html xmlns=”http://www.w3.org/1999/xhtml” xmlns:mml=”http://www.w3.org/1998/Math/MathML” xmlns:epub=”http://www.idpf.org/2007/ops”>
In the United States, injury is the leading cause of death among individuals between the ages of 1 and 44 years, and the third leading cause of death overall. Approximately 20% to 40% of trauma deaths occurring after hospital admission are related to massive hemorrhage and are therefore potentially preventable with rapid hemorrhage control and improved resuscitation techniques. Over the past decade, the treatment of this population has transitioned into a damage control strategy with the development of resuscitation strategies that emphasize permissive hypotension, limited crystalloid administration, early balanced blood product transfusion, and rapid hemorrhage control. This resuscitation approach initially attempts to replicate whole blood transfusion, utilizing an empiric 1:1:1 ratio of plasma:platelets:red blood cells, and then transitions, when bleeding slows, to a goal-directed approach to reverse coagulopathy based on viscoelastic assays. Traditional resuscitation strategies with crystalloid fluids are appropriate for the minimally injured patient who presents without shock or ongoing bleeding. This chapter will focus on the assessment and resuscitation of seriously injured trauma patients who present with ongoing blood loss and hemorrhagic shock.
The resuscitation of massively bleeding patients has changed markedly over the past century. The use of blood banking and the transfusion of injured patients originated during World War I when it was found to decrease mortality in bleeding and severely injured soldiers.[1] Whole blood was the sole transfusion product until the 1960s and 1970s when component therapy was adopted in the western world.
The next 40 years saw patients resuscitated initially with large volumes of crystalloid and colloid followed by packed red blood cells (RBCs), with limited transfusion of plasma, platelets, and cryoprecipitate based on laboratory values. In hindsight, this approach led to substantial iatrogenic resuscitation injury, and a growing body of literature developed indicating that aggressive resuscitation with large volumes of crystalloid led to cardiac and pulmonary complications, abdominal compartment syndrome, gastrointestinal dysmotility, coagulopathy, and disturbances of immunological and inflammatory mediators.[2]
After major trauma and shock, capillary permeability increases with a resultant decrease in the plasma colloid osmotic pressure and loss of intravascular fluid into the interstitial space. In response to decreased pressure and intravascular hypovolemia, volume returns from the extravascular space in an attempt to re-expand the vascular space and maintain blood pressure.[3,4] The rationale of large-volume crystalloid resuscitation was related to the theory that tissue injury resulted in sequestration of fluid into the traumatized area, with a resultant decrease in extracellular volume that should be replaced with fluids, the so-called “third space concept.” This theory has been refuted by several studies which demonstrated that postoperatively, there is a net “no change” or increase in the extracellular volume.[4–7] Trauma also leads to endothelial cell dysfunction and an increase in pro-inflammatory cytokines, which is exacerbated by pro-inflammatory crystalloid administration, and leads to the initiation of an exaggerated inflammatory response with resultant fluid overload and tissue edema.[8]
In the past decade, there has been a philosophical change in the way severely injured trauma patients are resuscitated which focuses on the early balanced transfusion of blood products and limited crystalloid use. This approach focuses on repairing the endothelium, maintaining intravascular volume, and decreasing edema.
Triage and initial assessment
The triage and initial assessment of injury severity in trauma patients is primarily driven by abnormalities of physiological variables such as blood pressure and heart rate. Basic vital signs are easily obtainable in the field and may provide insight as to whether or not a patient is “clinically stable.” This data is used in the prehospital setting to determine the patient’s mode of transport, destination of treatment, treatment priority, and need for potentially life-saving interventions. When grossly abnormal, vital signs serve as reliable indicators for early diagnostic and therapeutic decision making after injury. However, they can be problematic in many cases as normal physiological compensatory mechanisms may mask the true degree of injury and tissue hypoperfusion. These compensatory mechanisms, especially in young healthy patients, allow for a significant reduction in central circulating blood volume, stroke volume, and cardiac output to occur well before changes in blood pressure develop.[9] Patients may appear “clinically stable” when in fact they are approaching cardiovascular collapse. Hypotension in trauma patients was classically defined as a systolic blood pressure (SBP) of less than 90 mmHg. More recent literature suggests that a SBP of 90 mmHg does not represent the onset of circulatory failure, but rather the clinical manifestation of physiological decompensation associated with advanced hemorrhagic shock and impending cardiovascular collapse. A SBP of <110 mmHg may be a more clinically relevant definition of hypotension in trauma patients as it is at this threshold that tissue hypoperfusion begins to develop, as evidenced by increasing base deficit, with resultant increase in mortality, ICU length of stay, complications, and ventilator days.[10] To overcome the well-described limitations of blood pressure and heart rate, accurate non-invasive methods of delineating the compensated shock state need to be developed and validated.
