Venous Thromboembolism: Pulmonary Embolism and Deep Venous Thrombosis
Charles William Hargett III
Victor F. Tapson
Incidence and Natural History
Venous thromboembolism (VTE) includes the spectrum of deep vein thrombosis (DVT) and pulmonary embolism (PE). Embolization of material into the pulmonary venous circulation may lead to marked cardiopulmonary dysfunction and is of particular interest to the critical care practitioner. Although VTE is extraordinarily common in hospitalized patients, estimating the frequency of VTE and PE is problematic. The nonspecific clinical findings and high rate of undiagnosed events likely underestimate the true incidence of disease, whereas autopsy data may overestimate meaningful events by detecting asymptomatic cases. That being considered, the incidence of VTE is thought to be in excess of 600,000 cases per year in the United States [1]. In one population-based study with autopsy data, the annual incidences (age and sex adjusted) of DVT and PE were 48 and 69 per 100,000, respectively [2]. In a study of critically ill patients, 10% to 30% of medical/surgical intensive care unit (ICU) patients experienced DVT within the first week of admission, and approximately 60% of trauma patients had DVT within the first 2 weeks, most of which were clinically silent [3]. The prevalence of VTE as a cause of critical illness is also uncertain, but approximately 15% to 20% of patients with diagnosed PE have significant hemodynamic and/or respiratory compromise [4,5]. Untreated symptomatic PE has a mortality rate of approximately 30%, but treatment reduces this risk considerably [6,7,8,9,10,11,12,13]. Adjusting for patients with concomitant terminal illnesses, acute PE likely account for more than 100,000 deaths per year in the United States in patients with an otherwise good prognosis [14,15].
Risk Factors
Recognizing the presence of risk factors for VTE is essential because more than 90% of deaths due to PE occur in patients who are not treated because the diagnosis was unsuspected and thus undetected [14,15]. Virtually every risk factor for VTE can be derived from Virchow’s triad of stasis, venous injury, and hypercoagulability described nearly 150 years ago (Tables 52.1 and 52.2) [16]. Common major risk factors for VTE include increased age, malignancy, surgery, hospitalization with acute medical illness, and a history of prior VTE. Critically ill patients may be at especially high risk for VTE due to severe underlying disease, immobility, and venoinvasive catheters, and the incidence of VTE increases correspondingly with the number of risk factors present.
Inherited or acquired hypercoagulable states are now recognized in more than 20% of patients with VTE and occur at an even higher rate in patients with idiopathic or recurrent VTE (Table 52.3) [17,18]. Resistance to activated protein C due to the factor V Leiden mutation is one of the most common hypercoagulable states, with a prevalence of 5% in white individuals of European ancestry, and may be present in perhaps 20% of patients with PE [19,20,21,22]. Ethnicity is a risk factor, and whites and African Americans have a significantly higher incidence of VTE as compared with Hispanics and Asians [23].
Indwelling central venous catheters (CVCs), particularly common in critically ill patients, provide a constant nidus for clot formation. Thrombosis may occur in up to 67% of patients with invasive catheters [24]. Specific risk factors for thrombotic complications of CVCs may be related to technical or host factors and include femoral vein site, duration of cannulation (> days), nighttime placement, extremes of age, and multiple lines [25,26,27,28]. The frequency of clinically meaningful complications due to CVC-related thrombosis remains unclear.
Pathophysiology
The sequence of events leading to venous thrombosis is not fully understood and likely varies based on the dynamic interactions between genetic and acquired risk factors. In one proposed scheme, endothelial stimulation results from either blood
stasis-induced hypoxia or direct vein wall injury, after which point tissue factor is transferred to the endothelial cell and initiates the enzymatic cascade of coagulation reactions, leading to thrombin generation and fibrin deposition [29]. Thus, it is not surprising that many venous thrombi arise in valve pockets, where blood flow tends to stagnate, or at a specific area of vascular disruption, such as an indwelling catheter site.
stasis-induced hypoxia or direct vein wall injury, after which point tissue factor is transferred to the endothelial cell and initiates the enzymatic cascade of coagulation reactions, leading to thrombin generation and fibrin deposition [29]. Thus, it is not surprising that many venous thrombi arise in valve pockets, where blood flow tends to stagnate, or at a specific area of vascular disruption, such as an indwelling catheter site.
