Transposition of the Great Arteries
James A. DiNardo
A 3-day-old, full-term, 3.4-kg newborn is scheduled for an arterial switch operation (ASO). Shortly after birth, he demonstrated reverse differential cyanosis with a right arm arterial saturation of 40% and a lower extremity saturation of 60%, despite prostaglandin E1. A bedside balloon atrial septostomy was performed with resolution of reverse differential cyanosis and improvement of arterial saturation to 75%. Prostaglandin E1 was discontinued 4 hours after the septostomy. Now, he is extubated, receiving 0.5 L per minute supplemental O2 through nasal cannula. His arterial oxygen saturation is 75%; blood pressure, 63/37 mm Hg; pulse, 145 beats per minute; and respiration, 46 breaths per minute.
A. Medical Disease and Differential Diagnosis
What defines the cardiac situs?
What is transposition of the great arteries (D-TGA)?
What additional cardiac lesions are associated with D-TGA?
What is the natural history of D-TGA?
What is the pathophysiology of D-TGA?
What determines the oxygen saturation in patients with D-TGA?
Why did this infant have reverse differential cyanosis?
What are the preoperative issues pertaining to the coronary arteries in D-TGA?
What are the clinical subsets of D-TGA?
What is the differential diagnosis of D-TGA and how is the diagnosis made?
What preoperative interventions can help stabilize a patient with D-TGA?
What are the surgical options for repair? Why is one chosen over the others?
B. Preoperative Evaluation and Preparation
What information is important to prepare for this case?
What are the anesthetic goals before cardiopulmonary bypass (CPB)?
What is the plan for glucose management in this patient?
C. Intraoperative Management
How would you monitor this infant?
What would be the best method of induction?
Is CPB in infants and children different from adults?
What is modified ultrafiltration (MUF)?
Briefly describe the surgical techniques of the Mustard/Senning, ASO, and Rastelli procedures.
What is hypothermia and how is it classified? What is low-flow cardiopulmonary bypass (LFCPB)? What is deep hypothermic circulatory arrest (DHCA)?
Why is hypothermia beneficial to the brain during LFCPB and DHCA?
Explain the difference between α-stat and pH-stat blood gas management.
What are the immediate post-CPB issues following ASO?
D. Postoperative Management
How is myocardial ischemia addressed in the intensive care unit (ICU) following the ASO?
What immediate postoperative problems would be anticipated following the Rastelli procedure?
What intermediate and long-term surgical problems are seen after ASO?
What are the long-term outcomes after ASO?
What are the long-term outcomes after Rastelli repair for D-TGA with left ventricular outflow tract (LVOT) obstruction?
What is long-term neurologic outcome like after ASO?
A. Medical Disease and Differential Diagnosis
A.1. What defines the cardiac situs?
Cardiac situs refers to the orientation of the right atrium; if the right atrium is on its normal position, that is, to the right, the cardiac situs is “solitus” (or normal); otherwise, the cardiac situs is “inversus” (mirror). With the use of echocardiography, the atria, atrioventricular valves (tricuspid and mitral), ventricles, ventriculoarterial valves (pulmonary and aortic), and great arteries (pulmonary artery and aorta) can be identified, thus diagnosing the various cardiac congenital malformations. There are three “axioms,” which help to identify the atria and the ventricles: (1) The right atrium receives the vena cava and coronary sinus; (2) there is always concordance (agreement) between the atrioventricular valve and ventricle, that is, the tricuspid valve is always the inflow valve for the right ventricle and the mitral valve the inflow valve for the left ventricle; and (3) the inflow/atrioventricular (tricuspid) and ventriculoarterial valve of the right ventricle are separated by a muscular part, the conus or infundibulum, but the mitral and ventriculoarterial valve of the left ventricle are in fibrous continuity.
Baum VC, Duncan PN. When right is right and when it’s not: laterality in cardiac structures. Anesth Analg. 2011;113:1334-1336.
Gologorsky E, Gologorsky A, Giquel J, et al. An adult patient with congenitally corrected transposition of the great arteries. Anesth Analg. 2010;111:1122-1124.
A.2. What is transposition of the great arteries (D-TGA)?