Intravascular volume depletion, with or without associated hypotension, leads to the physiological state of shock which is characterized by tissue malperfusion and the inability to maintain aerobic metabolism. There are several different mechanisms by which the shock state can be produced after trauma. Hypo-volemia due to massive blood loss is the most likely etiology and the leading cause of death after injury. Other causes must also be considered and rapidly treated, including blunt myocardial damage leading to cardiogenic shock, spinal cord injury with resultant vasodilation and distributive shock, or obstructive shock secondary to tension pneumothorax or pericardial tamponade. Acute blood loss, if not expeditiously treated with hemorrhage control and restoration of intravascular volume, will lead to hemodynamic instability, tissue malperfusion, cellular hypoxia, multisystem organ failure, and death.
The two most commonly used laboratory markers in assessing tissue hypoperfusion and the development of anaerobic metabolism in trauma are serum lactate and base deficit. Both tests are easily obtainable and provide quick results which can help guide resuscitative efforts. Base deficit represents the number of mEq/l of additional base that must be added to a liter of blood to normalize the pH and is calculated directly from the blood gas analyzer from the pCO2, pH, and HCO3− values as applied to a standard nomogram.
Changes in base deficit occur early in hemorrhage and will often precede alterations in hemodynamic parameters such as blood pressure, urine output, and pH.[11] Normal values for base deficit vary among institutions but tend to be greater than −2 mmol/l. A significant base deficit has been shown to be a marker of mortality in several studies.[12–14] Mortality starts increasing when base deficit is >4, and when ≥8 mmol/l has predicted a 25% mortality rate in trauma patients less than 55 years old without head injury.[15] Lactate is a by-product of anaerobic metabolism and is often considered a marker for tissue hypoxia. It is produced by the conversion of pyruvate to lactate after glycolysis by the enzyme lactate dehydrogenase. Although lactate can be elevated in several other conditions, its use to guide resuscitation by lactate clearance has been validated in several studies. Both the initial lactate value and the time to normalization of lactate levels are predictive of mortality, with 100% mortality in ICU patients who fail to achieve normal levels.[16–20]
While blood volume varies with age and physiological state, the average adult blood volume represents approximately 7% of body weight (70 ml/kg of body weight). For a 70 kg individual, estimated blood volume is approximately 5 liters. There are many potential sites of major hemorrhage following injury. Injury to the base of the skull or neck can lead to rapid exsanguination from major vascular structures which are frequently difficult to control and can also lead to difficulty in securing a reliable airway owing to swelling and distortion of normal anatomy. Injuries to the chest can cause parenchymal lung or great vessel injury resulting in hemothorax, which is easily identified with standard chest X-ray. Penetrating cardiac injury can rapidly cause cardiovascular collapse secondary to pericardial tamponade or massive exsanguination. In the peritoneal cavity, hepatic, splenic, and mesenteric injuries can lead to massive hemoperitoneum if not rapidly controlled either in the operating room or interventional radiology suite. Bleeding in the retroperitoneal space from injuries to the great abdominal vessels, renal lacerations, or pelvic fractures with associated damage to pelvic vessels can be difficult to diagnose as ultrasound examination and peritoneal lavage are not reliable diagnostic tools. Extremity injury, such as a penetrating wound or open fracture of the proximal leg or upper arm, may cause massive bleeding from damage to arterial and venous structures. More commonly, and frequently overlooked, a closed fracture of the femur can easily hide 2–3 units of blood without obvious physical exam findings of ongoing bleeding.
In modern trauma centers, facilities and personnel are available to address each of these injuries. When patients present with multiple serious injuries, diagnostic and therapeutic dilemmas arise in properly sequencing the plan of care between advanced imaging studies and operative or angiographic intervention. For this reason, advanced trauma care centers with focused hybrid operating suites are becoming more common, allowing patients to be moved directly from the emergency department to the hybrid resuscitation area where all imaging and interventions can occur simultaneously and in one location. It is very likely that as this capability becomes more common, time to hemostasis after multisystem injury will decrease and survival will improve.
