The term acute respiratory distress syndrome (ARDS) describes acute inflammatory lung injury caused by a wide variety of insults, resulting in severe hypoxemic respiratory failure. ARDS has been recognized as a clinical entity in adults for 50 years and affects more than 100,000 adults in the United States each year. Reported mortality rates in observational studies persist at between 30% and 60%—depending on severity of illness and organ function. In survivors, long-term disability is a major problem. Data accumulated since the early 1990s have confirmed that a careful approach to mechanical ventilation can significantly improve outcomes and shorten the duration of illness. This chapter reviews current mechanical ventilation strategies and proven supportive interventions in ARDS.
Strategies for the Management of ARDS
Although ARDS arises from a wide variety of injuries and differing pathophysiology, mechanical ventilation is usually required to sustain life. Poor application of mechanical ventilation may worsen lung injury and increase mortality. There is no specific therapy for ARDS beyond treating the underlying disease process (e.g., infection) and supportive care. Therefore the focus of respiratory support by mechanical ventilation in patients with ARDS is to provide acceptable gas exchange while simultaneously minimizing further injury to the lung.
Ventilator-Induced Lung Injury
Until the 1990s mechanical ventilation (MV) strategy involved the use of relatively high tidal volumes (to reduce atelectasis) in the range of 10 to 15 mL per kilogram of body weight, which was usually estimated. Depending on local preference this could be delivered by volume assist-control, synchronized intermittent mechanical ventilation, or pressure assist-control. The application of positive end-expiratory pressure (PEEP) was variable, and there were periods where both high and low levels of PEEP were fashionable. MV as a therapy targeted normalized blood gases. An end-inspiratory airway pressure of less than 50 cm H 2 O was considered acceptable in the absence of pneumothorax or surgical emphysema.
In the 1970s, Webb and Tierney published data demonstrating that high peak inflation pressures severely damaged the lung in rats, revealing the existence of ventilator-induced lung injury (VILI), a process that could be attenuated by judicious use of PEEP. In the 1980s computed tomography (CT) showed that consolidation in lungs affected by ARDS was not as uniform as suggested by the plain radiograph, and that a multilayered topography of pathology was present ( Fig. 30-1 ). Effectively the lung is divided into three zones: a nonrecruitable zone, in the bases, an injured but recruitable midzone, and a spared though potentially overdistended zone in the apices. In the acutely injured lung, less than 50% of the lung may contribute to gas exchange. The anterior apical segments of the lungs are relatively disease free and, being more compliant, are vulnerable to stretch injury. These observations led to the description of the “baby lung” as a functional entity. The concept conveniently illustrates that healthy regions of lung parenchyma bear more stress and strain than the collapsed and consolidated regions. Repeated overdistension of this smaller and vulnerable lung tissue during tidal ventilation results in VILI. Key developments in the field of ARDS ventilation are summarized in Table 30-1 .

Study | Key Development |
---|---|
Ashbaugh et al. (1967) | The “original description” of ARDS suggesting a common pathway of lung injury irrespective of the initial injury. |
Webb and Tierney (1974) | An animal study illustrating the relationship among inflation pressure, PEEP, lung histology, and gas exchange. Confirmed the existence of ventilator-induced lung injury. |
Gattinoni et al. (1987) | The use of CT scanning in ARDS patients showed the heterogenicity of the lung injury. |
Dreyfuss et al. (1988) | Illustrated that inflation volume in mechanical ventilation may cause greater damage than airway pressure in itself. |
Hickling et al. (1990) | Advocated adopting pressure-limited ventilation and permissive hypercapnia strategies in ARDS management. |
Bernard et al. (1994) | American European consensus conference on ARDS. Publication of the current definition of ARDS and ALI. |
Tremblay et al. (1997) | Introduction of the concept of biotrauma: High tidal volume ventilation without PEEP releases proinflammatory cytokines from lung tissue. |
Amato et al. (1998) | Small RCT showing a decrease in mortality associated with low tidal volume ventilation and PEEP. |
NIH/ARDSnet (2000) | Large trial confirming reduced mortality in ARDS patients ventilated with low tidal volumes. It concluded the debate raised by earlier conflicting smaller trials. |
Modern mechanical ventilation strategy is as focused to preventing VILI as it is to normalization of blood gases. This involves the following:
- •
Lung protection: Ventilation with low tidal volume (V t ) and low airway plateau pressure (P plat; surrogate of alveolar pressure), using “permissive hypercapnia” where necessary.
