Fig. 12.1
Modified comparative composition of pain distribution in the cervical region provoked by injections of hypertonic saline in to the interspinous ligaments (a) Feinstein et al. [2]. Synovial joints: (b) (c) Significant overlap of these pain maps is due to the fact that injected structures are innervated by the cervical dorsal rami specifically the medial branches (MBDR). Similar relations exist in the thoracic, lumbar, and sacral regions (With permission from Dwyer et al. [3]; and Dreyfuss et al. [4])
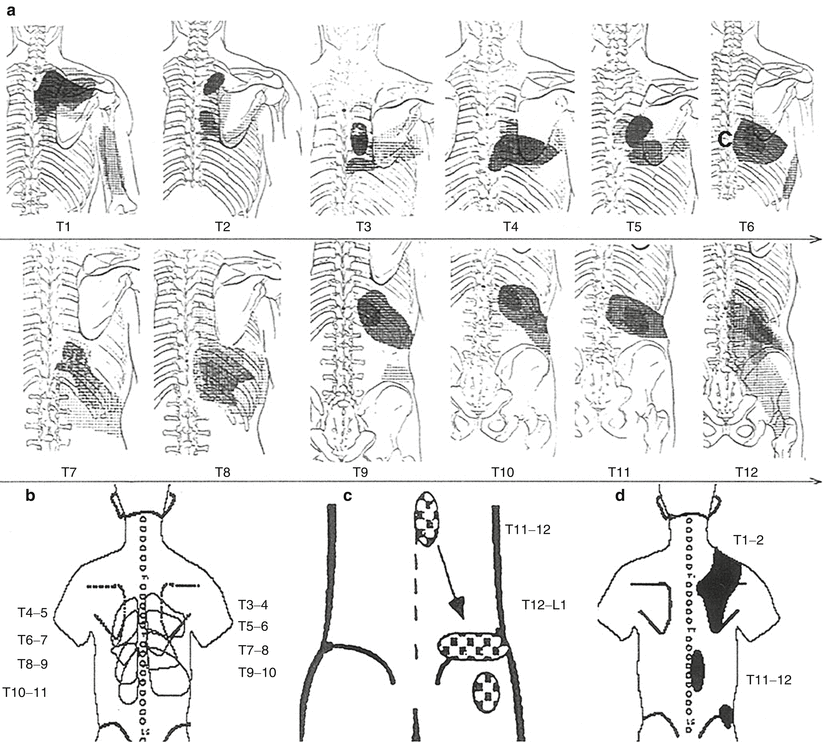
Fig. 12.2
A modified, comparative composition of pain distribution in the thoracic region provoked by injections of hypertonic saline into the interspinous ligaments by Feinstein et al. [2] (Upper two rows – a) and thoracic Z-joints (b) by Dreyfuss et al. [4], (c) by Dussault and Kaplan [5], and (d) by Fukui et al. [6]. Significant resemblance of the pain patterns and their overlaps is due to the fact that injected structures receive the same segmental innervated by the thoracic dorsal rami specifically the medial branches (MBDR)
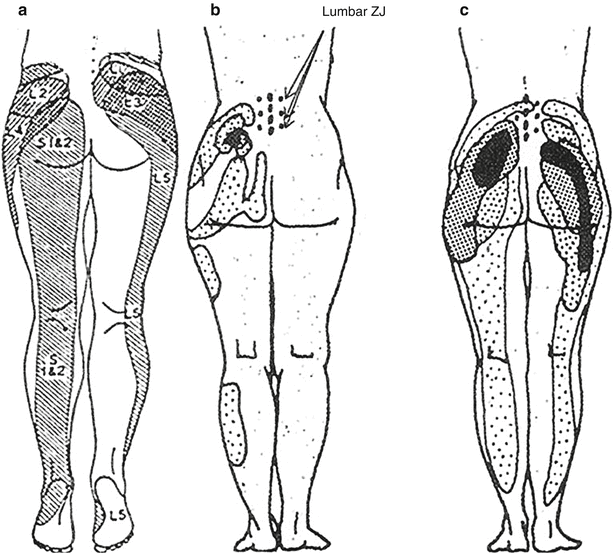
Fig. 12.3
Modified comparative composition of pain distribution in the lumbar region provoked by injections of hypertonic saline into the (a) lumbar interspinous ligaments dots in the midline from Kellgren et al. [7], from lumbar Z-joints, Mooney and Robertson [8] (b), and from asymptomatic subjects (c) of symptomatic patients (paravertebral dots); significant resemblance of the pain patterns and their overlaps is due to the fact that injected structures receive the same segmental innervated by the lumbar dorsal rami specifically the medial branches (MBDR)
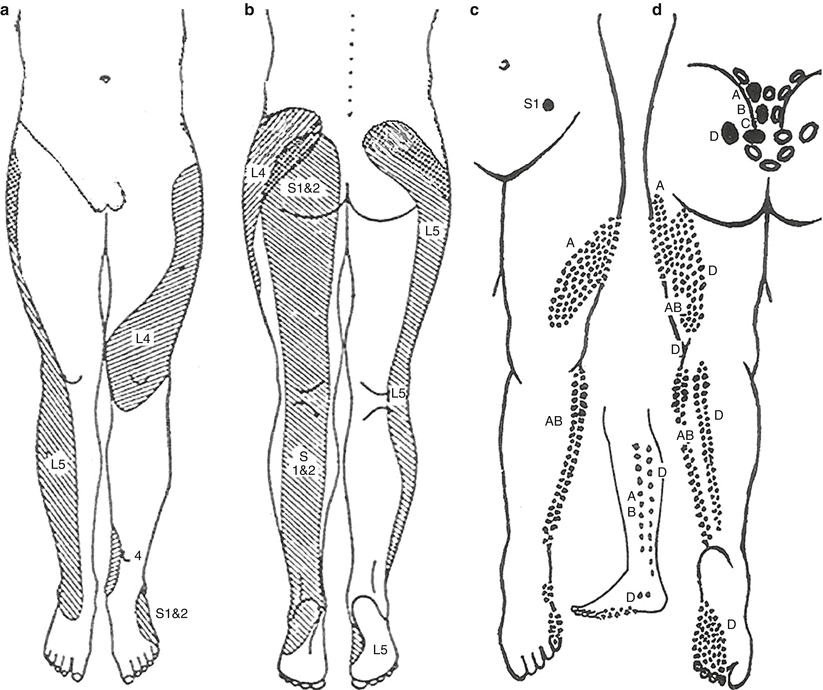
Fig. 12.4
Modified comparative composition of pain distribution from lumbosacral region provoked by injections of hypertonic saline into the (a, b) interspinous ligaments from L4–5 to S1–2 from Kellgren et al. [7]. (c, d) Referred pain maps from posterior sacroiliac ligament enthesopathies and sacroiliac joint instability (AB from the upper fibers, CD lower fibers ileum and sacrum) (Reproduced from Hackett [9]). Hackett published these maps after abolishing pain with local anesthetic infiltration in more than 7,000 injections over 17 years. Significant resemblance of the pain patterns and their overlaps is due to the fact that injected structures receive the same segmental innervated by the lumbar dorsal rami (Prepared for publication by Felix Linetsky M.D.)
