobial administration is to achieve drug concentrations sufficiently effective to exert maximum killing at the infection site and to prevent the emergence of antimicrobial resistance. Selection of empirical antibiotics should be based on the suspected source, such as community-acquired infection or nosocomial infection, medical and culture history, and local microbial susceptibility results.
The latest guidelines for the management of severe sepsis and septic shock provided by the Surviving Sepsis Campaign (SSC) consortium recommend to begin timely appropriate intravenous (IV) broad-spectrum antibiotics after forming a probable diagnosis and obtaining cultures (1B/1C grade recommendations to administer antibiotics within 1 hour after diagnosis of either sepsis or septic shock).
However, accurate infection diagnosis in critically ill patients is confounded by the systemic inflammatory response syndrome (SIRS). Intensivists must use the same criteria (e.g., fever, leukocytosis, tachycardia, tachypnea) supplemented by clinical judgment to distinguish patients with infections from those with SIRS. Because of the devastating consequences of missing a true infection, empirical antibiotic therapy often is initiated when patients are critically ill and physicians are unable to distinguish SIRS from infection. The result is probable overuse of antibiotics.
Incorrect use of broad-spectrum antibiotics has potentially serious consequences, including Clostridium difficile infection, renal toxicity, and encouragement of multidrug-resistant organisms. These events can lead to longer intensive care unit (ICU) stays, greater health-care costs, and a higher mortality rate. The full extent of these effects in ICU patients has not been well reported, and antibiotic overuse is inevitable. There currently is no consensus or benchmark for an acceptable rate of overtreatment or for the use of empirical antibiotics in the ICU.
Diagnostic Issues
Clinical Features
Establishing a definitive diagnosis of infection is paramount to the appropriate selection and use of antimicrobials. Once infection is suspected in the ICU patient, a comprehensive workup must be performed to identify the site of infection. The microbial causes of various ICU infections are reasonably predictable once the actual site of infection is known; thus appropriate drug selection properly begins with identification of a known or suspected site of infection. Unfortunately, the site of infection is often unable to be identified with any certainty; studies in septic patients have shown that no source of infection is identified in up to 30% to 40% of patients. The clinical manifestations of sepsis are highly variable, depending on the initial site of infection, the causative organism, the pattern of acute organ dysfunction, the underlying health status of the patient, and the interval before initiation of treatment. The signs of both infection and organ dysfunction may be subtle; thus the most recent international consensus guidelines provide a long list of warning signs of incipient sepsis. In particular, clinicians must keep in mind that there are numerous sources of fever in critically ill patients that are not associated with infection. The occurrence of new fever in an ICU patient should prompt a thorough evaluation of noninfectious sources for the fever before initiation of antimicrobial therapy. Patients who have begun receiving antimicrobial therapy and have persistent fever despite the resolution of other signs and symptoms of infection should also be evaluated for noninfectious sources of fever.
Acute organ dysfunction in sepsis most commonly affects the respiratory and cardiovascular systems. Respiratory compromise is classically manifested as the acute respiratory distress syndrome (ARDS), of which sepsis is the most common cause. Cardiovascular compromise may manifest primarily as hypotension or an elevated serum lactate. After adequate volume expansion, hypotension frequently persists, requiring the use of vasopressors, and myocardial dysfunction may occur. Other organ systems are commonly affected. Central nervous system dysfunction is typically manifested as confusion, delirium, or coma. Imaging studies generally show no focal lesions, and findings on electroencephalography are usually consistent with nonfocal encephalopathy. Critical illness polyneuropathy and myopathy are also common, especially in patients with a prolonged ICU stay. Acute kidney injury is manifested as decreasing urine output and an increasing serum creatinine level and frequently requires treatment with renal replacement therapy. Paralytic ileus, elevated bilirubin/aminotransferase levels, altered glycemic control, thrombocytopenia and disseminated intravascular coagulation, adrenal dysfunction, and the nonthyroid illness syndrome are all relatively common in patients with severe sepsis.
Gram Stain and Culture as an Aid to Empirical Therapy
Microbiologists have access to a wide range of invasive and noninvasive diagnostic techniques, and these should be used when appropriate. The institution of antimicrobial therapy should not be delayed for the sake of performing exhaustive diagnostic tests. Gram stain of appropriate specimens from potential sites of infection should be used to help determine appropriate empirical or antimicrobial therapy. Although the yield of useful information from Gram stains is usually not high in critically ill patients, performing this test is nevertheless of value for those patients in whom causative pathogens are identified. Gram stains from specimens obtained from certain sites such as the respiratory tract and wounds should be interpreted with caution because of high rates of colonization with nonpathogenic organisms, particularly in patients who have already been hospitalized for several days. Studies have clearly demonstrated the high frequency and rapid time course of microbial colonization of ICU patients. Classic studies demonstrated that rates of colonization of the oropharynx and bronchi of critically ill patients with gram-negative organisms reached 45% and 65% within 5 days after ICU admission, respectively, and more than 90% at both sites by day 10. These patients also become highly colonized with gram-positive cocci and particularly yeast soon after ICU admission.
Great care must be taken to differentiate colonizing organisms from true pathogens when evaluating Gram stain and culture results from nonsterile areas of the body or areas that may become colonized after the placement of foreign devices such as catheters (e.g., the urinary tract and respiratory tract). Colonization is often distinguished on the basis of Gram stain results showing multiple morphologic types of bacteria or the absence of clinically relevant signs and symptoms of infection despite the presence of microbial growth. However, in critically ill patients, colonization is often extremely difficult to distinguish from true infection, and antimicrobials are initiated based on a presumptive diagnosis.
Blood Cultures
The role of blood cultures is crucial for the correct fine-tuning of antibiotic therapy in sepsis. Blood cultures are the current “gold standard” of bloodstream infection diagnosis and are based on the detection of viable microorganisms present in blood. Blood cultures have the advantage of allowing for the evaluation of their antimicrobial susceptibility; this characteristic has still not been paralleled by any other technique available to date. This aspect is important because several studies have shown that inadequate antimicrobial therapy is an independent risk factor for mortality or microbiological failure for severely ill patients with life-threatening infections (see later).
However, several factors may still reduce the overall sensitivity of blood cultures. An intrinsic limitation of blood cultures is their low sensitivity to slow-growing and fastidious organisms such as Bartonella spp., Francisella tularensis , Mycoplasma spp., several molds, and Nocardia spp. Other uniformly uncultivable pathogens (by the usual bacterial culture systems) such as Rickettsia spp., Coxiella burnetii , Chlamydophila pneumoniae , and Tropheryma whipplei are better diagnosed by immunodiagnostic or molecular techniques. The presence of multiple interfering factors such as previous antimicrobial therapy, suboptimal sample collection, or incorrect preanalytic processing may deliver false-negative results even in septic patients with easy-to-culture pathogens such as Staphylococci and Streptococci .
