Valvular Heart Disease
Natalia S. Ivascu
Gregg S. Hartman
Nikolaos J. Skubas
A 78-year-old man was admitted with increasing shortness of breath. He had chest pain in the past but was able to continue with normal activities. He had passed out twice in the past year. On physical examination, a loud systolic murmur could be heard at the left sternal border radiating to the neck. His vital signs were blood pressure 150/90 mm Hg and heart rate 88 beats per minute and irregular. The electrocardiogram showed sinus rhythm with atrial premature contractions and left ventricular hypertrophy (LVH) with strain. A transthoracic echocardiogram (TTE) showed a hypertrophied left ventricle (LV), and Doppler examination demonstrated severe aortic stenosis (AS) with a pressure gradient of 64 mm Hg, mild aortic insufficiency (AI), and moderate mitral regurgitation (MR). He was scheduled for aortic valve (AV) replacement and possible mitral valve (MV) repair or replacement.
A. Medical Disease and Differential Diagnosis
What are the major etiologies of AS, AI, mitral stenosis (MS), and MR?
What are the major changes in the loading conditions of the LV that result from the four different lesions? Why do they occur? What changes result from them?
What are pressure-volume (P-V) loops? What do the different inflection points represent?
What are representative P-V loops for the four valvular lesions?
Draw the pressure/time curves for the LV, left atrium, pulmonary artery, and aorta for a normal patient and for patients with each of the four valvular lesions.
What are the basic principles of echocardiography? What are M-mode, B-mode, and Doppler color modalities? How do TTE and transesophageal echocardiography (TEE) differ?
What are the three TEE vantage points for the comprehensive imaging of the LV? How are pressure gradients measured by echocardiography? How do the pressure gradients derived from Doppler echocardiography differ from those obtained in the catheterization laboratory by direct pressure measurement?
What is the role for three-dimensional (3D) echocardiography in the operating room?
What are the echocardiographic and cardiac catheterization criteria for the four valvular lesions?
B. Preoperative Evaluation and Preparation
What are the presenting signs and symptoms of the four valvular lesions listed previously?
What is the New York Heart Association classification of heart failure?
Discuss the role of premedication for patients with the four different valvular lesions.
How would you premedicate the patient with severe AS and MR?
C. Intraoperative Management
Outline the hemodynamic management goals for each of the four valvular lesions. What are the anesthetic goals with respect to heart rate and rhythm, preload, afterload, and contractility?
What are the hemodynamic goals for this patient with the combination of severe AS and MR?
How would you monitor this patient with severe AS and MR?
Should the patient have a pulmonary artery catheter placed before induction?
Is a pulmonary artery catheter with pacing capabilities indicated?
What anesthetic technique would you employ? Why?
What muscle relaxant would you use for this patient?
What are the usual TEE findings in a patient with AS or MR? How do you grade the severity of AS by TEE? How do you quantify the severity of MR? What is the impact of AS on the severity of MR?
What special considerations particular to cardiopulmonary bypass (CPB) operations do you have for each of the four lesions? Focus on these concerns with respect to the induction and prebypass, bypass, and postbypass periods.
The patient cannot be weaned from CPB following an AV replacement and MV replacement. What are the possible causes?
How would you diagnose right-sided heart failure and pulmonary hypertension? How would you treat it?
How does an intra-aortic balloon pump (IABP) work to benefit the failing heart?
What role does the IABP have in this setting?
What role does TEE play in the placement, timing, and demonstration of efficacy of an IABP?
How would you properly time the IABP cycle?
What are the contraindications to the use of an IABP?
What is the role for ventricular assist devices?
D. Postoperative Management
In the intensive care unit (ICU) 4 hours later, the patient became hypotensive with a low cardiac output. How could you distinguish between cardiac tamponade and pump failure? How would the TEE images differ?
Would you extubate this patient early in the ICU? Why?
What are the advantages and disadvantages of early extubation?