Table 52.1 Strong, Moderate, and Weak Risk Factors for Venous Thromboembolism (VTE) | ||
---|---|---|
|
Lower extremity DVT is the most frequent source of PE and, in untreated patients with proximal DVT, approximately half will develop PE [30,31]. Other sources of PE include pelvic, renal, or upper extremity veins, as well as the right heart. After traveling to the lungs, a large thrombus may occlude a major pulmonary artery and cause significant cardiovascular symptoms, or it may break up into smaller clots and travel distally, where it is more likely to produce pleuritic chest pain. Thrombi are most frequently carried to the lower lobes due to the higher blood flow. Pulmonary infarction is relatively uncommon because of incomplete vessel occlusion by emboli and bronchial artery anastomoses.
Ventilation/perfusion ([V with dot above]/[Q with dot above]) mismatch is the principal physiologic effect of PE and leads to hypoxemia in most patients. [V with dot above]/[Q with dot above] mismatch results from increased physiologic dead space and intrapulmonary shunting, which frequently produces an elevated alveolar-to-arteriolar oxygen gradient. Concomitant physiologic responses to PE include increased minute ventilation and airways resistance, as well as decreased vital and diffusion capacities [32,33,34,35,36]. In patients with a potentially patent foramen ovale, progressive pulmonary hypertension may lead to a right-to-left intra-atrial shunt, resulting in worsening hypoxemia and, rarely, paradoxical embolization.
The hemodynamic response to PE may vary depending on the degree of occlusion of the pulmonary arterial circulation and on the presence of underlying cardiopulmonary disease. Physiologically, PE causes a decrease in the cross-sectional area of the pulmonary arterial bed and leads to an increase in the pulmonary vascular resistance. This impedes right ventricular outflow, leading to reduced left ventricular preload and, ultimately, a diminished cardiac output. Progressive vascular obstruction and hypoxemia stimulates vasoconstriction and a further rise in pulmonary artery pressure. The normal right ventricle fails acutely when it cannot generate a systolic pressure to overcome a mean pulmonary artery pressure greater than 40 mm Hg needed to maintain pulmonary perfusion. The normal pulmonary circulation has a large reserve capacity and more than 50% obstruction is generally required for a substantial increase in the mean pulmonary artery pressure [37,38].
Patients with underlying cardiopulmonary disease have less physiologic reserve as compared with healthy individuals, and it follows that they may suffer right heart failure with a lesser degree of pulmonary vascular occlusion [39,40].
Patients with underlying cardiopulmonary disease have less physiologic reserve as compared with healthy individuals, and it follows that they may suffer right heart failure with a lesser degree of pulmonary vascular occlusion [39,40].
Table 52.2 the Presence of Risk Factors in Patients Treated for Acute Venous Thromboembolism (VTE) | ||||||||||||||||||||||||||||||||||||||
---|---|---|---|---|---|---|---|---|---|---|---|---|---|---|---|---|---|---|---|---|---|---|---|---|---|---|---|---|---|---|---|---|---|---|---|---|---|---|
|
Table 52.3 Inherited and Acquired Risk Factors for Venous Thromboembolism | ||||||||
---|---|---|---|---|---|---|---|---|
|
Clinical Manifestations
Recognizing the presence of VTE may be challenging as neither the signs nor symptoms associated with DVT and PE are sensitive or specific for the diagnoses [13,14,41,42]. Because DVT is usually asymptomatic and most cases of fatal PE are unsuspected prior to death, the most critical step in the diagnosis of VTE is the development of a clinical suspicion of the disease [6,43,44]. This suspicion is based on the constellation of risk factors, symptoms, signs, electrocardiography, blood tests, and chest radiographic findings. Although clinical assessment alone is inadequate in diagnosing and excluding DVT and PE, both clinical gestalt and clinical prediction rules are useful in establishing a pretest probability in which patients are typically classified into three groups based on the estimated prevalence of disease (Table 52.4) [45,46,47,48,49]. This clinical pretest probability serves as the root of algorithms for the diagnosis of DVT and PE [50,51,52,53].