An important factor influencing blood culture diagnostic yield is blood volume. Several studies of adults and pediatric patients confirmed that the rate of isolation from blood cultures increases with the quantity of blood submitted. Another important variable is the time taken from blood withdrawal to the loading of blood culture bottles into the instrument. Ideally, blood cultures should be loaded immediately into the continuous-monitoring instrument to minimize the time to detection and to reduce the number of false-negative samples caused by delays in loading. A decrease in recovery has been observed when bottles are held at room temperature for more than 12 hours and even more so when they are preincubated at 37° C before being loaded into the automatic instrument.
Role of Rapid Microbiological Diagnostics to Guide Empirical Therapy
The role of the clinical microbiology laboratory in the acute phase of sepsis has traditionally been marginal because at least 24 to 72 hours are necessary for the confirmation of an infectious etiology, identification of the pathogen, and evaluation of its antimicrobial susceptibility. However, with the advent of rapid speciation methods, clinicians are encountering increasing windows of time when they are aware of an infecting organism’s species without yet knowing its susceptibilities. The most promising techniques are proteomic technologies, including matrix-assisted laser desorption-ionization time-of-flight mass spectrometry. This technique is able to identify bacteria or fungi by determining their proteomic profiles. It has also been used to identify bacterial virulence factors or antibiotic resistance markers. This method has the main advantage of allowing a definitive identification, or typing, of isolated microorganisms in only a few minutes. In addition, several pathogen-specific, broad-range, and multiplex polymerase chain reaction–based amplification strategies have been used to identify positive blood cultures, to aid in rapid speciation, or to diagnose sepsis directly from blood samples. Other molecular methods are being used, including fluorescence in situ hybridization with oligonucleotide probes targeting bacterial or fungal genes (typically ribosomal RNA genes).
With the advent of rapid speciation techniques, there is an opportunity to improve antimicrobial coverage with the added possibility of decreasing unnecessary antimicrobial usage. This will suddenly make institutional species-specific antibiograms much more clinically useful. Moreover, clinicians may be able to more quickly discontinue therapy after identification of obvious culture contaminants (e.g., Corynebacterium species) or more rapidly de-escalate therapy after identification of organisms with predictable susceptibilities (e.g., Listeria monocytogenes ). In some instances, species identification will result in pathogen-guided escalation before susceptibility-guided de-escalation of empirical therapy (e.g., Enterococcus species or Pseudomonas species).
Despite the remarkable technical advances of nucleic acid testing–based approaches, their widespread use for the microbiological diagnosis of sepsis is still limited by several shortcomings. For example, the detection of circulating microbial DNA (DNAemia) does not necessarily indicate the presence of a viable microorganism responsible for a given infection. The high sensitivity needed for the diagnosis of sepsis may increase the risk of false-positive results due to carryover contamination or due to the detection of environmental DNA contaminating the blood sample. Moreover, DNAemia may be the footprint for transient bacteremia not related to any infection, or it may be related to the persistence of circulating DNA still detectable several days after successful anti-infectious therapy has been completed. Another major drawback of the available molecular assays for the diagnosis of sepsis is that they do not provide information on the antimicrobial susceptibility of the detected pathogen.
The rapid detection of a pathogen may allow for better fine-tuning of empirical therapy with possible economic savings, but the lack of a specific susceptibility spectrum, especially with multidrug-resistant pathogens on the rise, may limit the clinical usefulness of these assays. In cases in which the presence of a single gene is always associated with phenotypic resistance (i.e., the mecA gene for oxacillin resistance and van genes for vancomycin resistance), it is relatively simple to design molecular strategies that allow their detection. More troublesome are cases in which the phenotypic resistance is influenced by several concurrent factors, such as the regulatory role of distinct genes that modulate the levels of expression of the gene(s) determining resistance.
Other Assays to Guide Empirical Antibiotic Therapy
Fungi continue to be a major cause of infection-related mortality principally for ICU patients and patients with hematologic malignancies. However, early diagnosis remains a challenge, mainly because of the low specificity of clinical symptoms and the low sensitivity of fungal cultures. The development of an enzyme-linked immunosorbent assay for the detection of galactomannan (GM), an Aspergillus sp. cell wall component, has been an important advance for the diagnosis of invasive aspergillosis. A positive test confirmed with two sequential samples is considered to be a valid index for invasive aspergillosis diagnosis with European Organisation for Research and Treatment (EORTC) diagnostic criteria. Although the detection of GM in serum is easy to perform, a major disadvantage of the serodiagnosis of invasive aspergillosis is the occurrence of false-positive results.
β-Glucan (BG) is another component of the fungal cell wall present in a wider variety of fungal species, including Candida spp. For most patients with confirmed invasive fungal infections, BG levels were elevated several days before clinical diagnosis.
The limitations of blood cultures have also fostered interest in the development of sensitive and rapid laboratory tests aimed at detecting nonspecific biomarkers of sepsis. Assays for C-reactive protein, procalcitonin (PCT), interleukin (IL)–6, and IL-8 have been evaluated for their clinical usefulness. PCT is a propeptide of calcitonin that is ubiquitously expressed as part of the host’s inflammatory response to various insults. A growing body of evidence suggests that PCT is a marker of severe bacterial infection and can distinguish patients who have sepsis from patients who have SIRS. In particular, PCT levels in plasma have been correlated with sepsis-related organ failure scores and may be useful in risk assessment. High and persistent elevations in PCT levels have been associated with poor outcomes for ICU patients. Although several studies suggested that PCT is among the most promising biomarkers for sepsis, considerable controversy surrounding its clinical usefulness still remains. A recent meta-analysis indicated that PCT cannot reliably differentiate sepsis from other noninfectious causes of SIRS in critically ill adult patients.
Early Treatment: The Evidence
In a well-known study, Rivers et al. demonstrated an impressive absolute reduction of 16% in the in-hospital mortality rate of septic shock and severe sepsis in their single-center, prospective, randomized trial of early goal-directed therapy (EGDT). This was the first study to demonstrate that there was a golden hour (or 4 to 6 hours) for patients with severe sepsis.
The results of the Australasian Resuscitation in Sepsis Evaluation (ARISE) trial and the Protocol-Based Care for Early Septic Shock (ProCESS) study, both of which evaluated the use of resuscitation bundles in sepsis, seem to refute the findings of Rivers et al. These trials failed to show benefit from EGCDT versus controls. One of the criticisms of EGDT as described by Rivers and of other sepsis bundles concerns the uncertain effect that individual parts of the bundle have on survival. EGDT as a whole seems to save lives, but individual parts of the therapy may not be efficacious (central mixed venous oxygen monitoring) or may even be harmful to patients with sepsis (i.e., blood transfusion). The only consistently important factor in several multivariate analyses of the utility of individual elements of the sepsis bundle has been rapid antimicrobial initiation. Indeed, in the ARISE and ProCESS studies, septic shock was recognized early in most patients. Seventy-six percent of the patients in ProCESS received antimicrobial agents by the time they underwent randomization, which occurred a mean of 3 hours after patients’ arrival in the emergency department (ED). The rate of antimicrobial administration 6 hours after randomization was approximately 97%, which undoubtedly contributed to the higher rates of survival than projected in this study.