E. Alternative Treatments
What are the percutaneous options for MV repair?
How is percutaneous MV repair performed?
What are the percutaneous options for AV repair and replacement?
How is percutaneous AV replacement performed?
What anesthetic techniques and monitors are used?
What is minimally invasive cardiac valve surgery?
A. Medical Disease and Differential Diagnosis
A.1. What are the major etiologies of AS, AI, mitral stenosis (MS), and MR?
AS occurs as a congenital lesion but more commonly as an acquired disease. Stenosis may develop on a previously normal AV following rheumatic fever or from progressive calcification. Congenitally bicuspid AV are also prone to calcification with eventual stenosis. Calcification of the AV leaflets can result in incomplete closure of the valve with associated insufficiency.
AI is usually an acquired disease. The most common causes include bacterial endocarditis and rheumatic heart disease. Annular dilation with resultant AI may result from diseases such as cystic medial necrosis and collagen disorders or following aortic dissection.
MS is almost always caused by rheumatic fever, although only half of patients will have a history of an acute febrile illness. The inflammatory process of rheumatic fever results in thickening of the leaflets and fusion of the commissures. Other rare causes include congenital stenosis and other systemic diseases, such as systemic lupus erythematosus and carcinoid. Pathophysiology similar to that seen with valvular MS can occur with obstructing left atrial tumors and cor triatriatum. MS commonly occurs in conjunction with other valvular heart disease; only 25% of patients present with isolated MS, and approximately 40% have combined MS and MR.
MR can result from defects in the leaflets, the annular ring or the supporting chords or papillary muscles, or any combination of these. Primary leaflet dysfunction occurs with rheumatic fever but can also follow bacterial endocarditis, connective tissue disorders, and congenital malformations. Annular dilation can follow left ventricular dysfunction and dilation. MV prolapse and rupture of papillary muscles result in incomplete leaflet closure or coaptation with resultant MR. Left ventricular ischemia can affect papillary muscle contraction and is the cause of functional MR.
Czarny MJ, Resar JR. Diagnosis and management of valvular aortic stenosis. Clin Med Insights Cardiol. 2014;8(suppl 1):15-24.
Helms AS, Bach DS. Heart valve disease. Prim Care. 2013;40(1):91-108.
Iung B, Vahanian A. Epidemiology of acquired valvular heart disease. Can J Cardiol. 2014;30(9):962-970.
Nishimura RA, Otto CM, Bonow RO, et al. 2014 AHA/ACC guideline for the management of patients with valvular heart disease: executive summary: a report of the American College of Cardiology/American Heart Association Task Force on Practice Guidelines. Circulation. 2014;129(23):2440-2492.
A.2. What are the major changes in the loading conditions of the LV that result from the four different lesions? Why do they occur? What changes result from them?
AS represents a chronic systolic pressure load on the LV. This increases the wall tension (stress) in accordance with Laplace’s law:

The LV undergoes parallel duplication of muscle fibers in an attempt to compensate, and this results in increased wall thickness or concentric hypertrophy and some decrease in radius, thereby normalizing wall stress. If the MV remains competent, little change occurs in the other cardiac chambers.
AI causes left ventricular diastolic volume overload due to the additional volume flowing retrograde into the LV across the incompetent AV during diastole. This results in eccentric hypertrophy and left ventricular dilation (increased muscle mass and chamber radius). However, AI decreases the aortic diastolic pressure, which is the pressure that must be exceeded by the contracting LV to open the AV and result in LV systolic ejection. Therefore, LV work is performed against a lower outflow impedance (aortic diastolic pressure). Stroke volume and ejection fraction may be preserved until late in the disease process. As with AS, the presence of a competent MV confines the changes to the LV. However, the LV dilation that follows chronic AI may result in mitral annular dilation or alteration in the papillary muscle orientations with resultant MR. Left atrial enlargement secondary to MR, or left atrial pressure overload as LV end-diastolic pressure (LVEDP) rise in the course of AI can occur.
