Ventilator-Supported Speech: Introduction
Under normal circumstances, the respiratory system supplies the aeromechanical drive that allows the vocal folds, tongue, lips, and other structures to create the sounds of speech. Although a simplification, this drive can be understood as the tracheal pressure. Usually this pressure is exquisitely and actively controlled by muscles of the chest wall. When speech is produced with ventilator support, however, the ventilator and the respiratory system must work together to produce the pressure that drives speech production. In most cases, this pressure is markedly different from that of normal speech production. As a result, the act of speaking can be challenging for patients, and they often require assistance from their pulmonologists, respiratory therapists, and speech-language pathologists. The views presented here are that patients should be enabled to speak whenever possible, and that ventilator-supported speech can often be improved by using interventions such as those described in this chapter.
The Importance of Speech
Communication is critical to maintaining good quality of life. Communication is the key to being able to express needs, participate in social activities, and retain control over important life decisions.1–5 It can be especially important in intensive care or during end-of-life care.6,7 There are many ways to communicate, but of these, speech is the fastest and most convenient. It works in the dark and across telephone lines, and it conveys meaning and emotions through words and tone of voice (i.e., pitch, loudness, quality, and timing).
Patients who are ventilator-supported often complain of speech problems, especially unwanted pauses and inadequate loudness.8 This is illustrated in the following quotation,9 which comes from a patient who was asked, “Do you have any problems with your speech?” Note that the pauses between phrases were 3 to 4 seconds (• = 1 second), and that some words were produced without voice (shown in parentheses). To appreciate the severity of the speech problem, this quotation should be read aloud with timed pauses:
“Yes •••• Um, getting cut (off) •••• people interfering with me ••• trying to finish my sentences (for me) •••• that’s the most frustrating (part).”
Fortunately, it is nearly always possible to improve ventilator-supported speech. The following quotation comes from the same patient, after she received speech interventions. Pauses were reduced to approximately 1 second, and the amount of speech per breath increased by approximately 50%.
“Since I’ve been able to talk better • I’ve par- participated in talking with more people and • and in conversation instead of just doing the listening • and talking as little as possible • I’ve joined in and, and • I don’t know, I’ve just been part of a group more • Very few people try to finish my sentences now and • second guess what I’m going to say • It’s, it’s helped a lot.”
Fundamentals of Speech Breathing
Speech comprises the tones, hisses, pops, and buzzes that are the acoustic representation of language.10 Speech is usually produced by the coordinated efforts of more than 100 muscles spanning the chest wall, larynx, and upper airway structures (pharynx, velum, jaw, lips, and tongue). Speech breathing is the process by which the driving forces are supplied to downstream structures to generate speech, and it is tailored to simultaneously serve speech-related functions and meet gas exchange requirements.11
The normal speech breathing cycle has an inspiratory phase ranging from 0.5 to 0.7 second.12–16 Inspirations during speaking are so short that the pauses they create are hardly noticeable. The longer, more variable expiratory phase of the cycle is the speaking phase. Its duration averages 3 to 5 seconds, but varies substantially as a function of linguistic factors, cognitive variables, mechanical constraints, and ventilatory needs.13,14,16–18 Speech is usually produced throughout most of the expiratory phase, although nonspeech expirations are likely to occur in senescent subjects,19 with high cognitive-linguistic demands,20 and under conditions of elevated ventilatory drive.16,21,22 The tidal volumes used in speech breathing are typically about twice as large as those of resting tidal breathing.23
The normal speech breathing cycle has a characteristic tracheal pressure profile. During inspiration, pressure is substantially and briefly negative, and during expiration (speaking), it is moderately positive and relatively steady. For speech of conversational loudness, pressure is generally in the range of 5 to 10 cm H2O.24,25 Pressure usually remains relatively constant throughout expiration so that loudness and voice quality remain relatively constant.26,27 An increase in pressure generally causes an increase in loudness. To produce voiced speech sounds (such as vowels), the vocal folds must oscillate, which requires a minimum pressure of approximately 2 cm H2O.28,29 When voiced sounds are produced with a constricted oral airway (such as the plosive /d/ or the fricative /v/), slightly higher pressures are needed.
