Chapter 36
Trauma Anesthesia
Etiology of Traumatic Injury
Traumatic injury is a unique condition. Unlike other diseases that have a biologic basis, trauma is a result of an external force that ultimately disrupts normal structure and function of the body. In most situations, the initial cause of injury is not a result of genetics or environmental exposure, but circumstance and misfortune. Traumatic injury is a disease of human behavior. Although improvements to automobile safety and development of public policy have successfully reduced the annual number of traumatic injuries caused by motor vehicle collisions (MVCs), falls, and firearms by nearly a third, traumatic injury still remains the leading cause of death for Americans under the age of 40.1 This translates to 180,000 deaths in the United States alone, or more simply put, one death every 3 minutes.2 The mortality of injury is striking; however, it represents only a small fraction of those affected by trauma.
There is no absolute number that can precisely quantify the toll and cost of trauma. Although figures vary by author, it has been estimated that the cost for medical care and lost productivity in the United States was more than $400 billion in 2005—of that amount, nearly $100 billion resulted from MVC alone.1,3 These figures, while staggering, account only for expenses and do not account for the burden that injury places on utilization of scarce medical resources.2 Mortality is only the tip of the iceberg. Trauma accounts for nearly 30 million emergency care treatments per year in the United States, a tenth of which will require hospital admission and possibly surgical intervention.2,3,4
Some progress in improving these statistics has clearly been made in the United States, but prevention and treatment of trauma outside of the United States has lagged. Globally, injury is increasing in developing nations.5 The World Health Organization (WHO) demonstrated in the year 2000 that 1.6 million people died as a result of trauma.6 Although half of these deaths were a result of suicide, the remainder were due to homicide or combat-related violence.6 The WHO estimates that injury will be the leading cause of death worldwide by 2020.1 If the prediction is true, the morbidity and mortality of injury—a manmade illness—will far surpass that of infectious diseases. This change will represent a true paradigm shift in global health.
Coordinated Management of Care
The implementation of the modern trauma system has resulted in significant improvement in patient outcomes. Pioneering examples of this paradigm shift can be seen throughout many cities in the United States and around the world; the impact of this shift on patient morbidity and mortality was formally recognized in 1998. The Skamania Symposium (Skamania Lodge, Oregon; Academic Symposium to Evaluate Evidence Regarding the Efficacy of Trauma Systems) was the first academic group to evaluate the efficacy of a trauma system.7 This group systematically evaluated published data to determine the impact of trauma systems. Their findings were that care in a trauma center (versus a nontrauma center) was associated with fewer unnecessary deaths and less disability.7,8 These findings have been supported by several other groups in the United States. Risk of death is considerably lower among patients who require early operative intervention if they are treated at a designated Level I trauma center. These outcomes are not a result of more rapid assessment and intervention alone, and emphasize the complex factors that contribute to the survival benefit of trauma center care. Studies in the United States show that mature, statewide trauma systems dramatically reduce unnecessary deaths from greater than 30% to less than 5%, compared with nontrauma system care.9–12
Organization of trauma systems varies across the United States. Even though systems are not the same and may vary widely, the American College of Surgeons (ACS) Committee on Trauma has developed standards to which all trauma systems must adhere to become accredited.13 Through these standards, a level (Level I, Level II, etc.) can be assigned to a particular center that designates the resources the center can provide to care for an injured patient. Figure 36-1 describes the characteristics of the modern trauma system, its resources, and the levels of trauma care. What we can take away from the conclusions of the Skamania Symposium and guidance of the ACS Committee on Trauma is that positive outcomes in patient care are directly related to experience and development of a trauma system. Trauma can no longer be considered an offshoot skill where the paradigm involves massive fluid resuscitation, but a specific clinical expertise in which management and therapy directly impacts patient outcome. In short, experience and proficiency matter.