The diagnosis of VTE in the critically ill patient may be particularly challenging. Underlying systemic illnesses or other superimposed acute illness may mimic or mask the common signs and symptoms of VTE. Also, common clinical likelihood models for predicting VTE may not be valid in the ICU setting [54]. Furthermore, definitive testing for VTE may be precluded by relative contraindications, such as mechanical ventilation, shock, and renal failure.
Symptoms and Signs
Although most DVT begins in the calf, the presenting symptoms and signs are often not noted until more proximal veins are involved [55]. The initial clinical manifestations of DVT may be acute, progressive, or resolve spontaneously and may include warmth, erythema, swelling, and pain or tenderness. Pain on forced dorsiflexion of the foot (Homans’ sign) is neither sensitive nor specific for DVT [42]. The differential diagnosis of DVT should always be framed by the clinical presentation and consideration of risk factors for VTE. Cellulitis, trauma, Baker’s cyst, musculoskeletal pain, or asymmetric edema unrelated to DVT may all result in signs and symptoms compatible with acute DVT.
Table 52.4 Determining the Pretest Probability of Acute Pulmonary Embolism (PE) Using A Standardized Point Systema | ||||||||||||||||||||||||||
---|---|---|---|---|---|---|---|---|---|---|---|---|---|---|---|---|---|---|---|---|---|---|---|---|---|---|
|
Most patients with proven acute PE present with at least one of the following: dyspnea, pleuritic chest pain, or tachypnea (Table 52.5). Other findings may include tachycardia, a loud pulmonic component of the second heart sound, fever, crackles, pleural rub, wheezing, and/or leg tenderness or swelling. Pleuritic chest pain and hemoptysis occur more commonly with pulmonary infarction due to smaller, peripheral emboli. PE must always be considered in cases of unexplained dyspnea, syncope, or sudden hypotension. Symptoms and signs of PE are nonspecific and may frequently be seen in patients with concomitant cardiopulmonary disease; these findings may be due to a coexisting disease or a superimposed acute PE. Pulmonary embolism may be confused with many conditions including pneumonia, chronic obstructive lung disease exacerbation, pneumothorax, myocardial infarction, heart failure, pericarditis, musculoskeletal pain or trauma, pleuritis, malignancy, and, occasionally, intra-abdominal processes such as acute cholecystitis.
Given the kaleidoscopic presentation of VTE and the significant associated morbidity and mortality, there should be a low threshold for the clinical suspicion of PE in the ICU. Subtle signs such as worsening hypoxemia, a reduction in arterial carbon dioxide with spontaneous respirations (especially in a patient with chronic lung disease), increased central venous or pulmonary artery pressure, or unexplained fever should all be considered potential heralds of PE. Even in the presence of alternative diagnoses, the evaluation for possible VTE may still be appropriate when suggestive signs, symptoms, and risk factors are present.
Diagnostic Tests
Chest Radiograph and Electrocardiogram
Under almost all circumstances, chest radiography cannot be used for the conclusive diagnosis or exclusion of PE. The chest radiograph is abnormal in more than 80% of patients with PE, but is nearly always nonspecific, with common findings including atelectasis, pleural effusion, pulmonary infiltrates, and mild elevation of a hemidiaphragm [41,56,57]. Classic findings such as Hampton’s hump (juxtapleural wedge-shaped opacity at the costophrenic angle indicating pulmonary infarction) or Westermark’s sign (focally decreased vascularity distal to the occlusion) are suggestive of the diagnosis of PE but are infrequently seen. These findings may be even more difficult to appreciate on portable anteroposterior films commonly employed in the ICU. A normal chest radiograph in a patient with severe dyspnea and hypoxemia and without bronchospasm or cardiac shunt strongly suggests the diagnosis of PE [56]. In young patients with acute pleuritic chest pain, the presence of a pleural effusion raises the probability of PE [58]. Although chest radiography may be useful in excluding other thoracic conditions (e.g., pneumothorax, rib fracture, pneumonia) that may produce signs and symptoms similar to PE, it is important to remember that PE may be present with other cardiopulmonary diseases.
