Ultrasonography in Emergency Cardiovascular Care
Anthony J. Dean
Sarah A. Stahmer
Ultrasonography is rapidly available, noninvasive, and can be performed at the bedside in the emergency department. When performed by trained and skilled individuals, it can speed diagnosis in the critically ill or arrested patient, allowing for targeted interventions.
Use in undifferentiated hypotension
An adjunct to assessment and triage of chest pain and dyspnea
An aid to central access to the circulation
Rapid assessment for correctable causes of pulseless electrical activity
Introduction
Evaluation and management of the critically ill often necessitates rapid interventions initiated on the basis of a brief and limited assessment. Occasionally the history and physical examination are unequivocal; but for many patients with hemodynamic instability, the clinical evaluation is not diagnostic. The ultrasound evaluation in this setting will be directed to obtaining immediate anatomic and pathophysiologic data about the patient’s condition and response to therapeutic interventions. Cardiac assessment may include evaluation of function, chamber size, valves, and the pericardial space. The inferior vena cava (IVC) may provide information about volume status. The lungs and pleura can be checked for consolidations, effusions, and pneumothorax. The peritoneal cavity may be evaluated for hemorrhage, aortic disease, or gross visceral abnormalities. Each of these studies may be repeated, performed once only, or not at all, depending on the clinical scenario and the patient’s condition and course. Ultrasound will also be used in guiding invasive procedures.
In order to put this wide range of sonographic applications in context, the material in this chapter is presented through clinical scenarios. These are centered around four cardinal syndromes of the critically ill:
Shock/unexplained hypotension/circulatory collapse
Chest pain
Shortness of breath/respiratory insufficiency
Cardiac arrest
While technical aspects of some applications are discussed in detail, this chapter is not intended as a tutorial on sonography. For such information, the reader is directed to specialty-specific educational resources.

Bedside Ultrasound in the Evaluation of Undifferentiated Hypotension: Clinical Scenario
A 52-year-old man with end-stage renal disease who was last dialyzed the previous day presents with 6 hours’ severe chest pain, productive cough, shortness of breath, and subjective chills. He has a history of heavy smoking, hypertension, atrial fibrillation, and myocardial infarction. He is unable to provide a list of his medications. The patient appears diaphoretic and anxious, with pale extremities. BP 106/70, HR 82, RR 22, and oral temperature 100°F (37.8°C) (rectal temperature pending). His lungs reveal distant breath sounds with rhonchi. His heart sounds are also distant but regular, with a systolic murmur.
Indications for Sonography
The commonest mechanisms of shock are hypovolemic, cardiac, distributive, and mechanical. Current concepts of “septic” and “neurogenic” shock, both of which used to be considered as separate categories, are now recognized as special cases in which one or more of the first three are concurrent in a single patient. Thus the shock of sepsis (in varying combinations) may result from hypovolemia (secondary to dehydration and vasodilation), myocardial depression, and distributive mechanisms (secondary to vasodilation and microcirculatory dysregulation). Similarly, neurogenic shock arises from a combination of neurally mediated vasodilation and microcirculatory dysregulation, often compounded by bradycardia. Mechanical causes of shock [e.g., cardiac tamponade, tension pneumothorax, or massive pulmonary embolus (PE)] are especially important to consider in the sonographic evaluation of pulseless electrical activity because they may be readily treatable.
This patient with chronic hypertension almost certainly is relatively hypotensive. Potential causes in this patient would include infection with distributive shock and/or hypovolemic shock, myocardial infarction with cardiogenic shock, pericarditis with tamponade, and aortic dissection. In the evaluation and management of hypotension, invasive monitoring by central venous and pulmonary artery catheters has been the mainstay of diagnostic information about volume status, cardiac output and central venous oxygen saturation. However, invasive monitoring has associated risks and complications, and its clinical validity continues to be debated. In addition, central venous access requires allocation of precious time and human resources.
Focused, limited, clinician-performed bedside ultrasonography is noninvasive and can be rapidly deployed in a patient without invasive monitoring devices. It is directed to determining the type of the patient’s shock, its severity, and, where possible, its cause. Several causes (e.g., infarction, pneumothorax, and massive PE) are discussed elsewhere in this chapter. The following discussion focuses on the utility of ultrasound in determining the type of the patient’s shock and its severity, both of which form the basis of initial treatment decisions, including the choice between volume resuscitation, diuretics, or neither and the use of pressors of cardiac inotropes. In addition to guiding immediate fluid management, bedside sonography assesses cardiac function and the pericardial space.