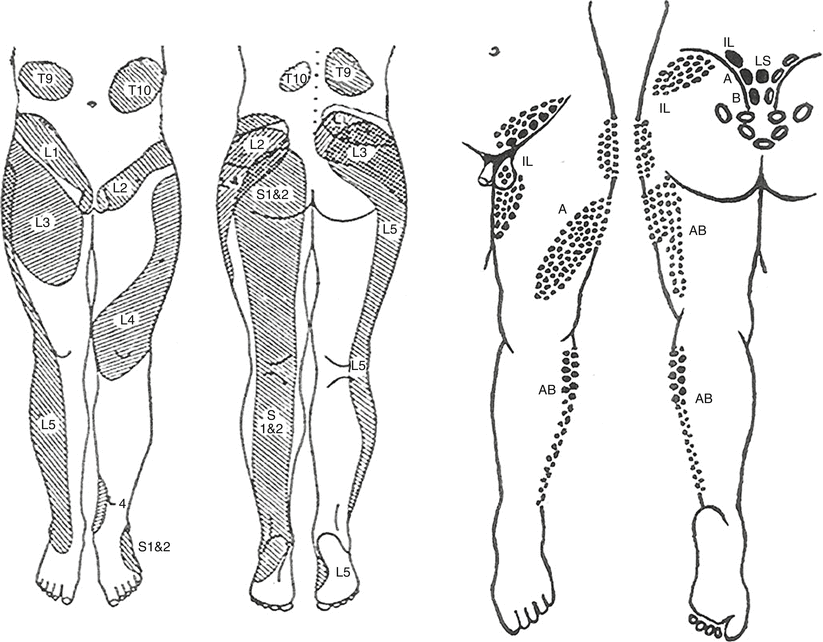
Fig. 12.5
Modified comparative composition of pain distribution from lumbosacral region provoked by injections of hypertonic saline into the (a, b) interspinous ligaments from L1–2 to S1–2 from Kellgren et al. [7]. (c, d) Trigger areas and referred pain from iliolumbar (IL) and posterior sacroiliac (upper AB) ligaments (lumbosacral (LS) and sacroiliac joint instability). Hackett published these maps after abolishing pain with local anesthetic infiltration in more than 7,000 injections over 17 years. Significant resemblance of the pain patterns and their overlaps is due to the fact that injected structures are innervated by the same segmental lumbar dorsal rami (From Hackett [9]. Prepared for publication by Felix Linetsky M.D.)
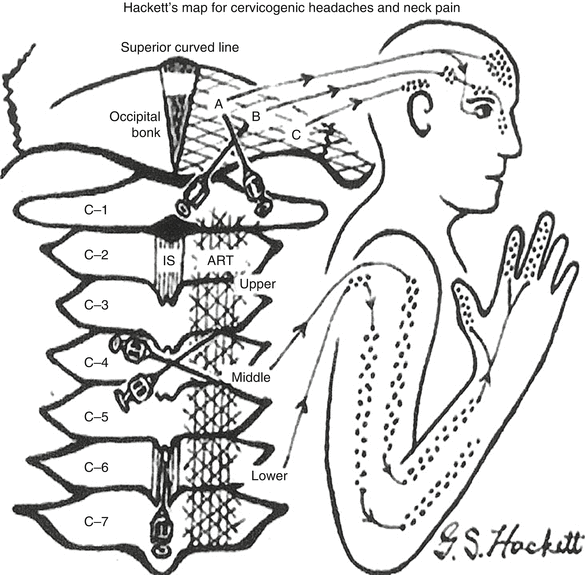
Fig. 12.6
Trigger areas and needle positions for diagnosis and treatment of cervical enthesopathies with small fiber neuropathies and neuralgias with their respective referral pain maps from ligaments and tendons. (A–C) Between superior and inferior nuchal lines. ART ZJ articular ligaments and periarticular tendons, IS Interspinous ligaments (From Hackett [9])
Evolution of Terminology
Prior to 1930s, this treatment was called “injection treatment” with addition of a pathologic descriptor such as of injection treatment of varicose veins or injection treatment of hydroceles [17]. Biegeleisen coined the term “sclerotherapy” in 1936 [18].
Concluding that sclerotherapy implied scar formation, Hackett coined the term prolotherapy as “the rehabilitation of an incompetent structure by the generation of new cellular tissue.” Hackett’s supposition that “… prolotherapy is a treatment to permanently strengthen the ‘weld’ of disabled ligaments and tendons to bone” led to treatment with injections at the fibro-osseous junctions [11]. More recent work found significant amount of degenerative changes in the midsubstance of the ligaments and tendons as well as ruptures at the fibro-muscular interfaces, and intersubstance changes.
Further, current understanding of the basic science is such that regeneration and repair extend beyond the proliferative stage which is only a short phase of the healing process. More so, proliferation is an integral part of a malignant unsuppressed growth as well as degenerative changes which are present in the bones, synovium, intervertebral discs, ligaments, tendons, and fascial connective tissues. Regenerative injection therapy was coined by Dr. Linetsky because it is a more appropriate nomenclature for the treatment modality which promotes natural healing [1, 19–22].
Local Anesthetics in the Diagnosis of Musculoskeletal Pain
Differential diagnosis of musculoskeletal pain based on infiltration of procaine at the fibro-osseous junctions was pioneered in the 1930s by Leriche [16, 19, 22]. Steindler and Luck described that posterior primary rami provide sensory supply to muscles, tendons, thoracolumbar fascia, ligaments, and aponeuroses and their origins and insertions; therefore, no definite diagnosis could be made based on clinical presentation alone. They established the following criteria to prove a causal relationship between the structure and pain symptoms: reproduction of local and referral pain by needle contact, suppression of local tenderness, and referral/radiating pain by procaine infiltration [23]. Haldeman and Soto-Hall [24] infiltrated procaine in to posterior sacroiliac and interspinous ligaments, zygapophyseal joint capsules producing a field block with a marked relaxation of spastic musculature facilitating a routine use of sacroiliac and facet joint manipulations. They have introduced manipulation of axial joints under local anesthesia [24].