Massive transfusion
Traditionally, massive transfusion has been defined as the administration of 10 or more units of RBCs over a 24-hour period. Several alternative definitions have been proposed, including transfusion of an entire blood volume over 24 hours, 10 units over 6 hours, 5 units over 4 hours, 8 or more units over 12 hours, or 3 or more units over a 1-hour period.[21] Older definitions suffered from significant bias, and have been replaced with rate-based definitions that quantify transfusion requirement over shorter time periods. Regardless of definition, this patient population represents the most critically injured and those most likely to die secondary to massive hemorrhage. In a civilian hospital setting, the incidence of trauma patients receiving massive transfusion ranges between 1% and 3% of all admissions. The mortality of trauma patients who receive massive transfusion is between 20% and 50%.[22–26] Also of critical importance is that these patients die quickly after admission, with the median time to death from hemorrhage of 2 hours.[26] These high mortality rates, especially in those patients who die very soon after admission, are frequently associated with the “lethal triad” that follows severe trauma, characterized by hypothermia, metabolic acidosis, and coagulopathy.[27] Historically, both hypothermia and acidosis were aggressively treated, without a specific focus on coagulopathy. In recent years it has become understood that trauma-induced coagulopathy (TIC) is a multifactorial event which is strongly associated with increased mortality. It is important to promptly recognize those patients who develop TIC, and focus all resuscitative efforts on reversing and preventing any exacerbation of this coagulopathy. The exact etiology of TIC is unclear but the systemic shedding of the glycocalyx and resultant increased permeability of the endothelium is at least partially responsible. Hemorrhagic shock leads to systemic endothelial injury and dysfunction that causes disturbances in coagulation, vascular leak, edema, inflammation, and tissue injury, termed “endotheliopathy of trauma.” Plasma has been shown to repair endothelial tight junctions, decrease paracellular permeability, and restore the glycocalyx, while crystalloids have no effect. The exact mechanism is unknown, but resuscitating with plasma appears to help repair the vascular endothelium, minimizing edema and avoiding the iatrogenic resuscitation injury associated with large volumes of crystalloid administration.[28,29]
Historically, blood product components were infused in a serial fashion. Many liters of crystalloid were infused first, then moving to RBCs and then adding plasma when the entire blood volume was substituted, followed by the addition of platelets when two blood volumes were substituted. This strategy was problematic as the dilution of coagulation factors and platelets by large volumes of crystalloid and RBCs led to further coagulopathy and ongoing bleeding. This transfusion strategy was challenged early in the new millennium, primarily by US military experiences with combat injuries in Iraq, where thawed AB fresh frozen plasma was administered together with RBCs and platelets from the start of resuscitation.[30]
In 2007, Borgman et al. found a survival advantage in massively transfused patients utilizing a balanced transfusion strategy.[31] The transfusion of a 1:1:1 balanced ratio of blood product components (plasma:platelets:RBCs) became the foundation for the strategy described as “damage control resuscitation” (DCR). At the same time that combat surgeons were developing this concept, Johansson and colleagues in Copenhagen were implementing a similar approach, based on their transfusion experience with ruptured aortic aneurysms.[32] The DCR approach has been examined and validated by multiple authors, and has undergone evaluation in single- and multicenter retrospective studies, multicenter observational studies, and recently a prospective and randomized multicenter study.[31,33–35] The pragmatic randomized optimal plasma and platelet ratio (PROPPR) study evaluated 680 patients randomized to either a 1:1:1 or a 1:1:2 ratio. The authors found that there was no mortality difference at 24 hours and 30 days, but more patients in the 1:1:1 group achieved hemostasis and fewer experienced death due to exsanguination by 24 hours. There was a significant difference (p = 0.02) in mortality at 3 hours and this difference persisted over the ensuing 30 days. No other safety differences were identified between the two groups despite the increased use of plasma and platelets transfused in the 1:1:1 group. These results have been viewed favorably and many guidelines now recommend starting transfusion with a 1:1:1 approach in massively bleeding patients.[36]
At our center, the Texas Trauma Institute, we have 4 units of universal donor liquid plasma and RBCs available in the emergency department at all times. If the door is opened to the refrigerator housing these units, the massive bleeding protocol is activated and 6 units of RBCs, 6 units of plasma, and a 6 pack of platelets are delivered automatically to the emergency department. The goal of this protocol is to keep these products readily available for transfusion at the patient’s bedside until hemostasis is achieved. We utilize an active performance improvement process to review each bleeding protocol activation, ensuring optimal efficiency of this critical pathway.