Intravascular Volume Assessment
Volume assessment can be accomplished by evaluating the heart and inferior vena cava (IVC). An extensive body of literature describes a relationship between the sonographic appearance of the IVC and both intravascular volume and cardiac filling pressures.1,2,3,4,5 Assessment of the IVC is made both qualitatively (shape) and quantitatively (absolute size and collapse index). There is strong evidence that in a given patient, increasing intravascular volume will result in both an expanding IVC diameter and diminishing the percent variation in diameter related to the respiratory cycle (collapse index). Decreasing intravascular volume will have the opposite effects.1,3,4,4a,6 In healthy volunteers at rest, the expiratory diameter ranges from 15 to 20 mm and the inspiratory diameter from 0 to 14 mm.1,3 Since a wide range of normal and abnormal values for these parameters has been reported in the variety of patient groups and clinical settings where it has been investigated, it is likely that absolute, universally applicable cutoffs do not exist.3,7 This is consistent with the physiologic role of the IVC as a capacitance vessel that can buffer fluctuations of hydration. Thus the clinician should become familiar with the spectrum of IVC findings encountered in normovolemic patients and use this as a basis for the recognition of patients at extremes with either grossly distended or grossly underfilled cavae.
The absolute measurement of the IVC is often made at the maximal diameter (atrial contraction) in expiration, although some have used inspiratory diameters, and many studies do not specify. As noted, a range of “normal” IVC diameters has been reported, with those in the cardiology literature frequently larger than those in studies made of previously healthy patients with acute illnesses. This may be due to a higher prevalence of heart disease among patients in echocardiographic studies, many of whom require chronically elevated preload. The consensus that emerges is that an adequate central venous pressure (CVP) (for hearts not depending on increased preload) can be predicted by an IVC diameter of greater than 10 to 12 mm.4 Young, conditioned athletes will normally have significantly larger IVC.8 With an IVC diameter below 5 mm, abnormally low CVP becomes increasingly likely.
The IVC collapse index (IVC-CI) is defined by the following equation:
IVC-CI = (IVCD-exp – IVCD-insp) / IVCD-exp
See Figure 8-1.
![]() Figure 8-1 • IVC Dmax and Dmin should be measured during expiration and inspiration, respectively. They do not refer to beat-to-beat variation of IVC diameter. |
Where IVCD-insp is the IVC diameter in inspiration and IVCD-exp is the IVC diameter in expiration (see Figs. 8-2 and 8-3 and Video Clip 8-1). Again, precise cutoffs between abnormal and normal are not available. However, the closer that the IVC-CI approaches either 0% or 100%, the more likely that the patient is volume overloaded or depleted, respectively.9,10,11 IVC-CI in normal healthy volunteers has been reported in the range of 25% to 75%.1,3 In hypovolemic patients with slit-like IVCs it is sometimes necessary to give primary consideration to the absolute IVC diameter over IVC-CI. For example, a patient with a maximum IVCD-exp of 4 mm that collapses to a minimum IVCD-insp of 2 mm is volume-depleted regardless of the normal IVC-CI of 50%. Positive-pressure ventilation, by reversing the usual pressure changes through the respiratory cycle, might be expected to undermine the utility of IVC-CI assessment.5,10,12,13,14,15 Studies have not been consistent on this subject, although several have confirmed that maximal IVC diameter will be found in inspiration in ventilated patients.12,13 These studies also suggest that the collapse index in ventilated patients is much lower than that commonly encountered in unvented patients, so that any IVC-CI >10% to 20% may be an indicator of fluid-responsive shock.12,13 The lack of consensus and the findings of these reports suggest that caution is needed in interpretation of IVC findings in ventilated patients.
Video Clip 8-1. This clip shows a subxiphoid view of the IVC shown in Figure B. The biphasic beat to beat fluctuations in IVC diameter reflect the classically described central venous pressure waveform. They may not always be this clear. The ability to image the vessel both longitudinally and transversely, as in this case, is ideal (see text).