Anatomic Biomechanical and Pathologic Considerations
Ligaments are dull white, dense connective tissue structures that connect adjacent bones. They may be intra-articular, extra-articular, or capsular. Collagen fibers in ligaments may be parallel, oblique, or spiral, each of these orientations contains specific cross-linking formations. Such orientations represent adaptation to specific directions in restriction of joint displacements. Under a light microscope, ligaments have a crimped, wavelike appearance which unfolds during initial loading of collagen [22, 26–28]. When elongated up to 4 % of original length, ligaments and tendons return to their original crimped wave appearance. Beyond 4 % of elongation, they lose elasticity and become permanently laxed, causing joint hypermobility. In degenerated ligaments, subfailure was reported at earlier stages of elongation. At its best, natural healing may restore connective tissue to their pre-injury length, but only 50–75 % of its pre-injury tensile strength [22, 27–30].
There are three types of nerve terminals in posterior spinal ligaments: free nerve endings and the Pacini and the Ruffini corpuscles. A sharp increase in the quantity of free nerve endings at the tips of lumbar spinous processes was documented (Fig. 12.7) [29].
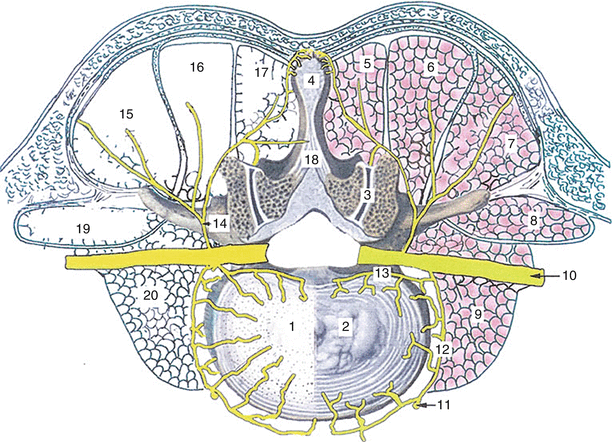
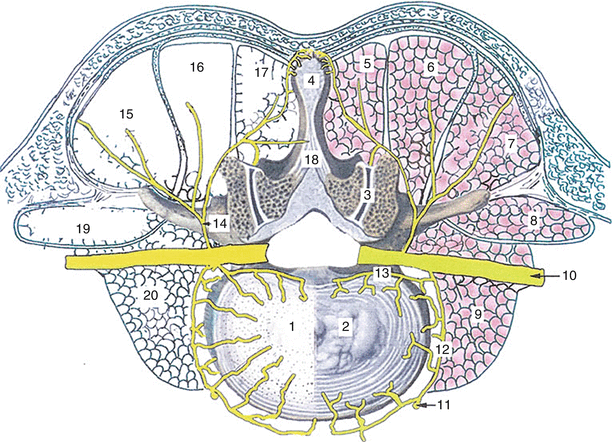
Fig. 12.7
Cross-sectional semi-schematic drawing of lumbar area illustrates 1 vertebral body, 2 intervertebral disc, 3 zygapophyseal joint (ZJ), 4 spinous process, 5 multifidus, 6 longissimus thoracis, 7 iliocostalis lumborum, 8 quadratus lumborum, 9 psoas major, 10 ventral ramus, 11 sympathetic trunk, 12 gray ramus communicant, 13 sinuvertebral nerve, 14 dorsal ramus, 15 lateral branch of the dorsal ramus (LBDR) in longissimus thoracis compartment, 16 intermediate branch of the dorsal ramus (IBDR) in iliocostalis lumborum compartment, 17 medial brunch of the dorsal ramus (MBDR) in multifidus compartment, 18 interspinous ligament, 19 quadratus lumborum compartment, and 20 psoas major compartment. MBDR innervates ZJ, multifidi, and interspinous ligaments and forms a several fold increase of the free unmyelinated nerve fibers at the tips of the spinous processes (Modified from Sinelnikov [31]. Modified and prepared for publication by Tracey James. All rights reserved. No part of this picture may be reproduced or transmitted in any form or by any means without written permission from Felix Linetsky M.D.)
Collagenous tissues are deleteriously affected by nonsteroidal anti-inflammatory drugs (NSAIDs), steroid administrations, inactivity, and denervation. A single corticosteroid injection into a ligament or tendon has been reported to have debilitating effects on the strength of collagen contained therein [27].
In the presence of repetitive microtrauma with insufficient time for recovery, use of NSAIDs and steroids, tissue hypoxia, metabolic abnormalities, and other less defined causes, connective tissues lose their homeostasis and cycle toward an accelerated degenerative pathway [17, 22, 27, 30, 32–34]. Therefore, a cautious use of anti-inflammatory therapy continues to be a useful, but an adjunctive, therapy [32]. It should be noted that unless homeostasis is reestablished in a joint which the ligament protects, further progressive degenerative changes occur with time when continued laxity is present. A well-known example of this is the development of osteoarthrosis in the knee joint following ACL injury with associated laxity of the joint capsule.
As opposed to ligaments, tendons are glistening whitish collagenous bands interposed between muscle and bone that transmit tensile forces during muscle contraction. There are considerable variations in shape and structure of fibro-osseous attachments and myotendinous junctions. A normal tendon with a cross section of 10 mm in diameter can support a load of 600–1,000 kg [22, 26, 33].
Collagenous tissue response to trauma is inflammatory/regenerative/reparative in nature and varies with the degree of injury. In the presence of cellular damage, regenerative pathway takes place; in the case of extracellular matrix damage, a combined regenerative/reparative pathway takes place. Both are controlled by hormones, chemical, and growth factors [17, 22, 27, 30, 32–34]. Central denervation, such as in quadriplegia, paraplegia, or hemiplegia, leads to a statistically high, accelerated tendon degeneration [33]. Radiofrequency procedures may not be an exception. Corticosteroids do not arrest or slow the course of degenerative process. Neoneurogenesis and neovasculogenesis are also integral components of degeneration.
The presence of vascular and neural ingrowth into degenerated intervertebral discs, posterior spinal ligaments, the hard niduses of fibromyalgia, and tennis elbow tendinopathies have been known for some time. Presence of neuropeptides in the facet joint capsules and articular and periarticular tissue of the sacroiliac joints with the absence of inflammatory markers are also well established, rendering the aforementioned structures nociceptive; nonetheless, corticosteroid injections are still the advocated therapeutic interventions [35–39].