MS results in a chronically underfilled LV because of progressive obstruction to left atrial emptying. This chronic underloading condition can result in diminished contractile function (a “disuse atrophy” of sort). In addition, if the cause of the MS is rheumatic, myofibril damage may also occur. Contrary to the LV pressure and volume underloading, the left atrium is both pressure and volume overloaded in order to maintain flow across the progressively narrowing mitral orifice. According to Gorlin’s equation for pressure gradient:

It would predict that the pressure gradient increases by the square of any increase in flow rate or decrease in valve area. The elevated left atrial pressure leads to hypertrophy and eventually dilation that predisposes to premature atrial contractions and subsequent atrial fibrillation. The loss of atrial contraction will further diminish the flow across the stenotic MV. At the same time, the elevated left atrial pressure hinders pulmonary venous flow with consequent pulmonary vascular engorgement. This will lead to intimal hypertrophy that induces irreversible elevations in pulmonary vascular resistance. As a consequence of pulmonary hypertension, the right ventricular (RV) systolic pressure increases and RV dilates, and these may lead to RV failure and tricuspid regurgitation.
MR results in volume overload of the LV. In MR, the LV ejects into both systemic circulation (across the AV; high afterload and low compliance) and retrograde to the left atrium (low afterload and high compliance) across the incompetent MV. This “back and forth” of the regurgitant volume results in LV volume overload. However, this work is performed at a low pressure; therefore, LV wall tension is minimally increased initially. As with AI, the volume overload results in marked LV dilation and eccentric hypertrophy. At the same time, the left atrium is also volume overloaded and undergoes dilation. When the volume overload occurs slowly, the pulmonary pressures increases minimally, despite large regurgitant volumes. In contrast, acute MR, for example, following an acute myocardial infarction with papillary muscle rupture, presents a sudden volume overload to the left atrium. Without the time to dilate, the left atrial pressure rapidly rises, limiting pulmonary drainage with resultant pulmonary engorgement and acute increase in pulmonary pressures.
Frogel J, Galusca D. Anesthetic considerations for patients with advanced valvular heart disease undergoing noncardiac surgery. Anesthesiol Clin. 2010;28:67-85.
Mann DL, Zipes DP, Libby P, et al, eds. Braunwald’s Heart Disease. 10th ed. Philadelphia, PA: Elsevier; 2015:1446-1523.
Schoen FJ. Cardiac valves and valvular pathology: update on function, disease, repair, and replacement. Cardiovasc Pathol. 2005;14:189-194.
A.3. What are pressure-volume (P-V) loops? What do the different inflection points represent?
The P-V loop analysis (Fig. 7.1) depicts the relation between LV volume and pressure during a single cardiac cycle. Opening and closing of the MV and AV are represented by the inflection points A, B, C, and D, respectively (Fig. 7.1). Moving from points A through D, AB depicts left ventricular filling, BC depicts isovolumetric contraction, CD shows left ventricular ejection, and DA shows isovolumetric relaxation. Point A coincides with opening of the MV and represents LV end-systolic volume and early diastolic pressure. Point B is closure of the MV and the relationship of end-diastolic pressure (LVEDP) and volume (LVEDV). Point C represents the opening of the AV and coincides with systemic, aortic diastolic pressure. Finally, point D is the closure of the AV and represents LV end-systolic pressure (LVESP) and volume (LVESV), coinciding with the dicrotic notch in the aortic pressure tracing (Fig. 7.1). LV compliance is the relation between pressure and volume and is defined by the slope of the filling phase (segment AB). Preload is the P-V relation before the onset of contraction (LVEDP). Contractility may be illustrated by the slope of a line termed the end-systolic pressure-volume relation (ESPVR). The ESPVR slope is created by connecting multiple points (D) from multiple P-V loops generated by changing the filling volume to the LV (Fig. 7.2). Increased contractility results in a steeper line, whereas diminished contractility results in a flatter line. The P-V loop analysis permits illustration of stroke volume and ejection fraction. Stroke volume is defined as difference in volume from the end of filling to the end of ejection (EDV-ESV), whereas the ejection fraction is the ratio of stroke volume to total volume in the heart at peak filling (SV/EDV). Therefore, the P-V loop analysis permits illustration of the volume-pressure relations and their changes with each of the four valvular lesions.