The electrocardiogram is commonly abnormal but findings are nonspecific in most patients with acute PE. Tachycardia, T-wave and ST-segment changes, and right- or left-axis deviation are common electrocardiography findings [41,59]. The
classic patterns of S1Q3T3; right ventricular strain; and new, incomplete, right bundle branch block are less commonly seen but may be more frequent with massive PE and cor pulmonale. A subepicardial ischemic pattern (T-wave inversion in the precordial leads) seems to correlate with the severity of PE and degree of right ventricular dysfunction [60].
classic patterns of S1Q3T3; right ventricular strain; and new, incomplete, right bundle branch block are less commonly seen but may be more frequent with massive PE and cor pulmonale. A subepicardial ischemic pattern (T-wave inversion in the precordial leads) seems to correlate with the severity of PE and degree of right ventricular dysfunction [60].
Table 52.5 Symptoms and Signs in Patients with Acute Pulmonary Embolism Without Preexisting Cardiopulmonary Disease | ||||||||||||||||||||||||||||||||||||||||||||||||||||||||||||||||
---|---|---|---|---|---|---|---|---|---|---|---|---|---|---|---|---|---|---|---|---|---|---|---|---|---|---|---|---|---|---|---|---|---|---|---|---|---|---|---|---|---|---|---|---|---|---|---|---|---|---|---|---|---|---|---|---|---|---|---|---|---|---|---|---|
|
Radiographic and electrocardiographic abnormalities are quite common in patients diagnosed with PE. Although these findings are nonspecific, it follows that a completely normal chest radiograph and electrocardiogram decrease the likelihood of PE.
Arterial Blood Gas and End-Tidal Carbon Dioxide
Arterial blood gas analysis is frequently used in assessing the severity of cardiopulmonary disease but is of limited value in diagnosing PE. Only about 85% to 90% of patients with proven PE have hypoxemia and an elevated alveolar–arterial difference [41,56,61,62]. Hypoxemia is almost uniformly present when there is a hemodynamically significant PE. Interestingly, however, in the Prospective Investigation of Pulmonary Embolism Diagnosis (PIOPED) study, there was no difference in either PaO2 or P(A-a)O2 between patients with or without PE, reflecting the common gas-exchange abnormalities present in multiple cardiopulmonary conditions. Due to increased ventilation, patients with PE will generally have a normal or reduced arterial carbon dioxide tension. Physiologic changes in PE may be particularly variable in young patients and those without underlying lung or heart disease. In any case, arterial blood gas values are of insufficient discriminant value to exclude the diagnosis of PE.
As a function of alveolar dead space, end-tidal CO2 is a physiologically intuitive marker of pulmonary arterial blood flow that unfortunately has been of limited utility in identifying patients with PE [63,64,65,66,67]. End-tidal CO2 may be physiologically insensitive for PE because of incomplete vessel occlusion by thrombus and also because of decreased ventilation at embolized areas due to local bronchoconstriction. Other cardiopulmonary conditions may alter the difference between the PaCO2 and the end-tidal CO2, decreasing the specificity for PE.
D-Dimer
Plasma measurements of D-dimer (a specific derivative of cross-linked fibrin) have been extensively studied in patients with acute DVT and PE [68,69,70]. Multiple inexpensive D-dimer tests are available, but rapid enzyme-linked immunosorbent assays are preferred. When used in the outpatient setting, D-dimer measurements are very sensitive and have shown a high negative predictive value in excluding the presence of VTE when used in concert with a low clinical pretest probability [51,71]. The low specificity of D-dimer testing for VTE (i.e., many conditions are associated with elevated levels) makes it less useful in unselected and hospitalized patients [72]. It follows that the positive predictive value of an elevated D-dimer for VTE is low and should not be used in isolation to initiate further evaluation.