The potential influence of delayed antibiotic therapy was first evaluated in patients with community-acquired pneumonia (CAP). In a study involving 297 U.S. acute care hospitals, Kahn et al. observed a 4% point reduction in 30-day mortality among Medicare patients who received antibiotics within 4 hours of admission and appropriate oxygen therapy. In the early 1990s, McGarvey and Harper demonstrated that care processes that included antibiotic delivery within 4 hours were associated with lower pneumonia mortality at two community hospitals. Meehan and colleagues undertook a multicenter retrospective study of 14,069 patients with CAP treated in 3555 U.S. acute care hospitals and demonstrated that administration of antibiotics within 8 hours of hospital arrival and collecting blood cultures within 24 hours were associated with improved 30-day survival. More recently, Houck et al. described that among 13,771 patients who had not received outpatient antibiotic agents, antibiotic administration within 4 hours of arrival at the hospital was associated with reduced in-hospital mortality (6.8% vs. 7.4%; adjusted odds ratio [AOR], 0.85; 95% confidence interval [CI], 0.74 to 0.98) and mortality within 30 days of admission (11.6% vs. 12.7%; AOR, 0.85; 95% CI, 0.76 to 0.95).
In a study of 261 patients in the ED, Gaieski et al. confirmed the association with timing of antibiotic therapy and mortality in patients with severe sepsis or septic shock.
In addition, Kumar and colleaguers, in a retrospective cohort study of 2154 patients who received empirical antibiotic therapy, observed that the survival was 80% in patients given antibiotics within the first hour of persistent or recurrent hypotension. However, for each hour of delay during the subsequent 6 hours, the chances of survival decreased by 7.6%. In multivariate analysis, the strongest predictor of outcome was time to effective antibiotic administration. Only half the patients received effective antibiotics within 6 hours of hypotension onset, and 30% had delays of more than 12 hours. It is important to point out that this was a retrospective study over 15 years, and recruitment rates were relatively low, with 2154 patients included from 10 sites (14 ICUs). Only 12% of patients had received antibiotics within the first hour. In addition, Kumar et al. focused on septic shock patients with appropriate antibiotic treatment.
In a prospective observational study in 77 ICUs based on propensity scores and adjusting for other treatments, Ferrer et al. reported that among 2796 severe sepsis/septic shock patients, empirical antibiotic treatment reduced mortality (treatment within 1 hour vs. no treatment within first 6 hours of diagnosis; odds ratio [OR] 0.67; 95% CI, 0.50 to 0.90; P = .008).
In a more recent retrospective analysis of a large dataset of 28,150 patients with severe sepsis and septic shock prospectively collected for the SSC in Europe, the United States, and South America, Ferrer and colleagues showed that delay in first antibiotic administration was associated with increased in-hospital mortality. There was a linear increase in mortality risk for each hour of delay in antibiotic administration. Reducing the time to first antibiotic from more than 6 hours to less than 1 hour may result in a mortality reduction of 9.5% (33.1 to 24.6%). These data demonstrate that the association between timing of antibiotic administration and mortality is true not only for patients with septic shock but also for patients with severe sepsis. Importantly, the beneficial effects of early antibiotic administration reported in this study are based on time from sepsis diagnosis and are not related to onset of hypotension.
On the basis of this evidence, the SSC guidelines recommend that after the recognition of severe sepsis or septic shock, IV broad-spectrum antibiotics should be administered as early as possible and always within 1 hour (for patients identified on the general medical wards) or 3 hours (for patients identified in the ED).
The relationship of prompt antibiotics and better outcomes might represent a surrogate marker for the quality of care in a broader sense. Other important sepsis treatments have shown time dependency, such as quantitative resuscitation or source control. In fact, in a systematic review and meta-analysis, Barochia et al. showed that the implementation of SSC bundles was followed by an improvement in most of the sepsis process-of-care variables, including time-to-antibiotic treatment, followed by a mortality reduction.
Appropriate Drug Selection
Initial selection of adequate or appropriate drug therapy also appears to be of importance in optimizing outcomes of antimicrobial use in critically ill patients. Few would argue with the initial use of broad-spectrum agents. Initial empirical anti-infective therapy should include one or more drugs that have activity against the likely pathogens (bacterial or fungal) and that penetrate into the presumed source of the sepsis. The choice of drugs should be guided by the susceptibility patterns of microorganisms in the community and in the hospital.
Selection of inadequate therapy has been demonstrated in numerous clinical studies to be associated with increased patient mortality, and the risk of inadequate therapy is often directly related to rates of antimicrobial resistance in certain pathogens. Simply put, adequate therapy is more than a reflection of “sensitive” or “resistant.” It needs to be governed by breakpoints that are relevant to the mode of action of an antibiotic and the probability that at any given dose, a drug will exceed either the concentration required for killing most strains of a given bacterial species (concentration-dependent killing) or that the concentration will remain above the minimum inhibitory concentration (MIC) of the strains for certain time periods (time-dependent killing).
Retrospective studies conducted in the 1960s and 1970s have shown that appropriate antimicrobial therapy, defined as the use of at least one antibiotic active in vitro against the causative bacteria, reduced the mortality of gram-negative bacteremia when compared with patients receiving inappropriate therapy. In a landmark study of 173 patients with gram-negative bacteremia, who were classified in three categories according to the severity of the underlying disease categories (i.e., rapidly fatal, ultimately fatal, and nonfatal), McCabe et al. observed that appropriate antibiotic therapy reduced mortality from 48% to 22%. Four subsequent studies that included larger numbers of patients yielded similar results. In a more recent prospective study of 2124 patients with gram-negative bacteremia, mortality was 34% in 670 patients who received inappropriate antibiotics and 18% in 1454 patients who received appropriate antibiotics. Smaller recent studies showed that the appropriateness of the antibiotic regimen favorably influenced the outcome of patients infected with specific gram-negative bacteria, such as Enterobacter species, Pseudomonas aeruginosa, and ceftazidime-resistant Klebsiella pneumoniae or Escherichia coli.
Fewer data have been published on the impact of appropriate antibiotic therapy in patients with gram-positive sepsis. Several studies evaluated the impact of appropriate antimicrobials in patients with severe infections due to gram-negative and gram-positive bacteria. In all but one study, appropriate antibiotic therapy was associated with a better outcome. Such studies are likely to involve special groups of organisms that are known to be less virulent: thus their role as pathogens is difficult to substantiate. This would include organisms such as coagulase-negative staphylococci and enterococci, in which there is controversy concerning either the diagnosis or attributable mortality and which are intrinsically less virulent organisms than gram-negative rods or Staphylococcus aureus. The study by Ibrahim et al. of patients with bacteraemia in critical care units showed those treated inadequately with antimicrobials fared far worse than those treated adequately (mortality 61.9% vs. 28.4%, P < .001), with almost one third receiving inadequate initial cover. Pathogens inadequately covered included Candida species in more than 8%, vancomycin-resistant enterococci, coagulase-negative staphylococci, and P. aeruginosa . The presence of fungal infection, prior administration of antibiotics, and central venous catheters each independently increased risk of inadequate cover.