Barash PG, Cullen BF, Stoelting RK, et al, eds. Clinical Anesthesia. 7th ed. Philadelphia, PA: Wolters Kluwer/Lippincott Williams & Wilkins; 2013:239-262.
Carabello BA. Volume overload. Heart Fail Clin. 2012;8(1):33-42.
Mérillon JP, Ennezat PV, Guiomard A, et al. Left ventricular performance is closely related to the physical properties of the arterial system: landmark clinical investigations in the 1970s and 1980s. Arch Cardiovasc Dis. 2014;107(10):554-562.
Suga H. Cardiac energetics: from E(max) to pressure-volume area. Clin Exp Pharmacol Physiol. 2003;30(8):580-585.
A.4. What are representative P-V loops for the four valvular lesions?
The hallmarks of AS, illustrated by the P-V loop analysis, are an increased LV systolic pressure and an upward and counterclockwise rotation in the end-diastolic P-V relation (AB) indicative of decreased chamber compliance (Fig. 7.3). Stroke volume and ejection fraction are well preserved, but the ejection phase of the loop occurs at much higher pressures.
This is permitted by an increase in contractility (counterclockwise rotation of the ESPVR line).
This is permitted by an increase in contractility (counterclockwise rotation of the ESPVR line).
The schematic P-V loop for chronic AI depicts an enlarged LV. The minimal change in LVEDP despite the large volume overload is seen by the shift in the diastolic P-V curve to the right (A″B″) (Fig. 7.4). Low systemic diastolic pressures result in a brief isovolumetric phase (B″C″) and early complete ejection. The isovolumetric relaxation phase is absent because the incompetent valve permits regurgitant filling of the LV from the aorta during diastole even before opening of the MV. In contrast, when acute AI occurs, the LV compliance is unchanged. Rapid increases in LVEDP from volume overload along the unshifted LV diastolic P-V curve (A′B′) rapidly lead to increased left atrial pressure and pulmonary congestion.
The P-V loop of MS illustrates hypovolemia, the cause of which cannot be determined from the loop alone (Fig. 7.5). Because the predominant impact of MS occurs proximal to the LV, the P-V analysis format is less useful.
In MR, the diastolic P-V relation (line AB) is shifted to the right, as it is in chronic AI, consistent with a marked increase in compliance (Fig. 7.6). The isovolumetric phase (BC) is nearly absent because the left atrium generally serves as a low-pressure/high-compliance route for ejection through an incompetent MV. Decreases in contractility are depicted by a decrease in the slope of the end-systolic-PV line (line through D).
Carabello BA, Zile MR, Tanaka R, et al. Left ventricular hypertrophy due to volume overload versus pressure overload. Am J Physiol. 1992;263(4 pt 2):H1137-H1144.
Nishimura RA, Carabello BA. Hemodynamics in the cardiac catheterization laboratory of the 21st century. Circulation. 2012;125(17):2138-2150.
A.5. Draw the pressure/time curves for the LV, left atrium, pulmonary artery, and aorta for a normal patient and for patients with each of the four valvular lesions.
Normal curves are shown in Figure 7.7. The points A, B, C, and D correspond to the same points in the P-V loops.