More recently, research dedicated to sports medicine shed light on degenerative changes in tendinosis and tendinopathy as a distinct pathologic and clinical entity [40]. The neurovascular ingrowth was studied extensively in Achilles, patellar, and supraspinatus tendinosis. Intratendinous microdialysis of these tendons found normal prostaglandin E2 (PGE2) levels in chronic painful tendinosis. Analyses of biopsies showed no upregulation of pro-inflammatory cytokines. The neurotransmitter glutamate, a potent modulator of pain in the central nervous system, was found in tendinosis. Microdialysis demonstrated significantly higher glutamate levels in chronic painful tendinosis in comparison with pain-free control tendons [41–44]. Significantly, higher lactate levels were found in chronic painful tendinosis in comparison with pain-free normal tendons, implicating either hypoxia or a higher metabolic rate in pathophysiology of tendinosis [45].
Biopsies from the areas with tendinosis and neovascularization followed by immunohistochemical analyses of specimens showed substance P (SP) in the nerves juxtapositioned to the vessels and in the nervi vasorum together with calcitonin gene-related peptide (CGRP) juxtapositioned to the vascular walls [46, 47]. The neurokinin-1 receptor (NK-1R), that is known to have a high affinity for SPP, has been found in the vascular wall [48]. The findings of neuropeptides indicate the presence of a so-called neurogenic inflammation mediated by (SP) – like neuropeptides. The use of diagnostic ultrasound is very helpful in evaluation of tendinosis and other musculoskeletal pathology and will be described under radiologic evaluation.
Rationale
The rationale for RIT in chronic painful pathology of ligaments and tendons evolved from clinical, experimental, and histological research performed for injection treatment of hydroceles and hernia. In hydroceles, hypertrophied subserous connective tissue layer reinforced capillary walls and prevented further exudate formation. The same principle is employed in the treatment of chronic bursitis. Conversely in hernias, proliferation and subsequent regenerative/reparative response lead to a fibrotic closure of the defect [17–22].
A similar ability to induce a proliferative regenerative repetitive response in ligaments and tendons was demonstrated in experimental and clinical studies, with a 65 % increased diameter of collagen fibers [18, 49–51]. Multiple recent studies demonstrated that injecting polidocanol in to the neovascularity proximal to Achilles, patellar, and supraspinatus tendinosis under color Doppler (CD) ultrasound guidance produced an ultrasound-documented resolution of tendinosis and neovascularity, allowing patients return to a full painless activities. Thus, the sclerosing agent acting directly on neovessels is capable of restoring connective tissue homeostasis by modulation of local hemodynamic [52–55].
Clinical Anatomy in Relation to RIT
The shape of a human body is irregularly tubular. This shape, cross-sectionally and longitudinally, is maintained by continuous compartmentalized fascial stacking that incorporates, interconnects, and supports various ligaments, tendons, muscles, neurovascular, and osseous structures. Collagenous connective tissues, despite slightly different biochemical content, blend at their boundaries and at the osseous structures, functioning as a single unit. This arrangement provides bracing and a hydraulic amplification effect to the muscles, increasing contraction strength up to 30 % (Fig. 12.7) [22, 26, 56–62].
Movements of the extremities, spine, and cranium are achieved through various well-innervated articulations, which are syndesmotic, synovial, and symphysial. For the ease of radiologic evaluation, spinal joints were allocated to the anterior, middle, and posterior columns. Syndesmotic joints are anterior and posterior longitudinal ligaments, anterior and posterior atlantooccipital membranes (ALL and PLL), supraspinous and interspinous ligaments (SSL and ISL), and ligamentum flavum (LF).
Symphysial joints are the intervertebral discs (IVD), which are absent at the cranio-cervical and sacral segments, but present from the sacrococcygeal segments caudally.
Spinal synovial joints are the atlantoaxial (AA), atlantooccipital (AO), zygapophyseal (ZJ), costotransverse (CTJ), and costovertebral (CVJ); sacroiliac (SI) joint is a combined synovial–syndesmotic joint [22, 26, 56, 57].
Differential diagnosis is based on understanding of the regional and segmental anatomy, pathology, as well as segmental, multisegmental, and intersegmental innervation of the compartments and their contents around the spine; this is provided by ventral rami (VR), dorsal rami (DR), gray rami communicants (GRC), sinuvertebral nerves (SVN), and the sympathetic chain (SC) (Fig. 12.7) [22, 26, 56, 57].
Lumbar interspinous ligaments receive innervation from the medial branches of the dorsal rami (MBDR). Three types of nerve terminals in posterior spinal ligaments have been confirmed microscopically. They are the free nerve endings and the Pacini and Ruffini corpuscles. These nerve endings arise from lumbar MB [29]. A sharp increase in the quantity of free nerve endings at the lumbar spinous processes attachments (enthesis) was documented, rendering them putatively nociceptive (Fig. 12.7) [29]. Experimental and empiric observations suggest that a similar arrangement exists at the cervical and thoracic spinous processes, especially at the C2, C7, and T1, rendering them putatively nociceptive (Fig. 12.8) [2, 10, 28, 56]. Willard demonstrated that cervical, thoracic, and lumbar MBs on their distal course are located very close to the bone descending to the very apex of the spinous process, innervating the multifidus and cervical interspinales muscles [28, 56]. A formal recent anatomic study by Zhang et al. reconfirmed these observations in the cervical region [62]. Proximal to the origin, cervical MB is located in the gutter formed by the neighboring ZJ capsules under the semispinalis capitis (SSCa) tendon and supplies twigs to ZJ capsules. Thereafter, MB continues dorsomedially supplying on its course the semispinalis cervices (SSCe) and SSCa. At the mid-lamina level, MB innervates the multifidi and continues adjacent to every spinous process bilaterally below C2 to become a
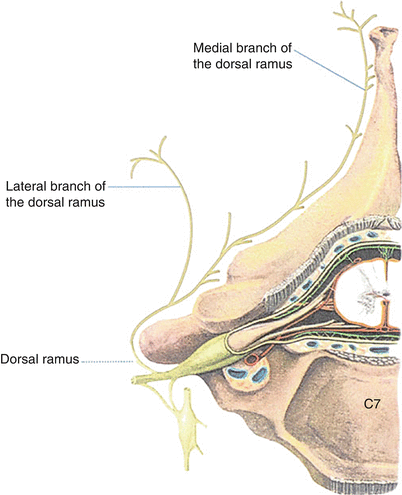
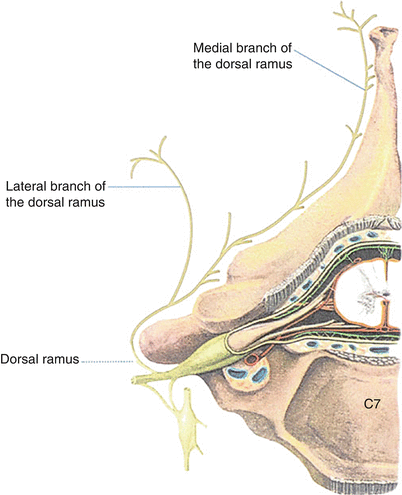
Fig. 12.8
The course of the dorsal ramus proper and its lateral (LBDR) and medial branches (MBDR) represented semi-schematically at the level of C7 (Modified from Sinelnikov [31]. Modified and prepared for publication by Tracey James. All rights reserved. No part of this picture may be reproduced or transmitted in any form or by any means without written permission from Felix Linetsky M.D.)