Early Evaluation of the Trauma Patient and Common Injury Patterns
Immediate Admission of the Trauma Patient
Prehospital
In most cases, urgent airway management is not required in the prehospital setting. Occult hemorrhage and its ensuing pathology, on the other hand, is the greatest cause of early death from trauma.14 Blood leaving the circulatory system can spill into cavities throughout the body (e.g., thorax, abdomen, retroperitoneum [the pelvis], or fascial planes of long bones) and/or bleed into the environment (i.e., the street). Classically, hemorrhage was met with intravenous (IV) access and volume resuscitation in the prehospital setting. This practice, however, is going through a renaissance of sorts in an effort to determine what is best practice and management.
Primary Survey
Upon admission to the hospital or trauma center, ATLS guidelines provide a logical and sequential treatment strategy for rapidly assessing the patient. This process is commonly referred to as the ABCDE’s of trauma care15:
The goal of the primary survey is to identify and rapidly manage life-threatening conditions or injuries. This sequence of events will be abbreviated and discussed individually from the anesthesia perspective shortly. Generally, however, the primary assessment involves a rapid assessment using physical examination techniques and American Society of Anesthesiologists (ASA) standard monitors. In addition, ultrasound and radiography are used to examine body cavities with the initial goal of determining the extent of injury. During this time, initial blood samples and IV access are obtained. All aspects of the primary survey are done simultaneously, thereby coordinating the efforts of surgical and anesthesia teams to prepare for potential surgical intervention. In the event the injuries are beyond the scope of the initial receiving facility, the primary survey provides the emergency team with enough information to stabilize and prepare the patient for transfer to a higher-level facility.
Blunt Versus Penetrating Trauma
Motor Vehicle Collision Trauma
Blunt trauma is most closely associated with motor vehicle collisions and falls. Blunt trauma tends to produce effects bodywide. The five types of motor vehicle collisions are classified as head-on, rear impact, side impact, rotational impact, and rollover. Injuries can be categorized as those above and those below the waist. The upper portion of the body may collide with the dashboard, steering wheel, or windshield, resulting in injuries of the head, neck, chest, abdomen, and upper extremities. Below the waist, injuries to the knees and femurs occur because of direct contact with the vehicle and lower dashboard. Acetabular fractures are typically a result of tensing the leg when bracing for impact. Blunt trauma rarely occurs in isolated body systems. As such, all blunt trauma victims, including those ultimately without high-risk mechanisms of cervical spine injury, should be suspected of and treated as if they have an unstable cervical spine until proven otherwise.15
Thoracic Trauma
Blunt chest trauma is the third leading result of injury—closely following traumatic brain injury and extremity trauma. Patients with blunt thoracic trauma present a unique series of concerns. These patients represent some of the most severely injured—with multisystem involvement—accounting for nearly 25% to 50% of all trauma deaths.16,17 In developed nations, thoracic trauma is most often associated with motor vehicle collisions.
Pneumothoraces are present in as many as 40% of all blunt thoracic injuries.16 The size and location of the pneumothorax may vary throughout the lung field. Although the etiology and management of pneumothoraces is somewhat commonplace to providers, the presence, or in many cases the assumed absence, of a pneumothorax should not be minimized. It is estimated that as many as 50% of pneumothoraces are not detected on initial radiography.17 This occurrence presents several clinical intraoperative issues and may alter an anesthetic plan. Nitrous oxide should be avoided in patients with suspected thoracic trauma.
Tension Pneumothorax
Massive pneumothorax can result in reductions in cardiac output and ultimately cardiovascular collapse. Under emergent situations, a large-bore intravenous catheter (needle chest decompression) can be inserted into the second intercostal space just above the third rib, along the midclavicular line. Release of pressure should restore cardiac function. Initially, the catheter can be temporarily attached to an intravenous line extension tube and placed under water seal by putting it in a bottle of sterile water positioned beneath the level of the patient until proper chest tube thoracostomy can be performed. See Chapter 26 for a complete discussion of the diagnosis and management of a pneumothorax.