Several slightly different techniques for IVC-CI measurement have been described, using either normal respirations or a forced sniff (both difficult to standardize). Measurements are often made in diastole (i.e., the moment when the IVC is smallest in the cardiac beat-to-beat cycle) and taken just inferior (1–3 cm) to the junction of the hepatic veins. The traditional window has been subxiphoid, although recent reports have suggested that equivalent results can be obtained using a right intercostal view with the liver as a sonographic window.16,17 This approach is highly useful, since many critically ill patients may have the subxiphoid view obscured by bowel gas or dressings.18 In addition, transducer placement in the epigastrium could theoretically cause artifactual narrowing of the IVC by probe pressure—a concern that is obviated by intercostal probe placement. Longitudinal and transverse planes are equivalent for measurement of the IVC.19 However, each plane has intrinsic advantages and disadvantages, many of which are mutually complementary, so that experienced sonographers usually perform assessment in both planes. This allows for a qualitative estimate of the size and dynamics of the IVC, which, with experience, often gives a better sense of intravascular volume than a raw number (see Video Clip 8-2).
Video Clip 8-2. If the B-mode image (on the right of the screen) were frozen and used as the sole determinant of the patients intravascular volume status, it might lead to an underestimation of her fluid needs. Measurements should always be taken in the context of the gross appearance of the vessel, which in this case is clearly underfilled.
The commonest and most serious pitfall in IVC evaluation is the misidentification of the vessel. Experienced sonologists are familiar with cases in which all the elongated fluid-filled structures in the upper abdomen (aorta most commonly, but also gallbladder, hepatic veins, portal vein, and pleural effusions) have been mistaken for the vein. This is especially likely to occur in cases where severe hypovolemia renders the slit-like IVC almost invisible (see Fig. 8-4 and Video Clip 8-3).
Color-flow may help in elucidation, but more often a reliable evaluation IVC depends on the following:
Color-flow may help in elucidation, but more often a reliable evaluation IVC depends on the following:
![]() Figure 8-4 • This figure demonstrates a classic pitfall of overlooking the IVC (arrow in B-mode, arrowheads in M-mode on right of image) when it is small, and instead measuring the aorta (shown also in Video Clip 8-3). The IVC often appears to overlie the aorta in the intercostal window, as in this case. Misidentification can be avoided by positively identifying both vessels in every patient. |
Video Clip 8-3. In this intercostal view (see accompanying Figure D), the sonologist is mistakenly concentrating on the aorta and overlooking the much less prominent IVC that can be seen coming in and out of plane in front of (closer to the probe) the artery.
Positive identification of both aorta and IVC in the transverse plane
Positive identification of the IVC entering the right atrium (longitudinal and transverse planes)
Experience and familiarity with the technique
IVC-CI measurements are expected to be less reliable in ventilated patients, as previously noted. Positive-pressure ventilation, by creating a gradient between thoracic and abdominal compartments, is also likely to result in an artifactually dilated IVC. In such patients a well-filled IVC should be interpreted with caution, and an IVC <12 mm is highly likely to be a sign of intravascular hypovolemia.14,15 Overestimation of intravascular volume status may also occur with any condition that impedes forward flow through the right heart. These include tricuspid regurgitation or stenosis, pulmonic stenosis, pulmonary hypertension (including hemodynamically significant pulmonary embolism; see above), right ventricular infarct, or severe left-sided failure. Young, healthy athletes have large and dilated IVCs, so that a “mildly collapsed” IVC in such a patient might represent a state of significant hypovolemia.8 Underestimation of intravascular volume will occur with extrinsic compression of the IVC. Tense ascites, which is usually clinically apparent, is a common cause.20 Hepatomegaly and retroperitoneal lymph nodes are less common but usually sonographically evident in the longitudinal view.
Cardiac Function
Several studies have shown that bedside ultrasonography provides estimates of ejection fraction (EF) that correlate as closely with the reading of an experienced echocardiologist as do the readings of two echocardiologists compared with one another.21,22,23,24,25,26 For the purposes of clinical decision making with an acutely ill patient, EF can be divided into four broad categories: “abnormally high” (>75%), “normal” (50%–75%), “moderately depressed” (30%–50%), and “severely depressed” (<30%) (see Video Clips 8-4, and 8-5a and b, 8-6, 8-7a and b, and 8-8). “Abnormally high” EF suggests intravascular hypovolemia with an underfilled heart, although it can also be seen with hypertrophic cardiomyopathy. This finding should be correlated with the findings of the IVC evaluation. An exam showing severely depressed myocardial contractility will mandate caution in the administration of fluids and may prompt consideration of diuresis if the heart is severely dilated as well as the initiation of inotropic therapy, early percutaneous transluminal coronary angioplasty (PTCA), and/or placement of an intra-aortic balloon pump (IABP). Conversely, demonstration of mildly depressed, normal, or hyperdynamic cardiac function excludes acute myocardial dysfunction as a primary cause of hypotension. Caution should be observed in ascribing hypotension to moderately depressed contractility, since these patients usually have chronic and compensated cardiomyopathy, so that acute hypotension usually betokens an acute and unrelated process such as sepsis or dehydration.