Aortic Stenosis
The additional systolic pressure work of AS can be seen in the LV pressure tracing (Fig. 7.8). Elevations in LVEDP (point B) can be seen to diminish the perfusion gradient for coronary flow to the LV. The augmentation in LV filling late in diastole secondary to atrial contraction (left atrial “kick” from sinus rhythm) is highlighted in the inset. Rising LV diastolic pressures secondary to decreased compliance necessitate elevations in left atrial pressures to permit complete LV volume loading. Atrial systole provides this elevation in left atrial pressure synchronous with elevations in LVEDP while keeping left atrial pressures relatively low during the remaining cardiac cycle facilitating pulmonary venous drainage.
Aortic Insufficiency
The rapid upstroke and rapid decline of arterial pressure indicate absence of AV closure and low end-diastolic aortic pressure (Fig. 7.9). Elevations in LVEDV and LVEDP are typical of AI. The early increase in LVEDP can result in LV pressures exceeding those of the left atrium during diastole, with resultant premature closure of the MV.
Mitral Stenosis
Elevations in pressure are seen in both the left atrial and pulmonary artery tracing with MS (Fig. 7.10). The large gradient between left atrial and LV pressures is highlighted in the inset. Chronic elevation in pulmonary volume induces changes in the luminary vascular bed and leads to pulmonary hypertension.
![]() FIGURE 7.7 Pressure curves for the left ventricle, left atrium, pulmonary artery, and aorta in a healthy individual. |
![]() FIGURE 7.8 Pressure curves for the left ventricle, left atrium (LA), pulmonary artery, and aorta in patients with aortic stenosis. LVEDP, left ventricular end-diastolic pressure. |
Mitral Regurgitation
The hallmark of MR is the marked elevations of left atrial pressure during systole and the occurrence of a giant “cv” wave and elevated pulmonary artery pressures (Fig. 7.11).
Carabello BA, Zile MR, Tanaka R, et al. Left ventricular hypertrophy due to volume overload versus pressure overload. Am J Physiol. 1992;263(4 pt 2):H1137-H1144.
A.6. What are the basic principles of echocardiography? What are M-mode, B-mode, and Doppler color modalities? How do TTE and transesophageal echocardiography (TEE) differ?
Echocardiography is the use of sound waves to image structures and blood flow within the heart and great vessels. To image tissue, sound waves are emitted from a transducer at known speeds and constant intervals. The sound packets bounce off structures in their path, and the reflected sound waves are received by the transducer during its “listening” mode. The time it takes for the reflected waves to return to the crystal is measured, and because the velocity of sound in tissues is relatively constant (1,540 m per second), solving for distance can be easily accomplished:
Distance = ½ (velocity × time)
(½ because the distance is traversed twice, once to the object and again on returning).
In this manner, the spatial orientation of cardiac structures can be determined. The strength of the returning signal can be quantified as an amplitude, therefore “A” or amplitude mode (Fig. 7.12B). The echocardiographic system codes this amplitude on a black and white scale, thereby converting the amplitude to brightness or B-mode scanning (Fig. 7.12C).
Figure 7.12D shows this “ice-pick” view through the LV of the heart. Each change in tissue density results in some sound waves being reflected and hence an interface. In this example, bold lines are seen at the epicardial, the endocardial-chamber, the chamber-endocardial, and the epicardial borders. If these amplitude bars are displayed in real time, a motion or M-mode display results (Fig. 7.12E). These images were difficult to reliably obtain and interpret because the views represent a linear slice without surrounding structural images for referencing. If the probe is moved in a sweeping mode, back and forth repetitively, multiple M-mode images can be obtained in a given instant and a two-dimensional (2D) image formed. This rapid back and forth sweep of the ultrasound beam is performed electronically in a phased array transducer. The images derived in this manner appear as a cineradiographic display of myocardial movement. Echocardiography can provide information about the size, shape, location, and movement of myocardial structures.