Thus, MBs do not exclusively supply innervation to the cervical, thoracic, and lumbar ZJ but also to the structures that have enthesis at the spinous processes. This explains the similarity of clinical presentations and the significant overlap of the known pain patterns (Figs. 12.1, 12.2, 12.3, 12.4, 12.5, 12.6, 12.7, and 12.8) [2–4, 10–13, 28, 56, 62].
Current prevailing trends in diagnostic efforts address discogenic, facetogenic, and neurocompressive components of spinal pain. The therapy is directed toward neuromodulation or neuroablation with radiofrequency generators or corticosteroid injections [25]. Example, cervical ZJ is responsible for 54 % of chronic neck pain after whiplash injury; the prevalence may be as high as 65 % [58]. In patients with headaches after whiplash, more than 50 % of the headaches stem from the C2 to C3 z-joint [25, 58]. Intra-articular corticosteroid injections are ineffective in relieving chronic cervical z-joint pain [59]. These statistical data strongly suggest the presence of nociceptors other than ZJ and IVD [22, 25, 58, 59].
Spondyloarthropathies with enthesopathies and muscular, ligamentous, and tendinous pain are rarely, if ever, included in the differential diagnosis or therapeutic plan. The unspoken reasons for this are economical. Major insurance carriers identify the MBDR block as a ZJ block. Any other injections are considered trigger point or ligament injections, and only two ligament or tendon injections or a maximum of three trigger point injections with corticosteroids are reimbursed during the same office visit at a very low rate. The fact that there may be several nociceptors in the same area in the same patient at the same time is disregarded.
The other reason can be explained by the spinal uncertainty principle. In a simple example of two motion segments, the disc, facets, and musculotendinous compartments are each considered as one putative nociceptive unit, the total number of clinically indistinguishable combinations rises to 63 possibilities. It is practically impossible to address such a magnitude of possibilities under fluoroscopic guidance.
In the majority of cases, RIT can be done without radiologic guidance, taking innervation into account. Therefore, it can afford evaluation of many putative nociceptors from the variety of pain presentations and offers a practical advantage that can be accomplished during the same procedure (Fig. 12.9). The syndromes and conditions treated with RIT are listed in Table 12.1 [11, 17–22, 39, 52–55, 57, 60–84].
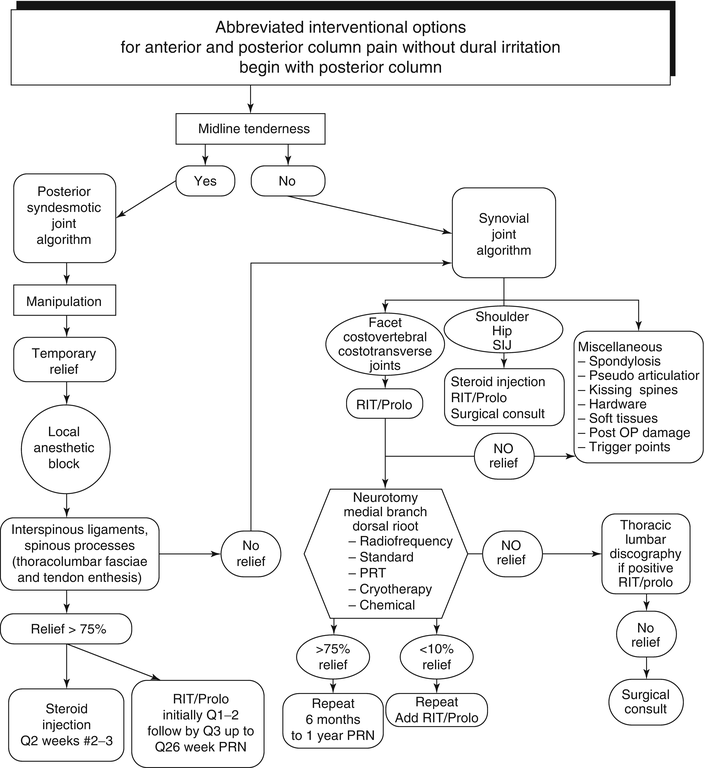
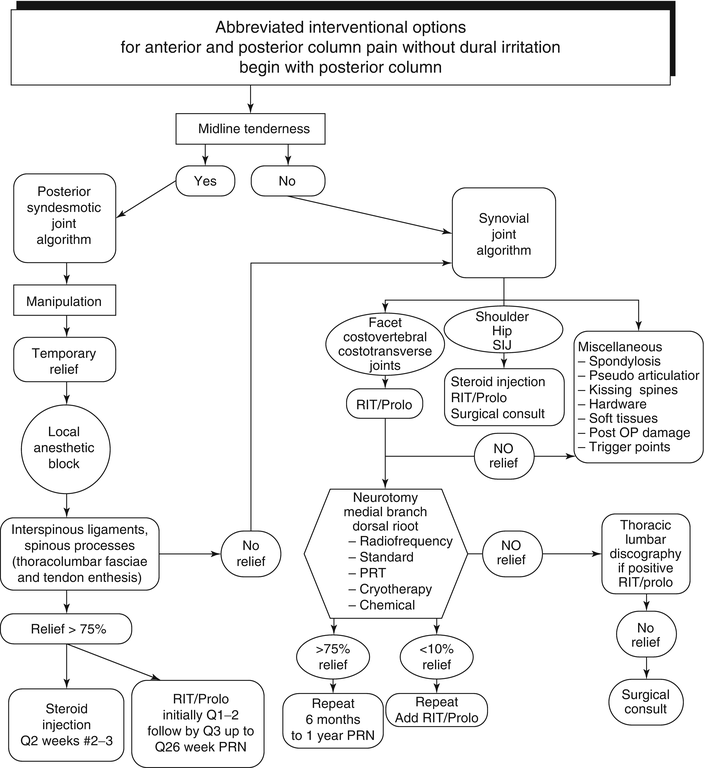
Fig. 12.9
Self-explanatory, modified, abbreviated excerpt from interventional options for spinal and paravertebral pain without dural irritation including large synovial joints (From Linetsky et al. [57])
Table 12.1
The syndromes and conditions treated with RIT
Barre–Lieou syndrome | Acromioclavicular sprain/arthrosis |
Cervicocranial syndrome (cervicogenic headaches) | Scapulothoracic crepitus |
Temporomandibular pain and dysfunction syndrome | Rotator cuff syndrome: supraspinatus, infraspinatus, subscapularis tendinosis, or impingement |
Whiplash injury syndrome, spasmodic torticollis | Proximal and distal biceps tendinosis |
Cervical and cervicothoracic spinal pain of “unknown” origin | Tennis and golfer’s elbow |
Cervicobrachial syndrome (shoulder/neck pain) | Baastrup’s disease – kissing spine |
Snapping scapulae syndrome or scapulothoracic crepitus | Recurrent shoulder dislocations |
Hyperextension/hyperflexion injury whiplash syndromes | Myofascial pain syndrome |
Cervical, thoracic, and lumbar facet syndromes | Ehlers–Danlos syndrome |
