Returning to the case study mentioned at the beginning of this article, the patient presented initially received PPV and then was extubated to spontaneous ventilation. Thus, the patient’s already depressed left ventricle then had to generate a higher pressure to obtain the same systemic output. However, the patient’s left ventricle was unable to maintain that increase in afterload and poor cardiac output as demonstrated by hypotension and poor peripheral perfusion developed. Clearly in this case, the effect of PPV on the left ventricle was augmented cardiac output, caused by an increased mean intrathoracic pressure, via decreased LV afterload.
Unlike the effects of PPV on LV afterload, the effects of PPV on LV preload beyond the concept of thoracic pump augmentation can be somewhat variable because the effect of PPV on LV preload is generally based on the patient’s intravascular volume status and on what has happened on the right side of the heart. If PPV is decreasing venous return to the right side of the heart, it is obviously going to decrease LV preload as well. The effects of this change in LV preload may be good or bad depending on the patient’s place on the Frank-Starling curve. Thus, LV preload is complex as it can be largely affected by ventricular interdependence.
The interplay of intrathoracic pressure and cardiac function can be very complex, especially in critically ill patients. As illustrated in Figure 2, the interactions between mechanical ventilation and cardiac function can be boiled down to the following three components. Increased intrathoracic pressure (1) will always decrease RV preload, (2) may or may not increase RV afterload, and (3) will decrease LV afterload. Again, whether the physiology positively or negatively affects an individual patient is largely determined by the patient’s overall cardiorespiratory status and degree of illness.
Figure 2. Increased Intrathoracic Pressure
Cardiorespiratory Interactions: The Lungs
When the lungs are atelectatic, the large pulmonary vessels become tortuous and the resistance in the large vessels is elevated. As the lung is opened, these large vessels straighten and the resistance decreases. As the lung expands to normal lung volume and then becomes overdistended, the resistance in the large vessels is minimal.
When the lung is collapsed, the resistance in the small vessels is very low. However, as the lung volume increases and the lungs overdistend, the resultant overdistended alveoli compress the surrounding capillaries, causing an elevation in PVR and a subsequent increase in RV afterload.
The total PVR will be highest at the extremes of alveolar overdistention and lung collapse and lowest at an optimal lung volume, which is approximated by functional residual capacity. The clinician’s goals are to maintain an optimal lung volume and to avoid overdistention and collapse. Optimal lung volume will minimize PVR.
Looking at PVR from a physiologic point of view, an animal trial studied the effect of alkalosis on hypoxia-induced pulmonary vasoconstriction in 13 neonatal lambs.1 As expected, PVR increased with hypoxia. Both respiratory and metabolic alkalosis similarly decreased PVR. These results demonstrate that PVR is truly an effect of acid–base balance, regardless of whether alkalosis is respiratory or metabolic in origin. Of course, this point does not take into account the potentially adverse cerebral perfusion effects of hyperventilation. Although the study focused on neonates, its findings may be applicable to all patient populations.
Looking at the physiology in more detail, another study analyzed the effects of PaCO2 on PVR by decreasing PaCO2 but buffering to a pH of 7.4.2 This in essence removes the pH effect and focuses purely on the carbon dioxide (CO2) effect. The authors found that hyperventilation, or decreasing CO2, does not affect PVR. What truly decreases PVR is the acid–base balance (ie, alkalosis).
Continuing to discuss this study by Malik et al, but moving from its application in neonatology to single-ventricle physiology allows for examination of the effects of PaCO2 on PVR in congenital heart disease. If the patient is hypoventilated, allowing the PaCO2 to increase while maintaining a constant pH will increase PVR. Thus, hypercapnia increases PVR at a normal pH, but hypocapnia does not decrease PVR if pH is buffered to 7.4.
From the pulmonary vasculature point of view and cardiorespiratory interactions, the goal of the clinician should be to optimize lung volume (ie, avoid overexpansion and atelectasis) to keep PVR to a minimum. As an aside, it is important to remember that inhaled gases, such as oxygen and nitric oxide, provide an opportunity to modify PVR as well.