- •
Lung recruitment: The use of appropriate levels of end-expiratory pressures (PEEP) to recruit collapsed alveolar units and avoid further injury to the lung associated with high alveolar volume swings (volutrauma) and shearing injury associated with recurrent opening and closing of collapsed lung units (atelectrauma). This general concept is known as the “open lung” approach to mechanical ventilation. Application of very high levels of PEEP for short periods of time has been proposed as a method for achieving further recruitment (recruitment maneuvers [RMs]).
“Low Stretch” Approach
Mechanical ventilation with tidal volumes restricted to 6 mL/kg predicted body weight, resulting in limited transpulmonary pressure, is associated with a decrease in mortality compared with higher tidal volumes and airway pressures.
Clinical research studies conducted in the mid-1990s into VILI by Brochard et al., Stewart et al., and Brower et al. were underpowered, suffered design flaws, and failed to deliver anticipated outcome benefits for low stretch strategies. In an “open lung” study, Amato did, however, demonstrate staggering outcome benefits (mortality rate 30% intervention group compared with 71% conventional group, P < .001), albeit with higher than expected mortality in the control group. The NIH/ARDSnet ARMA trial, published in 2000, eventually resolved the issue. The study overcame the problems of the previous trials by comparing patients who received tidal volumes of 6 mL/kg and plateau pressures (P plat ) less than 30 cm H 2 O with 12 mL/kg and P plat <50 cm H 2 O. Patients received volume assist-control ventilation, and oxygen therapy and PEEP were adjusted with a stepped protocol. Tidal volume was based on predicted (derived from height), rather than actual, body weight.
The intervention group had a mortality rate of 31%, compared with 39.8% in the high tidal volume group, absolute risk reduction (ARR) 8.8%, number needed to treat (NNT) 11 ( P < .05). Volumes could be adjusted between 4 and 8 mL/kg to maintain the plateau pressure below 30 cm H 2 O, and hypercapnia could ensue, although a pH above 7.30 was targeted. A number of issues remain unresolved from this study, in particular: (1) As minute ventilation was the same in each group, did the patients in the intervention group have more auto-PEEP as a consequence of a more rapid respiratory rate? (2) Is there a difference between 6 and 8 mL/kg if the P plat is <30 cm H 2 O?
Table 30-2 summarizes the data from the original low stretch studies. Although many studies have been performed subsequently, the ARMA trial remains the only large multicenter randomized controlled trial that has demonstrated a mortality benefit from a specific mechanical ventilator maneuver in ARDS.
Authors | n | Tidal Volume (mL/kg PBW) | PEEP (cm H 2 O) | Mortality (%) | P Value |
---|---|---|---|---|---|
Amato et al. (1998) | |||||
Conventional | 24 | 12 | 8.7 ± 0.4 | 72 | –– |
Protective | 29 | <6 | 16.4 ± 0.4 | 38 | <.001 |
Stewart et al. (1998) | |||||
Conventional | 60 | 10.7 ± 1.4 | 7.2 ± 3.3 | 47 | –– |
Protective | 60 | 7.0 ± 0.7 | 8.6 ± 3.0 | 50 | n.s. |
Brochard et al. (1998) | |||||
Conventional | 58 | 10.3 ± 7.7 | 10.7 ± 2.3 | 38 | –– |
Protective | 58 | 7.1 ± 1.3 | 10.7 ± 2.9 | 47 | n.s. |
Brower et al. (1999) | |||||
Conventional | 26 | 10.2 ± 0.1 | –– | 46 | –– |
Protective | 26 | 7.3 ± 0.1 | –– | 50 | n.s. |
ARDS network (2000) | |||||
Conventional | 429 | 11.8 ± 0.8 | 8.6 ± 3.6 | 40 | –– |
Protective | 432 | 6.2 ± 0.9 | 9.4 ± 3.6 | 31 | 0.007 |
In conclusion, the current standard for mechanical ventilation care for most patients with ARDS involves setting tidal volumes at <6 mL/kg and plateau pressure below 30 cm H 2 O.