Cardiac Troponin and Brain Natriuretic Peptide
Troponin is specific for cardiac myocyte damage, and patients with right ventricular strain due to acute PE may sometimes have elevated troponin T and I levels [73,74]. Patients with PE and elevated troponins are more likely to have elevated right ventricular systolic pressures, right ventricular dilation/hypokinesis, and are at increased risk for cardiogenic shock. Not surprisingly, a positive troponin is more common with large clot burdens and confers an increased risk of death [75,76]. Although an elevated troponin may hint at a potential
diagnosis of PE in the appropriate clinical setting, a normal value is not sufficiently sensitive to rule out PE.
diagnosis of PE in the appropriate clinical setting, a normal value is not sufficiently sensitive to rule out PE.
Plasma brain natriuretic peptide (BNP) is released in response to increased cardiac-filling pressure and can serve as a supplementary tool for evaluating right ventricular function in patients with acute PE. BNP appears elevated in the majority of cases of PE with right ventricular overload and may help in risk stratification [77,78,79]. However, because plasma BNP levels rise in a variety of cardiopulmonary conditions and are affected by several physiological factors, they are not diagnostic for PE.
Ventilation/Perfusion Scanning
Despite the increased use of contrast-enhanced spiral computed tomography (CT) of the chest, [V with dot above]/[Q with dot above] scanning is still frequently used in suspected PE. [V with dot above]/[Q with dot above] scans may be deemed normal or, when abnormal, are conventionally read as showing low, intermediate, or high probability of PE. A normal scan essentially excludes the diagnosis of PE. Otherwise, [V with dot above]/[Q with dot above] scanning can be combined with clinical suspicion in a Bayesian fashion to improve the accuracy of diagnosis. In general, the predictive value of a [V with dot above]/[Q with dot above] scan is highest with a concordant clinical likelihood assessment. In the PIOPED study, when the clinical suspicion of PE was high, PE was present in 96% of patients with high-probability lung scans [5]. However, in patients with a high clinical pretest probability for PE, 66% of patients with intermediate probability scans and 40% of patients with low probability scans were subsequently diagnosed with PE by pulmonary angiography. This emphasizes that low- and intermediate-probability [V with dot above]/[Q with dot above] scans are nondiagnostic when there is a high clinical suspicion for PE. In the setting of a low clinical pretest probability for PE, a normal or low-probability [V with dot above]/[Q with dot above] scan correctly excluded PE in more than 95% of cases. Because PE is commonly found in low- or intermediate-probability [V with dot above]/[Q with dot above] scans, such findings are generally considered nondiagnostic and further evaluation is often appropriate.
Although [V with dot above]/[Q with dot above] scanning can be successfully used in the intensive care setting, ventilation scans generally cannot be performed on mechanically ventilated patients, and the availability of bedside scintigraphic perfusion imaging has decreased [5,80,81]. The optimal scenario for the [V with dot above]/[Q with dot above] scan is in the patient with a clear chest radiograph and without underlying cardiopulmonary disease. Large PE, however, are occasionally identified with portable perfusion scans based upon very large perfusion defects in ICU patients.

Full access? Get Clinical Tree
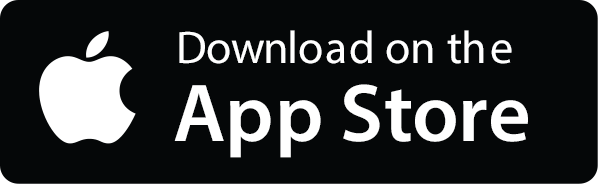
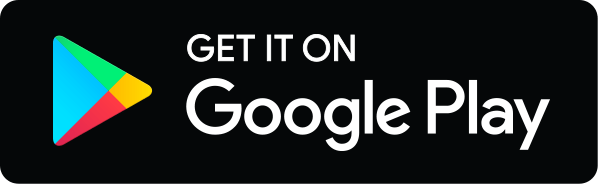