Tracheal Injuries
Airway injuries represent a devastating and potentially lethal event after blunt thoracic trauma. The relative infrequency of airway injury upon admission to a trauma center (0.2%-8.0%) is likely due to high victim mortality at the scene of injury.16,17 Partial disruption of the trachea or major bronchi often is mangaged through securing of the airway (by intubation or tracheostomy) and surgical correction. Total disruption of the trachea is often fatal unless rapid surgical retrieval of the distal disrupted airway segment is accomplished to allow lifesaving mechanical ventilation. The majority of thoracic airway injuries are found below the carina and are often visible only during bronchoscopy or computed tomography (CT) examination.
Penetrating Trauma
Penetrating injuries can range from a simple pinprick to high-velocity projectile injury. Damage depends on three interactive factors18:
1. The type of wounding instrument (e.g., knife; missile, such as a bullet; or fragment, such as a piece of shrapnel)
2. The velocity of the missile at time of impact
3. The characteristics of tissue through which it passes (e.g., bone, muscle, fat, blood vessels, nervous tissues, and organs)
Damage Control Surgery
Surgical management for the severely traumatized patient is often a multistep process. In many cases, patients present to the trauma center with surgical emergencies. Early repair is often simply a life-saving measure and is not intended to be a definitive repair but rather a stabilizing measure, intended to reduce operating room (OR) time and morbidity.19,20 After stabilization, patients will be transported for further evaluation (secondary survey) or additional resuscitation measures in the intensive care unit (ICU). Often patients will be returned to the OR several times because the surgical course involves several phases. This staged approach to surgical management is commonly known as damage control surgery (DCS).
Damage control surgery is a concept that developed in the early twentieth century. Its use fell in and out of favor until the 1970s and 1980s. Its utility in modern trauma care was rediscovered as advances in surgical technique, critical care medicine, and technology converged. DCS correlates with current concepts in trauma care that include damage control resuscitation with rapid surgical correction of bleeding and the prevention of the lethal triad of acidosis, hypothermia, and coagulopathy. It also involves limitation of crystalloid administration and application of high ratios of plasma and platelets to packed red blood cells.21,22 DCS is used in various surgical disciplines, from packing the abdomen after abdominal trauma to using external fixators to set complex orthopedic injuries.23
Abdominal Trauma
Blunt abdominal trauma is a leading cause of morbidity and mortality among all age groups. Although diagnosis and treatment of penetrating trauma is easily determined, occult bleeding in blunt abdominal injury is often misdiagnosed.24,25 Abdominal sonography, Focused Assessment with Sonography for Trauma (F.A.S.T.), computed tomography (CT) scan, magnetic resonance imaging (MRI), or angiography may help in the diagnosis of specific injuries and various treatment modalities. Extremely unstable patients, however, will require immediate surgery. It is essential that large-bore intravenous access be in place, above the diaphragm, prior to opening the abdomen in the event of massive hemorrhage as a result of liver or other organ injury.
The ABCD’s of Trauma Anesthesia
Airway
Endotracheal intubation is a routine procedure of anesthesia practice. Despite its regular use during general anesthetics, intubation poses significant risk and may be extremely challenging when caring for the acutely injured patient. Difficult tracheal intubation is the third most common respiratory-related event leading to death and brain damage as reported in the ASA Closed Claims analysis.26 Although certainly not all trauma patients will have a difficult airway, the anesthetist likely will not have the opportunity or time for a full airway examination because of several variables such as facial injuries and/or hypoventilation and apnea. As such, the provider must anticipate the worst-case scenario.
Emergent intubation in the trauma patient follows the general pathway of the ASA difficult airway algorithm.27 Three assumptions should be made when approaching this type of patient. First, there is no turning back. Intubation is not routinely elective for these patients. Therefore, once induction begins the patient must end up with a controlled airway. In addition to requiring a secured airway, trauma patients are assumed to have delayed gastric emptying and a full stomach. As such, they are at increased risk for aspiration. Finally, any patient with blunt trauma or with penetrating injuries to the neck and face must be considered for cervical spine instability.