Empirical Antimicrobial Selection
General Considerations
Because initial selection of adequate drug therapy is of vital importance in optimizing outcomes of antimicrobial use in critically ill patients, several factors are important to consider when choosing initial empirical therapy. These include suspected site(s) of infection (and corresponding potential pathogens), the patient’s immunologic status, therapy for a nosocomial- or community-acquired infection, rates of resistance of these pathogens to potentially used drugs, a patient’s prior exposure to antimicrobial therapy that may potentially increase the likelihood of antimicrobial resistance, and the results of any pertinent prior diagnostic tests. A reasonable understanding of the pharmacology, pharmacokinetics, pharmacodynamics, potential toxicities, potential drug interactions, and appropriate dosing of individual antimicrobials is also important in the selection of a specific agent once the antimicrobial has been chosen. In general, empirical antimicrobial regimens for critically ill patients should be aggressive (i.e., sufficiently broad spectrum in pharmacologic activity to cover the most likely [rather than all possible] pathogens, initiated promptly, and given in relatively high doses when the presence of any significant renal or hepatic dysfunction is considered).
Source and Microbiology of Sepsis
In a recent meta-analysis and review, Bochud and colleagues identified the predominant sources of infection in patients with severe sepsis and septic shock, by decreasing order of frequency, as the lungs, the bloodstream (without another identifiable source), the abdomen, the urinary tract, and soft tissues. This is corroborated by a multicenter prospective cohort study by Sands and colleagues, in which, in 866 cases of sepsis syndrome, respiratory infections were the most common, accounting for 42.4% of all infections. This was followed by bloodstream infections of undetermined origin (12.0%). Likewise, in a multicenter prospective study in French public hospitals, the primary source of infection was also pleuropulmonary, with almost half (41%) accounting for total episodes of documented severe sepsis. However, in this cohort, intra-abdominal infections accounted for 32% of episodes of sepsis in which a unique source was identified, whereas primary bacteremia was identified in only 4% of cases. Kumar and colleagues similarly found that pleuropulmonary, intra-abdominal, and urinary tract source infection were, in order, the largest contributors to a large (n = 5715), multi-institutional cohort of septic shock cases.
Data from various sources indicate that there have been important changes in the microbial etiology of sepsis over recent decades. The largest survey of sepsis epidemiology reviewed more than 10 million cases of sepsis over a 22-year period (1979 to 2000) from a nationally representative sample of U.S. acute-care hospitals. From 1979 to 1987, gram-negative bacteria were the predominant organisms causing sepsis, but they were overtaken by gram-positive bacteria thereafter. In 2000, among organisms reported to have caused sepsis, gram-positive bacteria accounted for 52.1% of cases, with gram-negative bacteria accounting for 37.6%, polymicrobial infection for 4.7%, anaerobes for 1.0%, and fungi for 4.6%. There was no specific breakdown of causative organisms to species level. A more recent longitudinal study from Spain had similar findings. This study examined 27,419 episodes of significant bloodstream infection in 22,626 patients from a single general hospital in Madrid over a 22-year period (1985 to 2006). There was an increase in the overall incidence of bloodstream infection throughout the study period, from 130.3 per 100,000 population in 1985 to 269.8 per 100,000 population in 2006. Overall, 55% of episodes of bloodstream infections were caused by gram-positive bacteria and 44% by gram-negative bacteria. In a pattern similar to the U.S. study, there was a similar number of episodes caused by both gram-positive and gram-negative bacteria during 1985 to 1987, but gram-positive bacteria became much more predominant thereafter, although the numbers were very similar again for 2004 to 2006. This relative increase in sepsis caused by gram-positive bacteria reflected a general increase in the incidence of all major gram-positive bacteria, although there were marked increases in the incidence of bloodstream infection associated with coagulase-negative staphylococci and Streptococcus pneumoniae . The incidence of S. aureus bloodstream infection increased steadily throughout the study period, from 24.3 episodes per 100,000 population in 1985 to 30.8 episodes per 100,000 population in 2006. E. coli was the most predominant gram-negative bacterial cause of bloodstream infection, with an incidence of 23.5 episodes per 100,000 population in 1985, increasing to 79.1 episodes per 100,000 population in 2006. Bochud et al. identified the gram-positive organisms responsible for sepsis syndromes as predominantly S. aureus , coagulase-negative staphylococci, enterococci, and streptococci. In contrast, gram-negative sepsis is commonly caused by members of the family Enterobacteriaceae, especially E. coli , K. pneumoniae , and P. aeruginosa.
There was a 207% increase in the number of cases of sepsis caused by fungal organisms, from 5231 cases in 1979 to 16,042 in 2000. Most causes of fungal sepsis were caused by Candida species, which are the fourth most common cause of bloodstream infection and are associated with high mortality. The Madrid study showed a steady increase in fungal bloodstream infections throughout the study period, with a progressive increase in non-albicans Candida spp. The overall incidence of fungal bloodstream infection was 1.7 episodes per 100,000 population in 1985 and 12.5 episodes per 100,000 population in 2006.
Anaerobic bacteria are a relatively uncommon cause of sepsis, accounting for 1.0% of cases of sepsis in the United States during 1979 to 2000 and 4.1% of episodes of bloodstream infection in Madrid during 1985 to 2006.
Combination Therapy and Monotherapy
There are several potential advantages to using combination anti-infective therapy for serious, life-threatening infections : (1) an increased likelihood that the infective pathogen will be susceptible to at least one of the components of the dual regimen, thereby allowing appropriate initial therapy; (2) prevention of emergence of resistance during therapy; and (3) additive or synergistic effect of the antimicrobials. In contrast, the disadvantages of using a combination of drugs include a greater likelihood of adverse effects, increased cost, possible antagonism between specific drug combinations, and the propagation of antimicrobial resistance.
Although several studies have attempted to address the issue of whether combination antimicrobial therapy improves outcomes in sepsis compared with a single agent, a consensus has not been reached. There are several reasons for this. Many studies are observational in nature. In these studies, factors such as selection bias and confounding by indication are difficult to avoid, especially with the use of relatively subjective criteria such as clinical response rather than mortality. Another difficulty is that most randomized studies are designed to assess noninferiority. These studies are explicitly designed with a structural bias in favor of showing equivalence between a newer, more pharmacodynamically potent drug and a combination of two weaker agents. In addition, randomized controlled trials (RCTs) often do not have sufficient numbers of a particular type of microorganism or a particular patient population (such as septic shock) to allow robust subgroup analyses, and as such, synergy and emergence of resistance cannot be rigorously assessed. Meta-analyses that have combined the results of individual studies allow for critical assessment of the literature, identification of important gaps and limitations, and generation of hypotheses for future trials, but they may also suffer from the heterogeneity and intrinsic weaknesses/deficits of the included studies. The evidence for specific combination versus monotherapy is discussed later.