Video Clip 8-4. Severely depressed EF/perfusing rhythm. This image was obtained from the parasternal window and demonstrates a heart with significant bi-ventricular hypokinesis. This patient was still able to generate a blood pressure with this degree dysfunction with a left ventricular ejection fraction of less than 10%.
Video Clips 8-5 a and b. The parasternal long axis (PSLA) view appears to show complete end-systolic collapse. This finding needs to be confirmed by short axis (PSSA) views, since it can be caused by the ventricular cavity moving off-plane during systole. The PSSA shows an abnormally high EF of around 80% with the thickened ventricular walls expected in an underfilled heart, but does not show complete systolic collapse.
Video Clip 8-6. This clip shows both parasternal long and short axis views in a vigorously contracting heart with an EF of around 70%. There is a small but circumferential effusion.
Video Clips 8-7 a and b. Parasternal long and short axis views of a heart with an EF of around 30%.
Video Clip 8-8. This is the parasternal long axis view of dilated cardiomyopathy with an EF of < 10%. The ventricular walls have become effaced through the dilatation, and a small effusion is present.
Cardiac Tamponade
Common nonsurgical causes of tamponade include neoplastic, idiopathic, and uremic effusions.27 Many pericardial effusions are clinically silent until they cause symptoms of tamponade, the physical findings of which (pulsus paradoxus and Beck’s triad) are unreliable and late findings.27,28,29,30,31 Fortunately, ultrasonography accurately detects pericardial fluid and identifies findings of tamponade early. Various categorizations of effusion have been proposed. That of the European Society of Echocardiography distinguishes “small” (often physiologic, <10 mm maximal thickness in diastole), “moderate” (10–20 mm maximal thickness in diastole), “large” (>20 mm maximal thickness), and “very large” (>20 mm maximal thickness with cardiac compression).27 The problem with this classification system is that it is most applicable to patients with long-standing or slowly evolving pericardial effusions. Rapidly forming pericardial effusions can cause tamponade physiology with circumferential effusions of less than 10 mm, especially in patients with cardiomyopathy dependent on high filling pressures.32,33 Thus, in assessing an acutely unstable patient without the benefit of prior studies or known cardiac history, the clinician may be better served by the categories of “noncircumferential/ physiologic” (see Fig. 8-5 and Video Clip 8-9); “circumferential, <10 mm maximal thickness” (see Fig. 8-6 and Video Clip 8-6); and “circumferential, >10 mm maximal thickness” (see Fig. 8-7 and Video Clip 8-10), with a further determination made in each case as to the presence or absence of tamponade (Fig. 8-8 and Video Clips 8-11 and 8-12).
Video Clip 8-9. This noncircumferential effusion seen on the subxiphoid view is larger than most physiological effusions, and probably represents some inflammatory process in this sickle cell patient with chest pain.
Video Clip 8-10. This parasternal long axis shows a very large pleural effusion without evidence of tamponade.
Video Clip 8-11. This apical 4-chamber image demonstrates a large circumferential pericardial effusion with pronounced diastolic collapse of the right atrium, and more subtle diastolic collapse of the apex of the right ventricle.