The pressures of normal speech breathing are controlled actively throughout the cycle by muscles of the chest wall.27 Inspiration is driven by the diaphragm (with activation of other muscles that serve to stabilize the chest wall), and expiration (speaking) is driven by both expiratory rib cage and abdominal muscular pressures (with the latter predominating) when in upright body positions, and by expiratory rib cage muscular pressure alone when in the supine body position. These muscular pressures are usually supplemented by positive recoil pressure, because most conversational speech is produced above the resting expiratory level. On rare occasions, when speech is initiated at a very large lung volume (i.e., near total lung capacity), positive recoil force may be so great that inspiratory muscular pressure must be called into play to counteract (i.e., “brake”) the high positive pressure.
A Framework for Understanding Ventilator-Supported Speech
During ventilator-supported speech breathing, tracheal pressure reflects the combined contributions of the patient’s prevailing respiratory recoil pressure, active muscular pressures (if the patient is able to activate chest wall muscles), and inspiratory and/or expiratory drive from the ventilator, among other factors. A useful framework for understanding, evaluating, and managing ventilator-supported speech is to relate speech to tracheal pressure.9,11,30
Speech with Invasive Positive-Pressure Ventilation
Invasive positive-pressure ventilation (InPPV) is used here to mean intermittent positive-pressure ventilation via tracheostomy. Because an endotracheal tube prohibits vocalizing and can damage the vocal folds, speech is one of several factors to consider when deciding whether and when to perform a tracheotomy (see Chapter 40). Speaking with InPPV is substantially different from speaking with other forms of ventilation, but it can be very successful. The primary differences stem from the fact that the ventilator-delivered air enters below the larynx.
For a patient to speak, the first and most critical step is to configure the tracheostomy tube so that flow (and thus pressure) can reach the larynx. This means that the cuff on the tube must be deflated (and/or the tube must contain a fenestration). If cuff deflation or use of a fenestrated tube is deemed inadvisable, alternative forms of speech or communication must be sought (see Chapter 40 and “Alternatives to Ventilator-Supported Speech”).
Cuff deflation is feasible in most chronically ventilated patients31,32 and in acutely ventilated patients who are otherwise stable. Indeed, in some patients, the cuff can be deflated and speech training initiated on the first day after the tracheotomy has been performed. Unfortunately, cuff deflation and speech training are often delayed (or not performed at all) because of unfamiliarity with the procedure, concerns about aspiration, and fear of causing hypoventilation and hypoxemia. Hypoventilation and hypoxemia can be avoided by increasing the ventilator-delivered tidal volume. In many stable patients, the cuff can remain deflated all day (and inflated only for sleep). In less-stable patients, cuff deflation may need to be brief (to allow communication with family members or caregivers) and performed only in the presence of a pulmonologist or respiratory therapist (see Clinical Scenario 1). It is sometimes helpful to deflate the cuff only partially in patients who tend to lose too much of the inspired tidal volume through an open larynx. The recommended procedure for initial cuff deflation is described in “Important Cautions”.
Mrs. Z was a 52-year-old woman with breast cancer that had metastasized to her lungs, causing her to be very short of breath and unable to breathe on her own. She obtained relief from assisted ventilation via a tracheostomy and could not be weaned from the ventilator. She was frustrated by not being able to speak to her caregivers, husband, and daughters about the nature and intensity of the care she wished to receive and about her impending death. Communication by writing was tedious. She was advised that the cuff of her tracheostomy tube could be deflated so that she could speak, but that she would likely experience periods of breathlessness at first while ventilator adjustments were being made. She was very fearful of this, but with encouragement and reassurance the procedure was successful. For several minutes at a time, with only slight shortness of breath, she was able to speak and communicate her wishes to her family. She went home on the ventilator and died peacefully there. |
After cuff deflation, the patient’s voice should be assessed. If voice problems are noted, the resistance to “bypass” gas flow around the cuff should be estimated to determine if it is sufficiently low to allow good vocalizing. Bypass resistance can be estimated by blocking expiratory flow where it exits the ventilator and observing tracheal pressure during expiration. When the resistive pressure drop is less than 5 cm H2O, cuff deflation is generally successful, but when pressure exceeds 10 cm H2O, cuff deflation often fails. Bypass resistance can usually be reduced by downsizing the tracheostomy tube. If the patient still cannot vocalize, the upper airway, larynx, and upper trachea should be viewed via nasendoscopy to rule out conditions such as excessive secretions, laryngeal edema, granulation tissue, tracheal scar formation, or vocal fold paralysis.