Community-Acquired Pneumonia
S. pneumoniae remains the most prevalent and lethal cause of CAP. However, in patients with CAP who require admission to the ICU, Legionella , gram-negative bacilli, S. aureus , and influenza are also important. Risk factors for CAP due to gram-negative bacilli include previous antibiotic therapy, prior hospitalization, immunosuppression, pulmonary comorbidity (e.g., cystic fibrosis, bronchiectasis, or repeated exacerbations of chronic obstructive pulmonary disease that require frequent glucocorticoid or antibiotic use), probable aspiration, and medical comorbidities (e.g., diabetes mellitus, alcoholism). Community-associated methicillin-resistant Staphylococcus aureus (MRSA) pneumonia remains uncommon, but it typically produces a necrotizing pneumonia with high morbidity and mortality.
The three most frequently recommended initial antibiotic regimens for hospitalized patients with CAP, which have activity against its major causes, include (1) an extended spectrum β-lactam (e.g., amoxicillin/clavulanic acid) with a macrolide, or (2) an extended spectrum β-lactam with a fluoroquinolone, or (3) an antipneumococcal quinolone alone. This last option is increasingly recognized as inferior to combination therapy for severe CAP. Several medical societies have issued guidelines for the treatment of CAP. Recommendations for antibiotic regimens for CAP have been issued by a collaboration between the Infectious Diseases Society of America (IDSA)/American Thoracic Society (ATS) in 2007 and separately by the British Thoracic Society (BTS) in 2009. For patients with severe CAP requiring ICU admission, the IDSA/ATS guidelines recommend a β-lactam (ceftriaxone, cefotaxime, ampicillin-sulbactam) plus either IV azithromycin or an antipneumococcal fluoroquinolone unless there is concern for Pseudomonas or MRSA infection. If Pseudomonas is a concern, an antipseudomonal agent (piperacillin-tazobactam, imipenem, meropenem, or cefepime) plus an antipseudomonal fluoroquinolone (ciprofloxacin or high-dose levofloxacin) should be used. If MRSA is a concern, then either vancomycin or linezolid should be added.
Arguments against dual antibiotic therapy focus on the lack of robust evidence of benefit and the potential harms of such therapy. A recent meta-analysis of randomized clinical trials of antibiotic therapy for CAP failed to find a mortality benefit with dual therapy. Furthermore, the use of broader dual antibiotic coverage increases the risk of antibiotic resistance. For example, macrolide use has been associated with increased risk of macrolide-resistant and penicillin-resistant S. pneumoniae isolates in patients with invasive pneumococcal disease. Finally, more antibiotic therapy increases the risk of adverse drug effects. For example, previous studies have found independent associations between macrolide use and the risk of cardiovascular morbidity.
However, as the guidelines suggest, and despite similar spectra of activity, emerging evidence from mostly retrospective studies suggests the superiority of dual therapy over monotherapy, particularly for patients with severe CAP, or bacteremic pneumococcal pneumonia.
In the study by Rodriguez and colleagues, a secondary analysis of a prospective observational cohort was undertaken for patients with CAP who developed shock. Among the 529 patients recruited for the original study, 51% or 270 patients required vasoactive support and were characterized as having shock. Among those patients, combination antibiotic therapy was associated with a significantly higher 28-day adjusted in-ICU survival (hazard ratio [HR] 1.69, CI, 1.09 to 2.6). In addition, even when monotherapy was appropriate in vitro, it still provided a lower 28-day adjusted ICU survival than an adequate antibiotic combination (HR 1.64, CI, 1.01 to 2.64). Of note, combination regimens were further examined to determine whether the difference seen in survival rate with combination or monotherapy was secondary to a specific antibiotic or combination thereof. When compared with monotherapy, survival rates were higher for antibiotic combinations, including β-lactam plus macrolide (HR 1.73, CI, 1.08 to 2.76) and β-lactam plus fluoroquinolones (HR 1.77, CI, 1.01 to 3.15).
More recently, Rodrigo et al. used data from the BTS national audits to retrospectively compare the outcome of CAP for 3239 patients treated with dual β-lactam and macrolide antibiotics with that for 2001 patients treated with β-lactams alone. The authors found that after adjusting for CURB65 scores, age, sex, comorbidities, IV administration of antibiotics, nursing home residency, and admission to ICU, the OR of mortality of patients treated with dual therapy was 0.72 (CI, 0.60 to 0.85) compared with those treated with β-lactams alone. Analyzing the data according to CURB65 score indicated that the beneficial effect of dual therapy was mainly for patients with moderate-severity CAP (CURB65 2) who had a startling mortality OR of 0.54 (CI, 0.41 to 0.72) compared with patients given β-lactams alone. There was also some effect for patients with severe CAP (CURB65 3+; OR, 0.76; CI, 0.60 to 0.96) but none for those with mild CAP (CURB65 0−1; OR, 0.80; CI, 0.56 to 1.16).
A recent meta-analysis of 16 observational studies comparing β-lactam–macrolide combination with a single β-lactam in more than 42,000 patients with all-cause pneumonia found a lower risk of death in favor of the combination treatment (OR, 0.67; 95% CI, 0.61 to 0.73).
Finally, more definitive evidence has been provided by an open-label, multicenter, noninferiority, randomized trial of 580 patients admitted to six hospitals in Switzerland for mildly to moderately severe CAP (Pneumonia Severity Index [PSI] categories I to IV). Patients were randomly allocated to receive monotherapy with a β-lactam or dual therapy with a β-lactam and a macrolide. Legionella pneumophila infection was diagnosed with urinary antigen testing, and macrolide therapy was added for patients in the monotherapy arm who had a positive test result. The primary study outcome was the proportion of patients not reaching clinical stability at hospital day 7 with validated criteria. For this outcome, a predefined noninferiority boundary of 8% was assessed with a one-sided 90% CI. Secondary 30- and 90-day outcomes included mortality, readmission, recurrence of pneumonia, and adverse effects to antibiotics. On hospital day 7, more patients in the monotherapy arm compared with the dual therapy arm had not reached clinical stability (41.3% vs. 33.4%; P = .07). The upper limit of the one-sided CI for this 7.9% difference was 13.3%, which exceeded the predefined noninferiority boundary. Planned subgroup analyses found a significant delay in reaching clinical stability for patients in the monotherapy arm infected with atypical pathogens (HR, 0.33; 95% CI, 0.13 to 0.85) and a trend toward a delay in PSI category IV (HR, 0.81; 95% CI, 0.59 to 1.10). Although a smaller proportion of patients treated with dual therapy than monotherapy were readmitted at 30 days (3.1% vs. 7.9%; P = .01), none of the other secondary outcomes varied between the treatment arms. On the basis of a well-designed noninferiority trial, the authors rejected their primary hypothesis that monotherapy was noninferior to dual therapy.