Rapid sequence intubation (RSI) is the standard method for traumatic airway management. This practice involves several steps often not used during standard induction. One of the greatest differences between routine induction and RSI is the use of a muscle relaxant before knowing whether the patient can be mask ventilated. Although daunting, and a deviation from the norm, muscle relaxation is associated with the highest overall rate of successful airway management and provides the greatest possibility for rapidly securing the airway.28
Steps to RSI
Direct laryngoscopy during manual in-line stabilization of the cervical spine is a safe and effective procedure in patients with potentially unstable necks.29,30 Cricoid pressure is applied and intended to prevent both gastric insufflation during bag-valve-mask ventilation and passive reflux of gastric contents. Although caution is appropriate, both cervical spine injury and aspiration during intubation are moderately low-risk events when compared with the possible risks and injury from hypoxia. Therefore, it must be remembered that airway management is the priority. In-line stabilization and cricoid pressure should be relaxed if they are interfering with successful intubation.
Common Indications for Airway Management
Preoxygenation
Adequate preoxygenation is likely the best asset for the anesthetist managing a trauma patient. Preoxygenation provides the greatest amount of time before occurrence of hypoxemia. Preoxygenation is accomplished using 100% high-flow (10-15 L) oxygenation via a nonrebreather facemask or bag-valve facemask. Although there is some debate in the literature, four to eight tidal volume breaths appear to provide superior preoxygenation when compared with 3 minutes of tidal breathing.31
Cricoid Pressure
Cricoid pressure was first described by Sellick in 1961.32 The goal of this maneuver is to reduce the risk of pulmonary aspiration of gastric contents by compressing the esophagus with the continuous ring of the cricoid cartilage.33 Cricoid pressure is maintained throughout the RSI and is not released until endotracheal tube (ETT) placement has been confirmed. Determining the appropriate pressure to apply to the cricoid ring has been the subject of debate. Vanner and Pryle34 have determined that 30 Newtons (approximately 10 lbs of pressure) adequately occludes the esophagus.
Induction Agents
Anesthetic induction for RSI can be achieved by a variety of agents. At this time no literature is available to support the superiority of one agent over another. All induction agents will cause dose-dependent decreases in blood pressure in the hypovolemic, hemorrhaging patient. Dose-dependent hemodynamic instability can likely be attenuated by reductions in the induction dose. Although no formula can precisely predict the dose for a hemodynamically unstable patient, some authors suggest a dose that is one tenth to one half of the normal induction dose of propofol.35 Ketamine can always be considered as an alternative trauma induction drug.36,37 Etomidate use is discouraged due to the suppression of adrenal function. Recall during induction, an undesirable consequence, is a secondary concern when urgently managing an unstable trauma patient.
Succinylcholine (1.5 mg/kg) provides favorable and rapid muscle relaxation to facilitate intubation. It is generally the preferred agent for RSI for any patient who has no specific contraindication to its use. Succinylcholine administration may cause lethal hyperkalemia in patients with neurologic deficits from spinal cord injury, but not until 24 to 48 hours after injury.38
Rocuronium (1.2 mg/kg) and vecuronium (0.2 mg/kg) also can be used for RSI. The onset time for high-dose rocuronium (1.2 mg/kg) is similar to that for succinylcholine with only a slight delay in achieving complete relaxation.39 Although these nondepolarizing agents may produce adequate intubating conditions, their use may influence what care can be provided. Prolonged paralysis will require sedation and will make any subsequent neurologic assessment more difficult.
Apneic Ventilation
To avoid potential hypoxemia or in the event of an already hypoxemic patient, clinical modifications have been made to RSI that include bag-valve-mask ventilation through cricoid pressure—often termed “modified RSI.” This method is actually the technique described by Sellick in 1961.32,40 To date, there does not appear to be any increase in aspiration by bag-valve-mask ventilation through cricoid pressure.