Figure 56-1 illustrates the flow routes for inspiration and expiration with an inflated cuff (closed system) and deflated cuff (open system). When the cuff is inflated, no flow reaches the larynx. When the cuff is deflated, flow reaches the larynx during inspiration and expiration, making it theoretically possible to produce speech during both phases of the ventilator’s cycle. The ventilator’s settings, however, are strong determinants of when within the cycle speech can be produced. Speech produced with InPPV is often plagued by long pauses, short phrases, variable loudness, and variable voice quality.30,33,34 These speech features are most easily explained by relating them to the tracheal pressure.
Figure 56-1
Inflated and deflated tracheostomy tube cuff during inspiration (left) and expiration (right). When the cuff is inflated, no flow reaches the larynx, and when the cuff is deflated, flow reaches the larynx during both inspiration and expiration. (Adapted and used, with permission, from Hixon and Hoit.11)
Figure 56-2 illustrates tracheal pressure with volume-controlled ventilation compared with that of normal speech production. During normal speaking pressure is low and constant. This steady pressure allows vocal fold oscillations to be relatively constant in amplitude and waveform, translating perceptually to relatively constant loudness and voice quality. Also, pressure remains above the vocalizing threshold throughout expiration.
Figure 56-2
Tracheal pressure during volume-controlled InPPV and during normal speech production. The dashed line shows the minimum pressure needed for vocalizing. (Used, with permission, from Hixon and Hoit.11)
By contrast, during volume-controlled InPPV pressure rises quickly during inspiration, falls sharply when the expiration valve opens, and then remains below the vocalizing threshold for a substantial portion of the cycle. The rapidly changing pressure is the primary cause of loudness and voice quality problems, and the periods of low pressure (below the vocalizing threshold) explain the long pauses and the short phrases.
Abnormal magnitude and time course of tracheal pressure are at the root of the speech problems associated with volume-controlled InPPV. Therefore, the unifying principle for improving speech is to adjust the ventilator so as to generate a pressure that is better suited for speech production, while accommodating the patient’s respiratory needs and comfort. Ventilator adjustments to improve speech are designed to (a) increase the portion of the cycle during which pressure remains above the vocalizing threshold (i.e., at least 2 cm H2O), and (b) reduce the rate and magnitude of pressure changes during the period in which speech is produced. Many adjustments are possible, and the optimal combination for a given patient must be determined empirically. The success of this approach has been well documented in patients with spinal cord injury and neuromuscular disease,30,34–36 and is likely to be effective in others. Examples of ventilator adjustments and their predicted influences on speech are given below.
One of the easiest and most successful adjustment combinations is lengthened inspiratory time and the application of positive end-expiratory pressure (PEEP). When inspiratory time is lengthened, more speech can be produced during the inspiratory phase of the ventilator’s cycle, because pressure is above the vocalizing threshold for a longer period. In general, as inspiratory time increases, the amount of speech produced during inspiration increases. Also, because the rate of pressure change is more gradual when inspiration is prolonged, loudness and voice quality variability may be reduced. The addition of PEEP extends speaking time during the expiratory phase by adding resistance to the ventilator’s expiratory line, which keeps pressure above the vocalizing threshold longer. In most cases, pressure will eventually fall to zero secondary to flow through the larynx.
Sometimes it is physiologically appropriate to also reduce the ventilator-delivered tidal volume in patients whose tidal volumes are too large. When tidal volume is reduced, peak pressure is lowered, which helps to smooth out loudness and voice quality. Although patients are usually uncomfortable when tidal volume is reduced, they are often comfortable if PEEP is added first.
An example of the effect of these adjustments on tracheal pressure and the acoustic speech signal is shown in Figure 56-3 as a comparison of a patient’s “usual” and “best” ventilator settings. Although the ventilator adjustments resulted in only subtle changes in the pressure waveform, the changes in speech were substantial and easily perceptible to listeners.30

Full access? Get Clinical Tree
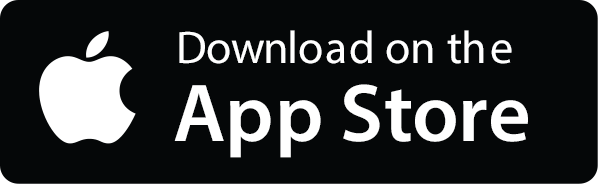
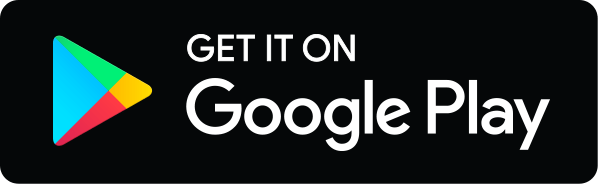