On the basis of this evidence, it appears that the benefits of combination therapy for CAP may not be limited to those only with severe CAP (PSI category IV/V) or with pneumococcal bacteremia and septic shock but may extend to all patients hospitalized with CAP. Possible explanations for the apparent beneficial effects of combination therapy in CAP include coverage for atypical pathogens (which account for up to 20% of moderate to severe CAP), polymicrobial infections, resistant pathogens, synergistic effects, and the anti-inflammatory immunomodulatory effects of the macrolides. That macrolide antibiotics are unique in that they not only inhibit the production of pneumolysin and other pneumococcal virulence factors but possess neutrophil-directed anti-inflammatory properties may account for the advantage of using them as part of combination therapy for severe CAP.
Ventilator-Associated Pneumonia
Most health-care–associated pneumonia is ventilator-associated pneumonia (VAP), which is the most common nosocomial infection acquired in the ICU. VAP develops in 10% to 20% of patients who undergo mechanical ventilation for longer than 24 hours and is associated with longer ICU stays, increased costs, and increased mortality.
The type of organism that causes VAP usually depends on the duration of mechanical ventilation. In general, early VAP is caused by pathogens that are sensitive to antibiotics whereas late-onset VAP is caused by multidrug-resistant and more difficult to treat bacteria. Typically, bacteria causing early-onset VAP include S. pneumoniae (as well as other Streptococcus species), Hemophilus influenzae , methicillin-sensitive S. aureus , antibiotic-sensitive enteric gram-negative bacilli, E. coli , K. pneumonia, Enterobacter species, Proteus species, and Serratia marcescens. Culprits of late VAP are typically multidrug-resistant bacteria, such as MRSA, Acinetobacter , P. aeruginosa , and extended-spectrum β-lactamase (ESBL)–producing bacteria. Commonly found bacteria in the oropharynx can attain clinically significant numbers in the lower airways. These bacteria include Streptococcus viridans, Corynebacterium , coagulase-negative Staphylococcus , and Neisseria species. VAP is frequently due to polymicrobial infection. VAP from fungal and viral causes has a very low incidence, especially in the immunocompetent host.
According to the ATS and IDSA treatment guidelines for health-care–associated pneumonia, recommendations for empirical treatment of early VAP are single-agent ceftriaxone, ampicillin/sulbactam, or a fluoroquinolone. In contrast, regimens for late VAP, which is more commonly caused by multiresistant organisms such as Pseudomonas spp., Acinetobacter , or MRSA, include a carbapenem with or without vancomycin or combination therapy composed of an aminoglycoside or quinolone with an antipseudomonal penicillin, a β-lactam/β-lactamase inhibitor combination, ceftazidime, or cefepime. Guidelines issued by the British Society for Antimicrobial Chemotherapy recommend co-amoxiclav or cefuroxime for patients with early-onset infections who have not previously received antibiotics and have no other risk factors for multidrug-resistant pathogens. In those who have previously received antibiotics or who have other risk factors, a third-generation cephalosporin (cefotaxime or ceftriaxone), a fluoroquinolone, or piperacillin-tazobactam would be appropriate. Acceptable treatment options for late-onset VAP according to these guidelines include ceftazidime, ciprofloxacin, meropenem, and piperacillin-tazobactam. When MRSA is a possibility, vancomycin or linezolid should be included in the antibiotic regimen. Although linezolid penetrates lung tissue better than vancomycin, a recent meta-analysis of RCTs suggest that it is no better than vancomycin.
In a meta-analysis of suspected VAP by Aarts and colleagues comprising 1805 patients, a total of 11 trials compared monotherapy with combination therapy. Eight of the 11 trials, composed of a total of 1459 patients, reported mortality. In a pooled analysis, there was no mortality difference for patients receiving monotherapy in comparison with combination therapy (relative risk [RR], 0.94, 0.76 to 1.16). Likewise, outcomes did not change in a sensitivity analysis of treatment failure (RR, 0.92, 0.72 to 1.17) or in the five trials exclusively enrolling ventilated patients (mortality RR, 0.95, 0.68 to 1.32). The investigators concluded that it did not appear likely that combination therapy was clinically superior to monotherapy.
It appears that the only reason to use combination therapy for VAP that is currently supported by evidence is the increased likelihood of appropriate initial anti-infective therapy. Once the organism is identified, then de-escalation to a single drug should be used if permitted by the susceptibility testing.
Sepsis
The choice of antibiotics in sepsis is largely determined by the source or focus of infection, the patient’s immunologic status, whether the infection is nosocomial or community acquired, and knowledge of the local microbiology and sensitivity patterns. Initial empirical anti-infective therapy should include one or more drugs that act against the likely pathogens and that penetrate into the presumed source of sepsis. However, whether the initial regimen in patients with severe sepsis and septic shock should include two or more antibiotics or an extended spectrum β-lactam antibiotic with the aim of treating all realistically possible microbial causes remains controversial.
In the guidelines for the management of severe sepsis of the SSC, initial combination therapy is recommended, and combination therapy is frequently used in clinical practice. Narrowing the spectrum of coverage after 3 to 5 days is recommended, except for infections caused by P. aeruginosa and infections among neutropenic patients for whom continued combination treatment is advised.
Several randomized trials of combination therapy versus monotherapy in serious infections, including endocarditis, gram-negative bacteremia, and neutropenic sepsis, and animal models have supported the possibility of clinically relevant antimicrobial synergism with appropriate combinations of antibiotics. In a recent large observational Spanish cohort study, Diaz-Martin and colleagues described the effect of empirical combination antimicrobial therapy in patients with severe sepsis and septic shock. Patients who received combination antimicrobial therapy experienced a 15% relative reduction in mortality and a 30% reduction in the odds of death when compared with patients who received single antibiotic therapy.
However, two separate meta-analyses have failed to demonstrate any consistent benefit with combination therapy of β-lactams and aminoglycosides in immunocompetent patients with sepsis, gram-negative bacteremia, or both.
Paul and colleagues performed a review and meta-analysis comparing β-lactam–aminoglycoside combination therapy with β-lactam monotherapy for severe infections in nonimmunocompromised patients with sepsis. In this analysis, a total of 69 randomized and quasirandomized trials were included, comprising 7863 patients, of which approximately 1000 had pneumonia. Paul and colleagues concluded there was no difference in all-cause fatality (RR, 0.97; 95% CI, 0.73 to 1.30) and that empirical evidence did not show the synergy effect when adding an aminoglycoside to a β-lactam in the clinical setting. In addition, nephrotoxicity was significantly less frequent with monotherapy (RR, 0.30; 95% CI, 0.23 to 0.39).