Direct Laryngoscopy
If intubation is again unsuccessful (third attempt), the next step should be an airway adjunct to support oxygenation. These adjuncts range from the Combitube to the laryngeal mask airway (LMA). In the situation of “cannot intubate and inadequate facemask ventilation,” LMA insertion should be the immediate next step.41
Airway Management of Cervical Spine Injuries
Cervical spine injury remains a significant concern when facing airway management of the trauma patient. The incidence of cervical spine injury after trauma is relatively rare at 2% to 3% of all trauma and approximately 6% to 10% for patients with traumatic brain injury.42 Cervical spine injury should be assumed until proven otherwise. Immobilization of the neck is essential. To that end, in-line stabilization is essential because it allows for removal of the front of the cervical collar, allowing more area for jaw and mouth movement, while limiting the risk for further injury.
The management and intubation of patients with suspected cervical spine injuries remains clinically controversial. Despite common belief, there is no evidence to suggest that fiberoptic intubation provides safer patient outcomes when compared with direct laryngoscopy with in-line stabilization.43–45 As such, management of a cervical spine injury may be done so with the concepts of “emergent” which involves in line stabilization and RSI versus “controlled” which involves an awake fiberoptic technique. Figure 36-2 describes a logical management strategy for these two situations.
Breathing (and Ventilation)
Pulmonary contusions represent the most common lung injury. It is reported that as many as 70% of patients with blunt thoracic trauma present with some degree of pulmonary contusion.17 Pulmonary contusions are injuries to the alveoli without gross disruption of pulmonary architecture. This injury is essentially a bruise to the lung tissue resulting in protein-rich fluid leaving ruptured pulmonary capillaries and settling into the alveolar membrane and interstitial space. Due to the widening of the pulmonary membrane, pulmonary contusions result in varying degrees of reduced gas diffusion that may be clinically relevant. Pulmonary contusions can develop or “blossom” over a range of time and ultimately may develop into acute respiratory distress syndrome (ARDS).
ARDS is a common problem in trauma care. It may be a result of injury or the resuscitation of the patient. It has been estimated that between 10% and 40% of patients with traumatic injury will develop ARDS over their hospital course. Pathologically, ARDS is a result of protein-rich fluid leaving the pulmonary capillaries. As the disease progresses, the pulmonary capillary leakage is compounded by embolic events, which further increase intracapillary pressure and intensify interstitial leakage. ARDS culminates in hypoxia and decreased pulmonary compliance. Ventilating these patients is a challenge. Clinicians are often faced with the inability to appropriately oxygenate the patient without using high pressures to ventilate.46 High pressures and decreased compliance sets the patient up for barotrauma and worsening pulmonary disease.
A variety of pulmonary techniques have been described for managing these patients. They range from high-frequency oscillation ventilation to cardiopulmonary bypass. At this time no one technique appears to be superior. It is widely accepted, however, that these patients should be ventilated using low tidal volumes to reduce peak pressures, and the plateau pressures should be maintained at less than 32 cm H2O.47,48 Although attempting to correct an Sao2 that is less than normal (less than 95%) by increasing the Fio2 may seem to be a desirable action, it would likely not be the best practice because of the toxic effects of oxygen over time, which can potentially worsen gas exchange.

Full access? Get Clinical Tree
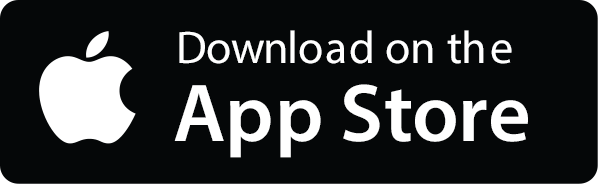
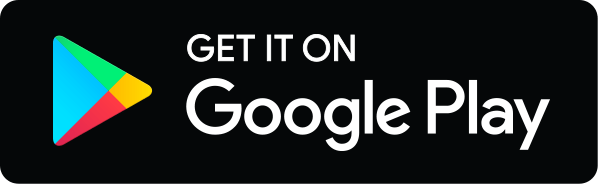