Conventional Ventilation
Conventional ventilation—specifically with regard to lung volume and patient–ventilator synchrony—plays a key role in optimizing O2 supply and demand. Regarding conventional ventilation, the dominant effect of the ventilator is always mean airway pressure. Any effects caused by phasic changes (ie, Δ P) are minor and are always offset by the mean airway pressure.
The predominant cardiac effect caused by overdistention is increased PVR, which will increase RV afterload and potentially decrease cardiac output. A study of overdistention and PVR shows this to be true, even though the study involved tidal volumes that may not necessarily be clinically applicable today as it was reported in 1998. The prospective, randomized controlled laboratory trial3 involved ventilating swine with tidal volumes of 10, 15, and 20 mL/kg and PEEP settings of 5 and 10 cm H2O. As tidal volume increased, so did PVR. When the PEEP is increased to 10 cm H2O, PVR dramatically increases, as illustrated in Figure 3. As the lungs are grossly overdistended, PVR increases significantly. As RV afterload increases, cardiac output plummets, as illustrated in Figure 4. Even though this physiology was studied in a laboratory animal setting, the physiology is applicable to clinical situations.
There is often not much that can be done to optimize oxygen consumption. One situation in which the clinician can help balance the oxygen supply-to-demand ratio by minimizing consumption is to correct patient–ventilator synchrony, if present. A patient whose breathing is asynchronous with the ventilator will be uncomfortable and agitated, resulting in an increased work of breathing and excessive oxygen consumption. If the patient’s ventilator asynchrony can be minimized, oxygen consumption will decrease, and cardiorespiratory interactions will improve.
Oxygen Delivery
A consensus conference on tissue hypoxia4 published the following recommendations in 1996:
- Achievement of normal hemodynamics is essential
- Initial management should ensure adequate intravascular volume
- There is no clear evidence favoring colloid versus crystalloid solutions for volume resuscitation
Figure 3. Overdistention and PVR.
Figure 4. Overdistention and CO.
Regarding the second recommendation, if the patient is intravascularly deplete, PPV will worsen RV preload. Therefore, these patients may need augmentation of preload to minimize the effects of PPV on RV preload. This happens frequently in critically ill ICU patients. When this patient population is transitioned from conventional ventilation to high-frequency oscillatory ventilation and the mean airway pressure is significantly increased, RV preload is depressed and global cardiac output is decreased. This most commonly is seen at the bedside by a decrease in blood pressure. When preload is augmented (usually requiring only about 5 mL/kg of intravenous fluid), patient condition improves. Of note, although debate continues today, there is no clear evidence favoring colloid over crystalloid fluids.
The consensus conference concluded that aggressive attempts to increase oxygen delivery to supranormal values in all critically ill patients are unwarranted. More is not necessarily better; most therapies designed to increase oxygen delivery carry some degree of potential toxicity. The panel also suggested that the therapeutic approach must be individualized,with close monitoring of markers of appropriate oxygen delivery.
Some clinicians believe that using vasoactive agents may adversely affect LV afterload, but it is important to acknowledge that such agents play an important role in the treatment of these patients. Increased myocardial oxygen delivery will generally optimize cardiac output even at the expense of increased ventricular afterload. If myocardial oxygen delivery can be improved, then heart function will improve. There is no clear evidence that any vasopressor or combination of agents is better than another at improving CRI. Finally, fluid and vasopressor administration must be guided by clinical indices of organ perfusion and must be individualized for each patient.
When seeking to minimize oxygen consumption, the clinician must optimize pharmacologic sedation to reduce excessive activity. In extreme cases, neuromuscular blockade may be appropriate. The clinician should consider treating high fevers aggressively to help decrease oxygen consumption.
PEEP and Oxygen Delivery
Although a thorough discussion of optimal PEEP is beyond the scope of this presentation, it must be noted that optimal PEEP plays a key role in optimizing cardiorespiratory interactions. It is clear that as the lungs are expanded, oxygenation improves. If the lungs are allowed to collapse, oxygenation declines. From that, some may conclude that more PEEP is the best tool for optimizing oxygen delivery because it is improves oxygenation. However, as the lungs are overdistended through the use of PEEP, cardiac output may deteriorate. In fact, oxygen delivery may be the lowest when PaO2 is at its best if cardiac output significantly deteriorates with excessive PEEP. So, the goal is not necessarily to optimize PaO2 or oxygen saturation but rather to optimize oxygen delivery.