Figure 7.12D shows this “ice-pick” view through the LV of the heart. Each change in tissue density results in some sound waves being reflected and hence an interface. In this example, bold lines are seen at the epicardial, the endocardial-chamber, the chamber-endocardial, and the epicardial borders. If these amplitude bars are displayed in real time, a motion or M-mode display results (Fig. 7.12E). These images were difficult to reliably obtain and interpret because the views represent a linear slice without surrounding structural images for referencing. If the probe is moved in a sweeping mode, back and forth repetitively, multiple M-mode images can be obtained in a given instant and a two-dimensional (2D) image formed. This rapid back and forth sweep of the ultrasound beam is performed electronically in a phased array transducer. The images derived in this manner appear as a cineradiographic display of myocardial movement. Echocardiography can provide information about the size, shape, location, and movement of myocardial structures.
![]() FIGURE 7.11 Pressure curves for the left ventricle, left atrium, pulmonary artery, and aorta in patients with mitral regurgitation. PAP, pulmonary artery pressure. |
In addition to determining how long it takes for a given sound wave to return and thereby deriving the distance from the transducer, contact of the sound wave packet with the reflecting object (tissue, blood cells, air) will alter the wavelength of the sound packet according to the Doppler principle. When the object coming in contact with the sound wave is moving toward the source of the ultrasound, the reflected ultrasound wavelengths are compressed (shorter). The opposite occurs when the contacted object is moving away from the sound source. These shifts in frequency (ΔF) are proportional to the velocity of the contacted structure, and thereby, the velocity and direction of the encountered object (usually of blood flow) can be calculated. With Doppler ultrasound, only the component of blood flow parallel to the Doppler beam will be analyzed, since the Doppler equation: velocity = c (ΔF) / 2 FT (cos θ), contains the cosine of the angle of incidence between the ultrasound beam and the moving object (FT is the transmitted frequency). Because the cosine of 90 degrees is zero, blood flow that is perpendicular to the ultrasound beam will not cause any Doppler shift and, therefore, cannot be measured. For this reason, it is important to
choose an ultrasound “window” in which the expected blood flow direction is most parallel to the ultrasound. This velocity information can be displayed on a color map (Doppler color flow) or on a time/velocity scale (spectral Doppler display). Doppler-derived blood flow velocity information can determine laminar and turbulent flow patterns, regurgitant or stenotic lesions, and congenital anomalies and can permit quantification of pressure gradients.
choose an ultrasound “window” in which the expected blood flow direction is most parallel to the ultrasound. This velocity information can be displayed on a color map (Doppler color flow) or on a time/velocity scale (spectral Doppler display). Doppler-derived blood flow velocity information can determine laminar and turbulent flow patterns, regurgitant or stenotic lesions, and congenital anomalies and can permit quantification of pressure gradients.
Pressure gradients (ΔP) can be estimated from the measured blood flow velocity using the modified Bernoulli equation (ΔP = 4 × velocity2). Simply, the greater the velocity of blood flow, the higher is the ΔP. TTE uses imaging points or “acoustic windows” obtained with the transducer handheld on the chest wall. TTE is simple and noninvasive. Most standard echocardiograms are obtained from this position. During cardiac surgery, the chest wall is in the sterile field and therefore unavailable. The esophagus lies immediately adjacent to the heart outside of the operative field and affords an excellent imaging vantage point. In 1976, Yasu Oka from the Albert Einstein College of Medicine developed a practical method of intraoperative imaging. She mounted an ultrasound crystal on the end of a gastroscope and, thereby, obtained images of the heart during surgery. This has been refined considerably since. TEE has become the standard of care for heart surgery at many institutions. The close proximity of the probe to the heart affords excellent resolution. The probe is not in the operative field, so surgery is unhindered and sterility is not an issue. Although mildly invasive, the risk for esophageal injury in the anesthetized state is very low.
Intraoperative TEE is beneficial for quantification of cardiac contractility, for determination of the severity of regurgitant and stenotic valvular disease, for detection of intracardiac shunts and the occurrence of dissections, and as a guide for catheter placement.
Oka Y, Goldiner PL, eds. Transesophageal Echocardiography. Philadelphia, PA: JB Lippincott; 1992:9-27.