Cervical, thoracic, and lumbar sprain/strain | Marie–Strumpell disease |
Cervical, thoracic, and lumbar disc syndrome | Internal disc derangement |
Slipping rib syndrome | Failed back surgery syndrome |
Costotransverse and costovertebral joint arthrosis pain and subluxations | Low back pain syndrome |
Sternoclavicular arthrosis and repetitive sprain and subluxations | Iliac crest syndrome |
Acromioclavicular arthrosis and instability | Friction rib syndrome |
Repetitive thoracic segmental dysfunction | Sacroiliac joint sprain/strain and instability |
Costosternal arthrosis/arthritis | Groin pull/sprain/strain |
Tietze’s syndrome/costochondritis/chondrosis | Coccydynia syndrome |
Interchondral arthrosis | Groin sprains |
Xiphoidalgia syndrome | Snapping hip syndrome |
Gluteus minimus and medius tendinosis | |
Trochanteric tendinosis | |
Patellar tendinosis | |
Osgood Schlatter disease | |
Achilles tendinosis |
Clinical Presentation and Evaluation
The list of syndromes and conditions gives the reader the idea that there is a wide variety of presenting complaints including headaches, neck pain, low back pain, pain between the shoulders, mid-scapular pain, pain mimicking pleurisy or various radiculopathies, thoracolumbar area pain, occipital and suboccipital pain, low back and hip pain, neck and shoulder pain, sharp pain with difficulty breathing, tail bone pain with difficulty seating, and any combination of these symptoms. The intensity, duration, and quality of pain are variable, and the onset may be sudden or gradual. The evaluation may reveal postural abnormalities, functional asymmetries, and combinations of kyphoscoliosis, flattening of cervical and lumbar lordosis, and arm or leg length discrepancies. A wide range of increased or restricted passive and active range of motions as well as frank deformities of axial or peripheral joints may be present.
Contractions against resistance usually denote a tendon-related pain, whereas passive attempts to bring a joint to the anatomic range indicate a ligament-related pain. The most reliable, objective clinical finding is tenderness which may be present at the fibro-osseous junction (enthesis) or at the midsubstance of a muscle, ligament, or tendon. Such areas of tenderness are identified and marked and become the subject of ultrasound investigation and eventually needle probing “needling” and local anesthetic block. The needle placement at the areas of maximum tenderness usually reproduces the pain that becomes temporarily worse during infiltration of local anesthetic and usually subsides within 10–15 s after infiltration. Such diagnostic blocks may be performed with or without fluoroscopic or ultrasound guidance. Abolishment or persistence of tenderness and or local or referred pain concludes the clinical examination and becomes the basis for clinical diagnosis (Figs. 12.9 and 12.10) [11, 22, 57, 63–65].
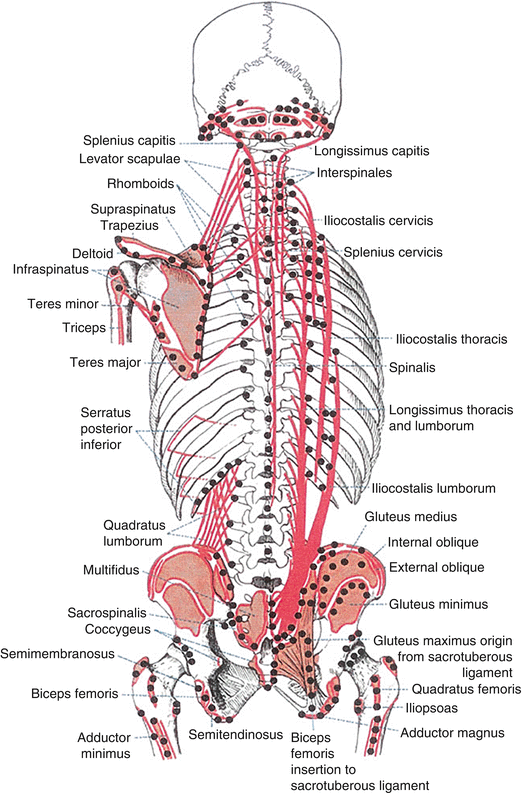
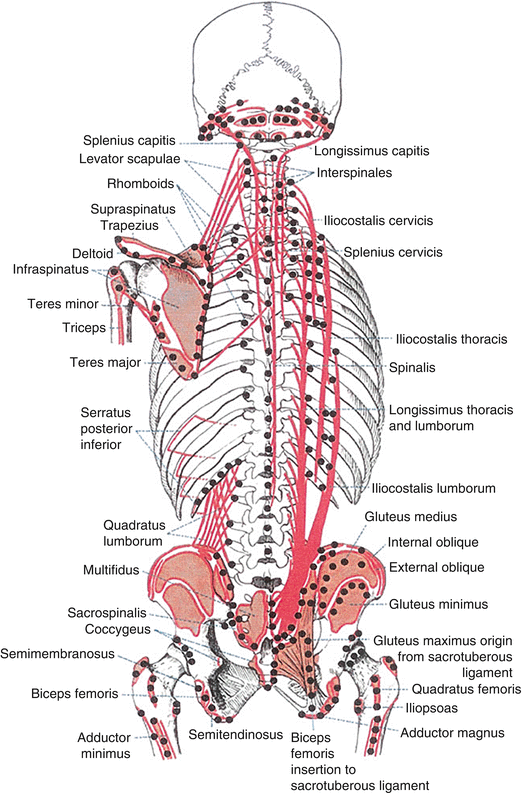
Fig. 12.10
Schematic drawing demonstrating sites of tendon origins and insertions (enthesis) of the paravertebral musculature in the cervical, thoracic, lumbar, and pelvic regions with parts of the upper and lower extremities. Clinically significant enthesopathies with small fiber neuropathies and neuralgias are common at the locations identified by dots. Dots also represent most common locations of needle insertion and RIT injections (Note: Not all of the locations are treated in each patient) (Modified from Sinelnikov [31]. Modified and prepared for publication by Tracey James. All rights reserved. No part of this picture may be reproduced or transmitted in any form or by any means without written permission from Felix Linetsky M.D.)