Tamponade occurs when the intrapericardial pressure equals or exceeds diastolic filling pressures. The more rapid the accumulation of pericardial fluid, the smaller the volume necessary to cause tamponade.27,32,33 Intrapericardial pressure can rise abruptly with the acute accumulation of as little as 80 to 200 mL of fluid.32 Right ventricular diastolic
collapse (RVDC) has been shown to be the most accurate finding in tamponade (Fig. 8-8 and Video Clips 8-11 and 8-12).34,35 Right atrial collapse is more sensitive but less specific.35,36 Left ventricular collapse may also occur.35,37 Subxiphoid and apical four-chamber windows provide good views of the right heart, although the former may be easier to obtain. If cineloop is available, a good image should be obtained, frozen, and the motion of the right ventricular free wall carefully reviewed frame by frame, since RVDC may be fleeting in the tachycardic heart.
collapse (RVDC) has been shown to be the most accurate finding in tamponade (Fig. 8-8 and Video Clips 8-11 and 8-12).34,35 Right atrial collapse is more sensitive but less specific.35,36 Left ventricular collapse may also occur.35,37 Subxiphoid and apical four-chamber windows provide good views of the right heart, although the former may be easier to obtain. If cineloop is available, a good image should be obtained, frozen, and the motion of the right ventricular free wall carefully reviewed frame by frame, since RVDC may be fleeting in the tachycardic heart.
![]() Figure 8-6 • This parasternal short axis view shows a moderate circumferential effusion (arrows) with measured thickness of 9.4 mm. |
Video Clip 8-12. The swinging of the heart within the massive pericardial effusion every two cardiac cycles is the physical basis of electrical alternans. The wall of the right ventricle in this apical four chamber view (on the left of the image) can be seen to collapse in diastole.
False-positive diagnoses of pericardial effusions have been caused by misidentification of epicardial fat, pleural effusions, and vessels posterior to the heart (e.g., descending aorta, pulmonary vessels, or coronary sinus).38 Epicardial fat is noncircumferential, localized, has an irregular outline, tends to lie along the paths of the coronary vessels (interventricular and interatrial grooves), and with optimal gain adjustment demonstrates internal echoes (see Video Clip 8-13). In contrast to effusions it usually gets thinner towards the apex. The distinction between pericardial and pleural fluid can be made by the conforming shape of the pericardial sac. Such an apparently obvious distinction may be challenging in the setting of an unstable patient with limited time, suboptimal cardiac windows, and poor patient positioning. Efforts should be made to image the fluid in the axillary or scapular lines, looking for the characteristic wedge shape of pleural fluid in the costophrenic sulci. In parasternal views, pericardial fluid will appear anterior to the descending aorta, while pleural fluid will appear to be surrounding or posterior to it (Video Clip 8-14). The descending aorta itself can be mistaken for pericardial fluid when seen longitudinally, but its tubular structure should be apparent in an orthogonal plane (Video Clips 8-15a and b).
![]() Figure 8-7 • This apical four chamber view in a patient with chronic liver failure shows a massive circumferential effusion of up to more than 3 cm in thickness. |
Video Clip 8-13. In this suboptimal quality parasternal long axis view, epicardial fat can be distinguished from effusion by its internal echoes, and its irregular outline which tapers towards the apex.
Video Clip 8-14. In this limited study, the fluid behind the heart might be mistaken for pericardial effusion, with the right ventricle (above the left ventricle on the image) as the anterior extension of this effusion. Careful attention to the contracting parts allows for correct identification of the right ventricle, while the non-conforming shape of the pleural fluid below the heart (on the image) identifies it as such.
Video Clips 8-15 a and b. Clip a shows a longitudinal image of the descending aorta behind a parasternal short axis view of a hyperdynamic heart. Clip b shows a short -axis view from another angle in which a non-circumferential pericardial effusion can be seen in front of a region of pleural fluid, with the transverse view of the descending aorta appearing next to (towards right on screen) the vertebral bodies.
Severe hypovolemia can also cause diastolic ventricular collapse, but other clinical findings usually clarify the situation.33,39 If there is any doubt, the IVC should be checked, and if it is not plethoric and with minimal or no respiratory collapse, tamponade is excluded. Conversely, tamponade may occur without RVDC in conditions causing elevated right heart pressures (e.g., pulmonary hypertension).36 The “sniff test” may help to identify tamponade in this setting: the IVC should collapse by 50% or more of its diameter with sudden inspiration, but will fail to do so with tamponade. Absence of IVC collapse with “sniff” is highly sensitive for tamponade, but since it occurs in many other conditions, it is very nonspecific.