Savage RM, Aronson S, Shernan SK, eds. Comprehensive Textbook of Intraoperative Transesophageal Echocardiography. 2nd ed. Philadelphia, PA: Wolters Kluwer/Lippincott Williams & Wilkins; 2011:3-41.
A.7. What are the three TEE vantage points for the comprehensive imaging of the LV? How are pressure gradients measured by echocardiography? How do the pressure gradients derived from Doppler echocardiography differ from those obtained in the catheterization laboratory by direct pressure measurement?
The heart has two main axes: the longitudinal axis running from the base to the apex and the short axis perpendicular to that. Multiple scan planes are required to completely image a 3D structure, such as the heart. The LV is divided into 17 segments: 6 at the basal level, 6 at the midpapillary level, 4 at the apical level, and 1 apical cap (Fig. 7.13). By moving the TEE probe in the esophagus and rotation of the crystal within the transducer tip, the LV can be imaged from three acoustic windows. These are the midesophageal four-chamber, the transgastric short axis, and the transgastric two-chamber view. Normal ventricular motion requires that each wall segment thickens and moves toward the LV cavity at systole. Segmental wall function is usually quantified as normal, hypokinesis, akinesis, and dyskinesis.
In addition to function, TEE permits the determination of wall thickness and chamber size—important parameters in understanding the pathophysiology of valvular heart disease.
As mentioned previously, pressure gradients are derived through analysis of the Doppler profiles of blood flow. A commonly determined echocardiography gradient is that which is present from the LV to the aorta in the setting of AS. To obtain the change in blood flow velocity across the AV with TEE, the probe is advanced far into the stomach and sharply anteflexed and left deflected to obtain the window from the apex of the heart (deep transgastric long axis) and align the ultrasound beam most parallel to the path of blood flow. From this window, the continuous-wave Doppler cursor is directed across the LV outflow tract and AV. An example of such a spectral Doppler display is seen in Figure 7.14. The large increase in blood flow velocity in this display occurs at the narrowest point along its path, which, in this case, is the stenosed orifice of the AV. Using the modified Bernoulli equation mentioned previously, a gradient is calculated (100 mm Hg in the example). This represents the maximum instantaneous systolic pressure difference between the LV and the aorta. AS is also quantified at the time of catheterization
by measuring the pressures from within the LV, and the aorta as a rapid response pressure transducer catheter is withdrawn from the LV back to the aorta across the stenotic valve. The standard reported pressure gradient is the difference between the maximum LV and aorta pressures. Figure 7.15 illustrates that these peaks are not simultaneous events. Therefore, Doppler-derived AS gradients are usually higher than those derived at the time of left heart catheterization.
by measuring the pressures from within the LV, and the aorta as a rapid response pressure transducer catheter is withdrawn from the LV back to the aorta across the stenotic valve. The standard reported pressure gradient is the difference between the maximum LV and aorta pressures. Figure 7.15 illustrates that these peaks are not simultaneous events. Therefore, Doppler-derived AS gradients are usually higher than those derived at the time of left heart catheterization.

Full access? Get Clinical Tree
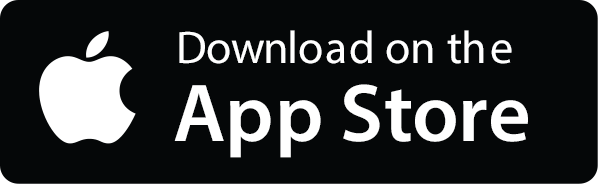
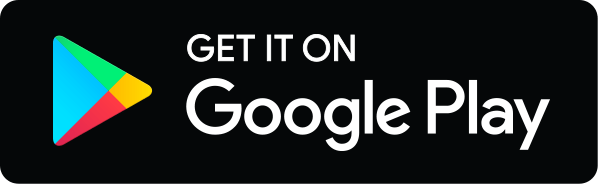