Radiologic Evaluation Relevant to RIT
Plain Radiographs
Plain radiographs are of limited diagnostic value in painful pathology of the connective tissue, but may indirectly suggest the presence of such pathology by detecting structural or positional osseous abnormalities, like anterior or posterior listhesis on flexion/extension lateral views and degenerative changes in general with deformities of the osseous and articular components such as osteophyte formations in various parts of the skeleton, ectopic calcifications, and improperly healed fractures [66].
Magnetic Resonance Imaging (MRI)
MRI may detect the pathology of intervertebral disc, ligamentous injury, interspinous bursitis, enthesopathy, ZJ disease, SIJ pathology, neural foramina pathology, bone contusion, infection, fracture, or neoplasia. Magnetic resonance imaging may exclude or confirm spinal cord disease and pathology related to extramedullary, intradural, and epidural spaces. MRI detects cartilage abnormality, degenerative tendon and ligament pathology, tendinosis, joint effusions, bursitis, soft tissue edema, hematoma, ligament tendon and muscle rupture, and vascular abnormalities [66, 67].
Computed Tomography Scans (CT)
CT scan may detect small avulsion fractures of facets, laminar fracture, fracture of vertebral bodies and pedicles, and neoplastic or degenerative changes in the axial or appendicular skeleton [66].
Bone Scan
Bone scans are useful in assessing entire skeleton to evaluate for metabolically active disease processes [66].
Diagnostic Ultrasound
Gray scale (GS) ultrasound can detect in real time joint effusions, bursitis, cystic formations, synovial hypertrophy, cartilage abnormality, muscle atrophy, attenuation or partial disruptions of ligaments, tendons or muscles, ectopic calcifications, tendon enlargement, inhomogeneity in tendinosis, and nerve hypertrophy like in carpal tunnel syndrome. Nerve and tendon subluxations or impingements are evaluated with dynamic ultrasound. GS ultrasound provides real-time needle guidance during various diagnostic or therapeutic injections including aspirations, nerve blocks, and percutaneous needle tenotomy. Ultrasound is becoming a more useful tool in the assessment of myofascial and osseous pain sources because it allows a dynamic pattern recognition as well as direct evaluation and patterning in superficial collagenous structures. Ultrasound is now a preferred method to evaluate rotator cuff pathology in the office setting and is gaining popularity in knee joint evaluation prior to arthroscopy.
The color Doppler (CD) ultrasound can detect neovascularities to be injected, when present, in tendinosis or synovitis and delineate positions of large vessels and nerves to be avoided during injections [52–55, 68, 69]. Unless the practitioner is very experienced in MSK, ultrasound correlations with plain radiographs, MRI, CT scans, and palpation are highly advisable. There are a multitude of weekend courses in musculoskeletal ultrasound; the industry is promoting the methodology, but the high quality hands on supervised training is not yet available at the academic institutions for the practicing physicians. Gaining a supervised high-quality experience takes time.
Solutions for Injections
Local anesthetics are an important component of the solutions used for RIT and were described under the heading of Local Anesthetics in the Diagnosis of Musculoskeletal Pain. When contemporary local anesthetics are combined with hyperosmolar injectates, they provide long-lasting diagnostic/therapeutic blocks, and the reasons for this scientifically proven effect will be described below.
Five types of injectates are used for RIT, and they are:
1.
Osmotic shock agents such as hypertonic dextrose, glycerin, or distilled water
2.
Chemical irritants such as phenol
3.
Chemotactic sclerosing agents such as sodium morrhuate, Sotradecol, or polidocanol
4.
Particulates such as pumice suspension
5.
Biologic agents such as whole blood, platelet-rich plasma (PRP), autologous conditioned serum (ACS), platelet-poor plasma (PPP), adipose-derived and bone marrow aspirate concentrates with their mesenchymal and hematopoietic biocellular components, and isolated and cultured mesenchymal stem cells
The injectates in groups 1–4 have been used as a single agent in various concentrations or in various combinations with other chemical agents, and their concentrations are mixed with local anesthetics, by the virtue of being injected into connective tissue, all of them become irritants [57, 60, 61, 63–65, 71–74]. Injectates in group 5 are also used as a single injectate agent in various concentrations or in various combinations of the agents and their concentrations.
Experimental studies demonstrated that any solution with osmolality greater than a 1,000 mOsm/l is neurolytic, causing separation of the myelin lamellae in myelinated nerve fibers and total destruction in unmyelinated fibers, after soaking for 1 h in solutions with osmolality greater than 1,000 mOsm/l or a distilled water. Hypoosmolar solutions produce a reversible conduction block of rabbit vagus nerve and potentiate the local anesthetics. C fibers showed evidence of axonal damage characterized by accumulation of macrophages and proliferation of Schwann cells. Osmotic fragility of axons is similar to that of erythrocytes after exposure to 0.4 and 0.5 dilutions of normal saline. When administered intrathecally, local anesthetics are more effective in hypobaric solution than in hyperbaric solution [85–88]. In humans, intrathecal hypertonic saline produced good results in chronic intractable pain and is currently used in epidurolysis of adhesions [17, 89–91]. Hypertonic/hyperosmolar dextrose has been successfully used for treatment of enthesopathies with small fiber neuropathies, spondyloarthropathies, and internal disc derangements [1, 11, 17, 19–22, 57, 73, 74].
Pharmacologic properties of phenol, glycerin, and hypertonic dextrose are both neurolytic and inflammatory. Various concentrations of water- and glycerin-based phenol solutions have been used to treat pain. The literature suggests that perineural phenol glycerin combinations produce a better regenerative/reparative response; these experimental findings support the use of phenol glycerin or phenol glycerin dextrose solutions in treatment of axial and peripheral enthesopathies with small fiber neuropathies and neuralgias [92–102].
Neurolytic intra-articular injections of a 10 % aqueous phenol, diluted to 5 % with omnipaque or omniscan contrast and local anesthetic, are used in the Pain Management Department of Mayo Clinic to facilitate nursing care in severely debilitated patients [103].