In 2004, Safdar and colleagues published a meta-analysis to determine whether a combination of two or more drugs would reduce mortality in patients with gram-negative bacteria. Their study included 17 studies, of which five were prospective cohorts, two were RCTs, and the rest were retrospective. Most studies used β-lactams or aminoglycosides alone and in combination. Overall, they did not observe a mortality benefit with combination therapy (OR, 0.96, 0.7 to 1.32). Several subgroup analyses were also performed to determine whether the findings would differ if trials were separated according to date of publication (i.e., before or after 1990, when more potent antimicrobials were made available) or study design (i.e., retrospective vs. prospective). Regardless of subset analyses, there remained no added benefit to combination therapy. However, in an analysis restricted to five studies of P. aeruginosa bacteremia, the summary OR was 0.5 (0.32 to 0.79; P <.007), suggesting a 50% relative reduction in mortality with the use of combination therapy. The investigators noted, though, that underlying populations in these studies varied considerably and that a sizable proportion of patients were immunocompromised, making it difficult to apply the results to the general population.
In contrast, a meta-regression study by Kumar et al. suggested that the beneficial effect of combination therapy may be restricted to critically ill patients with septic shock. Kumar and colleagues hypothesized that any beneficial effect of combination (i.e., two antibiotics of different antimicrobial classes active for the isolated pathogen) antimicrobial therapy on the mortality of life-threatening infection is restricted to patients with septic shock or otherwise high risk of death. This hypothesis was tested in a meta-regression study of 50 studies from which 62 evaluable datasets of varying monotherapy mortality were derived. Notably, Kumar and colleagues found the same absence of a significant benefit of combination therapy overall as did Paul and colleagues. However, combination therapy demonstrated a significant advantage over monotherapy when the rate of death/clinical failure exceeded 25% (pooled OR, 0.54; 95% CI, 0.45 to 0.66; P < .0001).
Another retrospective, propensity-matched, multicenter cohort study of 4662 patients with culture-positive bacterial septic shock, also by Kumar et al., demonstrated that combination therapy may decrease 28-day mortality (36.3% vs. 29.0%; HR, 0.77 [95% CI, 0.67 to 0.88]; P < .001) and hospital mortality (47.8% vs. 37.4%; OR, 0.69 [95% CI, 0.59 to 0.81]; P < .001). Combination therapy was defined as using two or more agents with different mechanisms of action for at least 24 hours after the onset of hypotension, or until death if the patient died in the first 24 hours, and the second agent had to be added within 24 hours of the first agent or within 24 hours of the onset of hypotension. To reach these conclusions, the authors used a complex, propensity-matched method, yielding 1223 matched pairs of patients for analysis. The use of combination therapy also was associated with increased ventilator-free and pressor/inotrope–free days and significant reductions in stay in the ICU. The beneficial effects of combination therapy in the study by Kumar et al. applied to both gram-positive and gram-negative infections, but these findings were restricted to patients treated with β-lactams in combination with aminoglycosides, fluoroquinolones, or macrolides/clindamycin. Carbapenems, extended-spectrum β-lactam or β-lactamase inhibitor combinations, and antipseudomonal cephalosporins, which tend to demonstrate optimal pharmacokinetic indices (with presumably maximal kill rates) for most septic shock pathogens, yielded the weakest evidence of benefit with combination therapy. Notably, the most potent β-lactams including carbapenems failed to exhibit evidence of combination therapy benefit. In this circumstance, the addition of a second drug may have little incremental benefit. In addition, in both groups, mortality was lowest if therapy was given rapidly, and the differences between monotherapy and combination therapy were minimized in the patients who received rapid initiation of therapy.
It is evident from this study that β-lactam/β-lactamase inhibitors (BL/BLIs) and carbapenems are often considered for the treatment of sepsis when the main suspected pathogens are gram-negative bacteria because of their broad spectrum of coverage. Shiber et al. conducted a systematic review and meta-analysis of RCTs that compared BL/BLIs with carbapenems for the treatment of sepsis. They found no differences between BL/BLIs and carbapenems in all-cause mortality (RR, 0.98; 95% CI, 0.79 to 1.20) or clinical failure at the end of treatment (RR, 0.99; 95% CI, 0.89 to 1.11). Subgroup analyses of patients more likely to have had infections caused by ESBL-producing bacteria did not reveal an advantage from using carbapenems. Adverse events requiring discontinuation were more common with BL/BLIs (RR, 1.36; 95% CI, 1.03 to 1.79), most probably related to diarrhea, which was significantly more common with BL/BLIs (RR, 1.46; 95% CI, 1.25 to 1.70). Seizures, vomiting, and Clostridium difficile –associated diarrhea (CDAD) were significantly more common with carbapenems. The RR of 0.29 (0.10 to 0.87) for CDAD denotes a 71% lower incidence with BL/BLIs, with 95% CIs ranging from a decrease of 90% to a decrease of 13%.
Finally, in the first randomized trial of its kind, 600 patients with severe sepsis or septic shock at 44 ICUs in Germany were randomized to receive either IV meropenem, 1 g every 8 hours, or meropenem and moxifloxacin, 400 mg per day. Antibiotics were recommended to be provided for 7 days at least, or up to 14 days at the clinician’s discretion. Primary outcome was Sequential Organ Failure Assessment (SOFA) score, with secondary outcomes of mortality at 28 and 90 days. There were no statistically significant differences in outcomes between groups. Use of combination antibiotic therapy appeared to decrease the emergence of resistant organisms, albeit to a small extent: eight patients in the meropenem monotherapy group had positive cultures for organisms resistant to meropenem, compared with one in the combination therapy group.
In summary, good quality observational data suggest that early empirical combination antibiotic therapy with two antibiotics of different mechanisms of action is associated with superior outcomes compared with monotherapy in the treatment of bacterial septic shock in patients who have a high baseline risk of mortality. However, beneficial effects of combination therapy may be limited to regimens that use less than maximally potent antimicrobials, such as extended penicillins (e.g., ampicillin, ticarcillin, pipercillin); semisynthetic penicillins (e.g., cloxacillin, oxacillin); and first-, second-, and third-generation (nonpseudomonal) cephalosporins. Agents with maximal potency in terms of T > MIC (the time the drug concentration remains above the minimum inhibitory concentration) for most septic shock pathogens (carbapenems, ticarcillin/clavulanate, piperacillin/tazobactam, ceftazidime) may not yield additional benefit with the addition of a supplemental antibiotic. The key issue may be the ability of combination therapy to augment bacterial clearance compared to monotherapy. If so, then it is likely that augmented bacterial clearance with combination therapy may only be clinically relevant when the β-lactam component of combination therapy is less than maximally potent. Monotherapy is recommended for patients who are not critically ill and at high risk of death. However, further study is clearly needed to definitively address under what circumstances combination therapy may be useful in sepsis and septic shock.
Intra-abdominal Infections
Most intra-abdominal infections (IAIs) are polymicrobial and most commonly involve enteric gram-negative bacilli. Health-care–associated IAIs, compared with community-acquired IAIs, are significantly more likely to involve resistant pathogens. In vitro susceptibility of organisms isolated from IAIs is documented by the Study for Monitoring Antimicrobial Resistance Trends (SMART), which is a surveillance program that monitors resistance patterns. Over the course of the SMART study, the five most commonly isolated gram-negative pathogens from IAIs were E. coli , K. pneumoniae , P. aeruginosa . Enterobacter cloacae , and Proteus mirabilis. The incidence of β-lactamase production was 8.8% and 8.9% for E. coli and K. pneumoniae , respectively. Overall, the most active antimicrobials were amikacin, piperacillin-tazobactam, imipenem, and ertapenem, although β-lactamase production reduced the activity of most agents. Among the ESBL-producing bacteria, the carbapenems retained their activity better than other antimicrobials.