Open Lung Approach
Four mechanisms have been proposed to explain the beneficial effect of PEEP in the injured lung: (1) increased end-expiratory lung volume, thereby improving gas exchange; (2) redistribution of alveolar lung water; (3) improved ventilation-perfusion matching; and (4) stabilization of recruited lung. The use of PEEP is also thought to be protective in preventing the cyclical collapse of alveoli with tidal ventilation, splinting open alveoli throughout the respiratory cycle and avoiding atelectrauma. Although there is general agreement among experts that some amount of PEEP is beneficial, an assertion supported by observational data, exactly what level of PEEP should be used has remained a contentious issue for decades.
Open lung studies by Amato et al. and Villar et al. used the lower inflection point of the volume–pressure curve to estimate the critical opening pressure of the lung; this point was used to determine the best PEEP ( Table 30-3 ). Although higher PEEP was administered in their study groups than in their controls (13.2 vs. 9.3 cm H 2 O, and 14.1 vs. 9.0 cm H 2 O, respectively), there was also significant variance in tidal volumes, and outcome benefits may have derived from low stretch rather than higher PEEP. Three large multicenter randomized controlled trials (RCTs) addressed the question of high versus low PEEP in the setting of a low stretch ventilation strategy ( Table 30-3 ). In both the ALVEOLI (Assessment of Low Tidal Volume and Elevated End-Expiratory Volume to Obviate Lung Injury) trial and the Lung Open Ventilation Study (LOVS), PEEP was determined according to higher and lower PEEP-F io 2 (fraction of inspired oxygen) tables, whereas the ExPress trial compared lower levels of PEEP (5 to 9 cm H 2 O) with higher levels set to achieve a plateau pressure of 28 cm H 2 O. In keeping with the original open-lung approach, the LOVS trial also used RMs.
a. Summary of Study Patients and Interventions | ||||||
---|---|---|---|---|---|---|
Study | n | Patients | PEEP | Mode | RMs | P plat |
ALVEOLI | 549 | Pa o 2 /F io 2 <300 | ||||
Open lung | High (PEEP/F io 2 chart) | AC | No | ≤30 cm H 2 O | ||
Control | low (PEEP/F io 2 chart) | AC | No | ≤30 cm H 2 O | ||
LOVS | 983 | Pa o 2 /F io 2 <250 | ||||
Open lung | High (PEEP/F io 2 chart) | PC | Yes | ≤40 cm H 2 O | ||
Control | low (PEEP/F io 2 chart) | AC | No | ≤30 cm H 2 O | ||
ExPress | 767 | Pa o 2 /Fio 2 <300 | ||||
Open lung | To keep P plat 30 cm H 2 O | AC | No | 28-32 cm H 2 O | ||
Control | 5-12 cm H 2 O | AC | No | ≤32 cm H 2 O |
b. Summary of Methodological Features | |||||
---|---|---|---|---|---|
Random | Baseline | Similarity in Other | |||
Study | Intentionization | Stopped Differences | Aspects of Care | To Treat | Early |
ALVEOLI | Central | Age, by 5.5 years | V t 6 mL/kg PBW | Yes | Yes |
Automated | (Lower control group) | Weaning | |||
LOVS | Central | Age, by 2 years | V t 6 mL/kg PBW | Yes | No |
Automated | (Higher control group) | Weaning | |||
ExPress | Central | None | V t 6 mL/kg PBW | Yes | Yes |
Automated | Weaning |

Full access? Get Clinical Tree
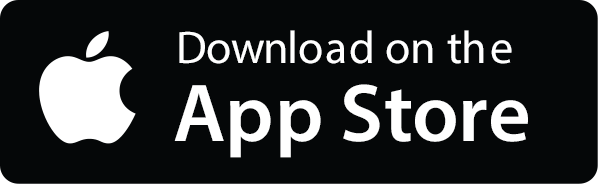
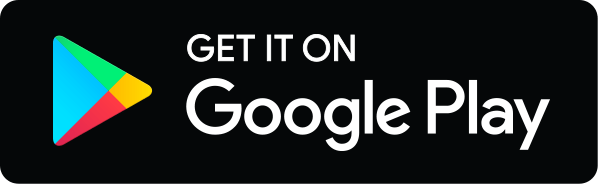