Acutely clotting blood (usually in traumatic tamponade) may be mistaken for thickened myocardium, although gain
optimization reveals heterogeneous echodensities reflecting blood at different stages of thrombosis (Fig. 8-9).40 While there are a number of echocardiographic findings that, considered in isolation, can be misleading, their significance is usually clarified by consideration of the clinical context (Fig. 8-8).
optimization reveals heterogeneous echodensities reflecting blood at different stages of thrombosis (Fig. 8-9).40 While there are a number of echocardiographic findings that, considered in isolation, can be misleading, their significance is usually clarified by consideration of the clinical context (Fig. 8-8).
Bedside Ultrasound in the Evaluation of Acute Chest Pain: Clinical Scenario
A 76-year-old presents to the ED complaining of approximately 2 hours’ midsternal pressure and shortness of breath. He has no prior cardiac history. At one point he felt as if he were going to “pass out.” He smokes and has mild hypertension. His vital signs reveal a BP of 148/88 mm Hg, a pulse of 88, and a pulse oximetry of 98%. His exam is remarkable only for his anxiety, discomfort, and pallor. His electrocardiogram (ECG) with ongoing symptoms shows a sinus rhythm with left ventricular hypertrophy (LVH) and nonspecific ST-T wave abnormalities. The patient has no prior ECGs for comparison.
Indications for Sonography
In view of the multiple risk factors, this patient’s symptoms are probably due to acute coronary syndrome (ACS). Other, less likely causes of chest pain include aortic dissection, pulmonary embolism, spontaneous pneumothorax, and pneumonia. The latter three are discussed in the section on the evaluation of acute dyspnea. This section considers the use of bedside ultrasound in the evaluation of acute ischemia and dissection.
Assessment of Cardiac Ischemia
Several studies have evaluated the ability of echocardiography to stratify patients presenting with acute chest pain according to their risk for cardiac events.41,42,43 The overall predictive value of a positive echocardiogram (identification of wall motion abnormalities) varies with disease prevalence in the study population. In studies where the observed cardiac event rate is high, the predictive value of a positive study is 90% or greater.44,45,46,47 Conversely, the absence of wall motion abnormalities predicts that the likelihood of infarct or active ischemia is low but not so low as to reliably exclude the presence of acute ischemia with negative predictive value (NPV) ranging from 57% to 93%. In groups of low-risk patients with overall event rates of less than 20%, a positive echocardiogram is less predictive of subsequent cardiac events, with positive predictive value (PPV) ranging from 34% to 44%.41,42,48 In this group, the absence of regional wall motion abnormalities identifies low-risk patients, with an NPV of 93% to 98%. Although reassuring, this may still not be sufficient to allow safe discharge from the ED. Thus, a resting echocardiogram cannot be used alone as the basis for decisions about the disposition of patients with possible ischemic chest pain.
The American College of Cardiology (ACC)/American Heart Association (AHA) guidelines on the clinical use of echocardiography give the diagnosis of suspected acute ischemia or infarction a class I recommendation for patients with suspected acute myocardial ischemia when baseline ECG and laboratory markers are nondiagnostic and when the study can be performed during pain or within minutes of its abatement.49,50 The studies of transthoracic echocardiography (TTE) in the evaluation of chest pain were performed and interpreted by trained echocardiologists with American Society of Echocardiography level 3 training, a requirement that, given the complexity of wall motion analysis, is unlikely to change. These conditions are met only at the minority of institutions in which echocardiologists are available on a stat basis for image interpretation at all times.51
TTE is directed to the detection of segmental wall motion abnormalities, which occur at the onset of myocardial ischemia and may precede clinical symptoms and ECG changes. The location of these abnormalities correlates well with the vessel involved and will reflect changes in regional perfusion in response to therapy.52,53

Full access? Get Clinical Tree
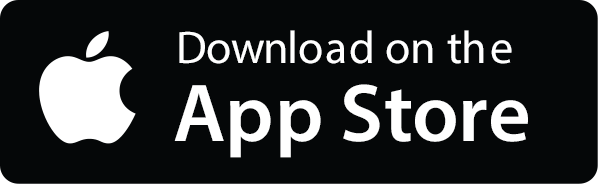
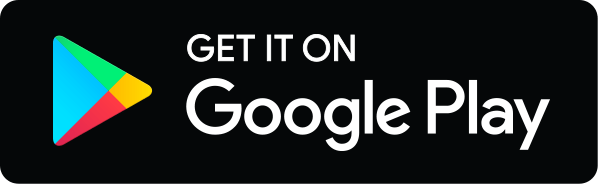