Diluted 5 % phenol in 50 % glycerin solution is used for the treatment of spinal enthesopathies and injections at donor harvest sites of the iliac crest for neurolytic and regenerative/reparative responses. Prior to injection, 1 ml of this solution is mixed with 4 ml of local anesthetic 1,086 mOsm/l [63, 64]. The most common solutions contain lidocaine/dextrose mixtures in various concentrations. Lidocaine is available in 0.5–2 %; dextrose is available in a 50 % concentration.
To achieve a 10 % dextrose concentration, dilution is made with lidocaine in 4:1 proportions (i.e., 4 ml of 1 % lidocaine is mixed with 1 ml of 50 % dextrose) and will produce a 0.8 % lidocaine with osmolality of 555 mOsm/l (hyperosmolar block).
To achieve a 12.5 % dextrose concentration, dilution is made with lidocaine in 3:1 proportions (i.e., 3 ml of 1 % lidocaine mixed with 1 ml of 50 % dextrose) and will produce a 0.75 % lidocaine with osmolality of 694 mOsm/l (hyperosmolar block).
To achieve a 20 % dextrose concentration, dilution is made with lidocaine in 3:2 proportion (i.e., 3 ml of 1 % lidocaine mixed with 2 ml of 50 % dextrose) and will produce a 0.6 % lidocaine with osmolality of 1,110 mOsm/l (hyperosmolar neurolytic block). In two studies, this solution produced a 50 % reduction in low back pain lasting for 2 years.
A 1:1 dilution makes a 25 % dextrose concentration with 0.5 lidocaine solution with osmolality of 1,388 mOsm/l (hyperosmolar neurolytic block). In two studies, this solution was used for intradiscal injections.
Dextrose/phenol/glycerin (DPG) solution is referred to as DPG or P2G and contains dextrose and glycerin in equal 25 % amounts, 2.5 % phenol and water. Prior to injection, DPG is diluted in concentrations of 1:2 = 1,368 mOsm/l, 1:1 = 2,052 mOsm/l, or 2:3 = 1,641 mOsm/l with a local anesthetic.
When dextrose-containing solutions are not controlling pain and dysfunction, progression to stronger solutions such as sodium morrhuate, Sotradecol, or polidocanol has been used in various dilutions up to a full strength.
Five percent sodium morrhuate is a mixture of sodium salts of saturated and unsaturated fatty acids of cod liver oil and 2 % benzyl alcohol (chemically very similar to phenol), which acts as both a local anesthetic and a preservative. This is very well tolerated in selective patients with rheumatoid arthritis or ankylosing spondyloarthropathies, personal observation of the senior author.
Sotradecol® (sodium tetradecyl sulfate injection) is a sterile nonpyrogenic solution for intravenous use as a sclerosing agent. Three percent (30 mg/ml) with 2 % benzyl alcohol: Each mL contains sodium tetradecyl sulfate 30 mg and benzyl alcohol 20 mg. It can be used interchangeably with sodium morrhuate; clinical results are similar, but there is a lesser possibility of allergic reactions.
Polidocanol is a nonionic detergent, containing a polar hydrophilic (dodecyl alcohol) and an apolar hydrophobic (polyethylene oxide) chain as active ingredients. On March 31, 2010, the US Food and Drug Administration (FDA) approved polidocanol injection for the treatment of small varicose veins. Polidocanol is a local anesthetic and antipruritic component of ointments and bath additives. The substance is also used as a sclerosant, an irritant injected to treat varicose veins. Professor Alfredson has extensively used 1 % polidocanol in 1–2 ml increments for the treatment of tendinosis [52–55].
Pumice suspension: Pumice is a substance of volcanic origin consisting chiefly of complex silicates of aluminum, potassium, and sodium. Pumice is insoluble in water and is not attacked by acids or alkali solutions. It is used in this preparation as a material irritant to stimulate the fibrosing process. Extra fine grade is defined as one that passes a 325 mesh sieve at 84 % or more, and only a trace is retained by a 200 mesh sieve:
Pumice (extra fine grade) – 1.0 g.
Glycerin – 5.0 ml.
Polysorbate 80–0.09 ml (2 standard drops).
Preservatives q.s.
Lidocaine 1–2 % q.s. ad 100 cc.
Place in a multidose bottle, sterilize, and shake well before use.
Two to three milliliter of this suspension is drawn in a 10-ml syringe mixed with dextrose formula of a choice or alone. Drawing in to the syringe should be done through the same gage needle that will be used for injection. Suspension was developed by Dr. Gedney for injections of sacroiliac ligaments to stabilize SI and lumbosacral joints [19–22].
Biocellular autografts include whole blood, platelet-rich plasma (PRP), autologous conditioned serum (ACS), platelet-poor plasma (PPP), and adipose- and bone marrow-derived aspirate concentrates with mesenchymal and hematopoietic components [104–109]. Widely popularized and accepted in recent years, these autografts are composed of three ingredients used separately or together:
1.
PRP or ACS provides platelet concentrates with cytokines and growth factors.
2.
Autologous fat cells provide a living collagen bioscaffold with its intrinsic stromal vascular tissue transferred in the form of a graft or a lyophilized collagen in the form of an injectate which may be utilized as a cellular bioscaffold matrix.
3.
Lipoaspirates or adipose tissue plus/minus bone marrow aspirate concentrate provides stromal vascular fraction with supporting mesenchymal stem cells.
PRP is a platelet concentrate of four- to eight-fold above baseline levels that contain signal proteins, platelet-derived growth factors, chemokines, and cytokines that control inflammatory cascade. Autologous conditioned serum (ACS or ACP) contains platelet concentrations of two to three-fold baseline levels, and whole blood contains platelet levels at baseline. It remains a point of debate in the literature which autograft provides a superior collagen growth. It may depend on the structure to be regenerated which level of chemokine and cytokine concentration or MSC concentration or pure scaffold regeneration proves most helpful.

Full access? Get Clinical Tree
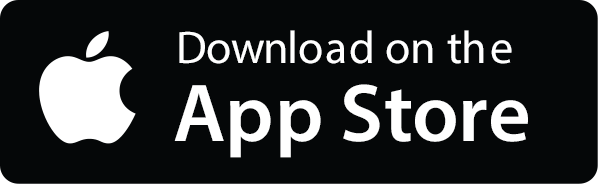
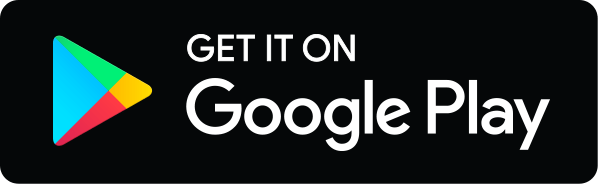