Measuring coagulation
The recent paradigm shift in the resuscitation of massively bleeding patients has also been associated with a change in the way that coagulation parameters in trauma patients are measured. Historically, conventional tests such as international normalized ratio (INR), prothrombin time (PT), partial thromboplastin time (PTT), platelet count, and fibrinogen levels were used to identify those patients with traumatic coagulopathy who were in need of blood product transfusion. These tests are problematic as they were not designed to guide resuscitation efforts or comprehensively describe coagulation function, and are poorly associated with bleeding and transfusion requirements. Furthermore, they are often clinically irrelevant in massively bleeding patients as the results take a prolonged time period to return. The optimal coagulation test used to guide resuscitation in critically injured patients would be a rapid assay based on whole blood that more accurately reflects the integrated function of all aspects of the coagulation system.
The cell-based model of hemostasis, which emphasizes both the importance of tissue factor as the initiator of coagulation as well as cellular elements such as platelets, replaced the classical cascade model of coagulation in the mid-1990s. According to the cell-based model, hemostasis occurs in three phases: initiation, amplification, and propagation, with the magnitude of thrombin generation determining the hemostatic capacity of the formed clot.[37] This integrated model of coagulation is now widely accepted, and the viscoelastic hemostatic assays (VHAs) are felt to reflect this approach more accurately than the conventional tests described above.
VHAs such as thromboelastography (TEG) and rotational thromboelastometry (ROTEM) have historically been used to guide resuscitation in cardiac and transplant centers, and are now frequently being used in the trauma population. These assays produce a tracing based on viscoelastic properties of a whole blood sample and represent components of the coagulation system including plasma proteins and fi-brinogen function, thrombin burst, platelet function, and the fibrinolytic system. Compared with conventional coagulation tests, results are available faster, cheaper, and provide a more global assessment of the functional aspects of intravascular coagulation.[38] They have also been shown to predict increased risk of pulmonary embolism following major trauma.[39] Rapid thromboelastography (r-TEG) can be implemented with computer software packages that allow the tracing to be monitored in real-time in the trauma bay, operating room, or intensive care unit as the test is being performed in the laboratory. This capability provides the clinician with clinically useful data that can be acted upon before the entire test is finalized and reported. When compared with conventional coagulation tests this technology provides more rapid results and has proven to be clinically superior, identifying patients with an increased risk of needing early RBC, plasma, and platelet transfusions, and those with excessive fibrinolysis. With a similar cost profile, it is feasible to replace conventional coagulation tests with VHAs in trauma patients.[31] However, in the rapidly bleeding patient the VHAs still do not return data fast enough for clinical utility. Rates of transfusion easily approach several units every 5 min, and the rate of death from hemorrhagic shock is highest in the first hour after admission. In these patients minutes really do make a difference, and it is in this scenario that the empiric 1:1:1 transfusion approach is utilized. Unfortunately, VHAs do not reveal any direct data about endotheliopathy of trauma and reveal limited information about platelet function. Newer coagulation tests should integrate these important data points, providing a more comprehensive picture of TIC.

Full access? Get Clinical Tree
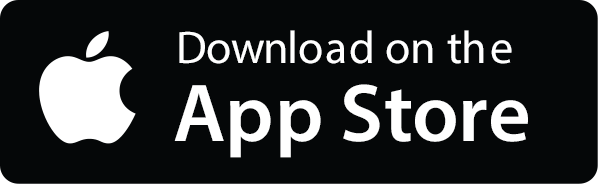
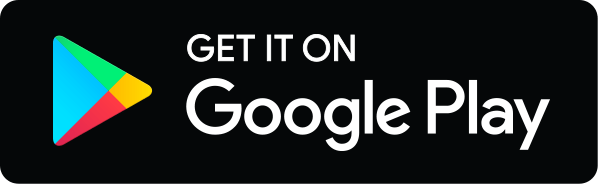