Oxygen Dissociation Curve
It is important to understand that many entities, among them permissive hypercapnia, play affect the oxygen dissociation curve. If the oxygen dissociation curve can be shifted to the right, the release of oxygen to the tissues can be improved. For the same Sao2, the PaO2 increases. What shifts the oxygen dissociation curve rightward? If the clinician decreases the pH level while letting the CO2 level increase, oxygen delivery can actually increase. Thus, permissive hypercapnia can be used to prevent ventilator-induced lung injury and also to help cardiorespiratory interactions by allowing the body to unload oxygen to the tissues and improve tissue oxygenation.
Tissue Oxygenation
When trying to optimize tissue oxygenation, the clinician must examine the relationship between oxygen delivery and tissue oxygenation. There is a portion of the curve where oxygendelivery directly affects tissue oxygenation, but once a critical point is passed, more is not necessarily better. Again, going to supranormal values to make oxygendelivery as high as it can be does not necessarily help as there are potential toxicities associated with interventions.
Another important point is that everything clinicians can assess in their patients are global indicators of oxygen delivery. Even if global indicators appear adequate, there may be regional areas of tissue that remain hypoxic. However, regional oxygen delivery is nearly impossible to assess. This is common in neonates who develop necrotizing enterocolitis. Even though global indicators of oxygen delivery may appear appropriate, regions of the gastrointestinal tract may be compromised.
Conclusion
In conclusion, oxygen delivery—but not necessarily PaO2 or Sao2—must be optimized for all vital organs and tissues. Specifically, the mean airway pressure must be titrated to optimize the balance between mean lung volume (ie, arterial oxygenation) and ventricular function (ie, cardiac output). PVR must be minimized. Cardiorespiratory parameters must be monitored as closely as possible as the bottom line is that the heart and lungs are connected both anatomically and physiologically.
References
- Lyrene RK, Welch KA, Godoy G, et al. Alkalosis attenuates hypoxic pulmonary vasoconstriction in neonatal lambs. Pediatr Res. 1985;19:1268–1271.
- Malik AB, Kidd BS. Independent effects of changes in H+ and CO2 concentrations on hypoxic pulmonary vasoconstriction. J Appl Physiol. 1973;34:318–323.
- Cheifetz IM, Craig DM, Quick G, et al. Increasing tidal volumes and pulmonary overdistention adversely affect pulmonary vascular mechanics and cardiac output in a pediatric swine model. Crit Care Med. 1998;26:710–716.
- Third European Consensus Conference in Intensive Care Medicine. Tissue hypoxia: how to detect, how to correct, how to prevent. Am J Respir Crit Care Med. 1996;154:1573–1578.
Self-Assessment
- What can be done to increase oxygen content?
(a) Increase hemoglobin (Hgb), SaO2, and PaO2
(b) Decrease Hgb, SaO2, and PaO2
(c) Increase Hgb but decrease SaO2 and PaO2
(d) Decrease cardiac output
- What is the best way to measure tissue oxygen delivery?
(a) Measure serum lactate level
(b) Assess acid–base balance
(c) Monitor mixed venous oxygen saturation (SvO2)
(d) None of the above

Full access? Get Clinical Tree
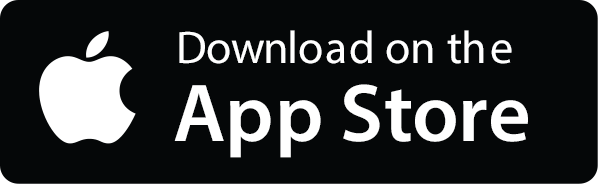
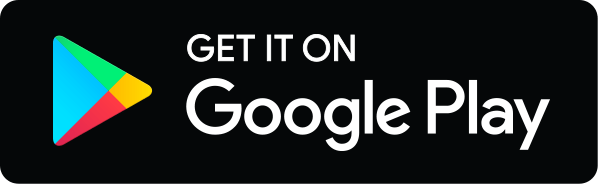