Once adequate source control is obtained, appropriate initial antimicrobial therapy heavily influences outcome in complicated IAIs, as with other severe infections. Evidence-based guidelines regarding selection of antimicrobial therapy for IAIs were formulated by the Surgical Infection Society, the IDSA, the American Society for Microbiology, and the Society of Infectious Disease Pharmacists. In the guidelines, the use of either single-drug regimens or combination therapy is recommended. Although it is stated in the guidelines that “antibiotic therapy for such (health-care-associated) infections may require the use of multi-drug regimens (e.g., an aminoglycoside or quinolone or a carbapenem and vancomycin),” no specific recommendations are made regarding the use of combination therapy. Alternative guidelines also exist that take into account newer antimicrobials and the treatment of resistant gram-positive and gram-negative infections.
Empirical therapy of community-acquired IAIs should include acylaminopenicillin/BLI or ertapenem or other carbapenems (imipenem/cilastatin, meropenem, doripenem). Alternatively, combinations of metronidazole with group cephalosporins, ciprofloxacin, levofloxacin, or moxifloxacin monotherapy are also appropriate. Antibiotics covering the enterococci are usually not required in community-acquired IAIs. Antibiotic treatment of enterococci is recommended in postoperative IAI or seriously ill patients. Microbial causes of postoperative peritonitis tend to be multidrug resistant, including enterococci (including vancomycin-resistant enterococci [VRE]), resistant gram-negative organisms (ESBL or AmpC or carbapenemase-producer), MRSA, and Candida species. Appropriate agents may be carbapenems, tigecycline, piperacillin/tazobactam, or moxifloxacin depending on microbial findings. Antifungal treatment is recommended for proven fungal infections (see later).
Several combination regimens have been investigated for treatment of IAIs, including aminoglycoside-based, cephalosporin-based, or quinolone-based regimens. Some, but not all, of the trials included patients in severe sepsis or septic shock. The systematic review by Bochud and colleagues included five trials that evaluated the use of combination therapy versus monotherapy for the empirical treatment of abdominal sepsis. In all five trials, there was no significant mortality difference between the two treatment arms. Subsequently, two RCTs have examined the use of combination therapy in abdominal infections. Yellin and colleagues found that success rates of ertapenem compared with combination therapy using ceftriaxone and metronidazole were similar at 83% (22/29) and 77% (24/31) in the ertapenem and comparator groups, respectively. Solomkin and colleagues compared moxifloxacin monotherapy with ceftriaxone plus metronidazole in patients with complicated community-origin IAI. Moxifloxacin was noninferior to ceftriaxone plus metronidazole in terms of clinical response at test-of-cure in the per protocol population (clinical cure, 90.2% for moxifloxacin vs. 96.5% for ceftriaxone/metronidazole; 95% CI of the difference, −11.7 to −1.7). However, the patients included in these trials were not critically ill. In the study by Yellin and colleagues, 94% of patients in each treatment arm had APACHE (Acute Physiology and Chronic Health Evaluation) scores of 14 or lower, and in the study by Solomkin and colleagues, all patients had community-acquired abdominal infections, none of whom were in severe sepsis or shock. A Cochrane review of antibiotics in secondary peritonitis showed no difference in all-cause mortality between aminoglycosides plus anti-anaerobes and other regimens (OR, 2.03; 95% CI, 0.88 to 4.71), nor was there evidence of an increase in adverse events between the groups (OR, 1.76; 95% CI, 0.87 to 3.53). However, there was evidence of a faster cure rate in the other regimen group, and this resulted in a shorter length of stay. On the basis of limited data, the use of combination therapy in abdominal sepsis does not appear to be advantageous compared with single-drug therapy for as long as the initial antimicrobial drug is appropriate.
Empirical Antifungal Therapy
Candida infections are underrecognized. Culture mechanisms lack sensitivity. This would suggest a possible role for empirical antifungals, particularly in patients with recent exposure to broad-spectrum antibiotics or immunosuppression. However, the SSC recommends against the routine use of empirical antifungals because only a small proportion of septic patients have fungal infections (5% of cases), although this is likely to rise. In the EPIC (Extended Prevalence of Infection in Intensive Care) II study comprising 7087 infected ICU patients in 75 countries, Candida spp. was the third most frequent organism cultured, accounting for 19% of all isolates, although it is unclear whether these were the organisms responsible for the sepsis.
A large retrospective study identified delay in administration of antifungal agents as a predictor of hospital mortality in patients subsequently found to have positive cultures for Candida spp. With the relatively high morbidity associated with the use of antifungals, it would seem reasonable not to recommend their routine use. However, it is very likely that the timing of antifungal therapy in severe infection is just as critical as that of antibiotic therapy. In high-risk patients, a high index of suspicion for primary or secondary fungal infection and a low threshold for the use of antifungal agents are required.
Fungi are more prevalent as isolates in patients with secondary or tertiary peritonitis, with Candida spp. identified in up to 20% of patients with gastrointestinal tract perforation. Risk factors include fecal soiling of the peritoneum, recurrent gastrointestinal perforation, immunosuppressive therapy, inflammatory diseases, and status after transplant. These patients have a high risk of mortality, and some case series suggest benefit from the empirical addition of agents with activity against Candida spp.
The IDSA has produced guidelines recommending the use of amphotericin B or fluconazole in patients with Candida peritonitis for a period of 2 to 3 weeks as a supplement to surgical drainage. However, these guidelines did not offer guidance on the use of prophylactic antifungal agents in patients with peritonitis with risk factors. However, the notion of previous Candida colonization is a strong argument in favor of early initiation of antifungal therapy. The increase in frequency of Candida glabrata may prompt some units to use echinocandins in preference to azole agents in these high-risk patients. In patients with septic shock and suspected Candida species, it seems reasonable to promptly initiate treatment with an echinocandin and consider de-escalation as soon as identification and susceptibility results are available.

Full access? Get Clinical Tree
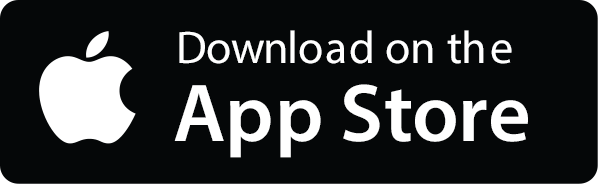
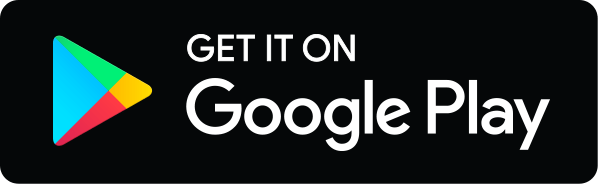