D-TGA refers specifically to the anatomic circumstance wherein there is concordance of the atrioventricular (AV) connections associated with discordance of the ventriculoarterial connections. By far the most common manifestation of this anatomy occurs in patients with [S,D,D] segmental anatomy, also known as D-TGA (there is atrial situs Solitus, D-loop ventricles, and D-loop great arteries). A right-sided right atrium (RA) connects through a right-sided tricuspid valve and right ventricle (RV) to a right-sided and anterior aorta. A left-sided left atrium (LA) connects through a left-sided mitral valve and left ventricle (LV) to a left-sided and posterior pulmonary artery (PA). As a result, there is fibrous continuity between the mitral and pulmonic valves with a lack of fibrous continuity (conus) between the tricuspid and aortic valves. In D-TGA, the combination of AV concordance (RA to RV; LA to LV) and ventriculoarterial discordance (RV to aorta; LV to PA) produces a parallel rather than a normal series circulation.
DiNardo JA. Anesthesia for congenital heart surgery. In: Jonas RA, ed. Comprehensive Surgical Management of Congenital Heart Disease. London: Arnold; 2004:45-65.
DiNardo JA. Transposition of the great vessels. In: Lake CL, Booker PD, eds. Pediatric Cardiac Anesthesia. 4th ed. Philadelphia, PA: Lippincott Williams & Wilkins; 2005:357-380.
A.3. What additional cardiac lesions are associated with D-TGA?
The most commonly associated cardiac anomalies are a persistent patent foramen ovale (PFO), patent ductus arteriosus (PDA), ventricular septal defect (VSD), and subpulmonic stenosis or LVOT obstruction. Approximately 50% of patients with D-TGA will present with a PDA before prostaglandin E1 administration. The foramen ovale is almost always patent, but a true secundum atrial septal defect (ASD) exists in only approximately 5% of patients. Although angiographically detectable VSDs may occur in 30% to 40% of patients, only approximately one-third of these defects are hemodynamically significant. Therefore, for practical purposes, 75% of patients have an intact interventricular septum (IVS). LVOT obstruction is present in approximately 30% of patients with VSD and is most often due to an extensive subpulmonic fibromuscular ring or posterior malposition of the outlet portion of the ventricular septum.
Wernovsky G. Transposition of the great arteries. In: Allen HD, Clark EB, Gutgesell HP, et al, eds. Moss and Adams’ Heart Disease in Infants, Children, and Adolescents: Including the Fetus and Young Adult. 6th ed. Philadelphia, PA: Lippincott Williams and Wilkins; 2001:1027-1086.
A.4. What is the natural history of D-TGA?
D-TGA is a common congenital heart lesion accounting for 5% to 7% of all congenital cardiac defects, second in frequency only to isolated VSDs. Without intervention, D-TGA has a high mortality rate: Forty-five percent will die within the first month, and 90% will die within the first year of life. This is particularly unfortunate because infants with D-TGA rarely have extracardiac defects. Advancements in medical and surgical therapy in the last 20 years have greatly improved the outlook for these infants. In fact, following stabilization with prostaglandin E1 and the Rashkind-Miller balloon septostomy, many of these infants can be offered a definite surgical procedure (arterial switch), giving them a quality of life similar to that of normal children.
Hypoxia and intractable congestive heart failure (CHF) are the two primary causes of death. The early onset and progression of pulmonary artery hypertension (PAH) plays a major role in the dismal outlook in these patients. Compared with other forms of congenital heart disease, infants with transposition of great arteries (TGA) are at particular risk for accelerated development of PAH. Systemic hypoxemia, the presence of bronchopulmonary collaterals (which deliver deoxygenated blood to the precapillary pulmonary arterioles), platelet aggregation in the lung, and polycythemia have all been implicated. Infants with TGA and large VSD without LVOT obstruction are at an even higher risk for the early development of PAH due to exposure of the pulmonary vascular bed to increased blood flow and systemic pressures. Advanced, nonreversible pulmonary hypertension reduces the corrective surgical options available to the patient.
DiNardo JA. Transposition of the great vessels. In: Lake CL, Booker, PD, eds. Pediatric Cardiac Anesthesia. 4th ed. Philadelphia, PA: Lippincott Williams & Wilkins; 2005:357-380.
A.5. What is the pathophysiology of D-TGA?
In the parallel arrangement of D-TGA, deoxygenated systemic venous blood recirculates through the systemic circulation without reaching the lungs to be oxygenated. This recirculated systemic venous blood represents a physiologic right-to-left shunt. Likewise, oxygenated pulmonary venous blood recirculates uselessly through the pulmonary circulation. This recirculated pulmonary venous blood represents a physiologic left-to-right shunt. Therefore, in a parallel circulation, the physiologic shunt or the percentage of venous blood from one system that recirculates in the arterial outflow of the same system is 100% for both circuits. Unless there are one or more communications between the parallel circuits to allow intercirculatory mixing, this arrangement is not compatible with life.
The sites available for intercirculatory mixing in D-TGA can be intracardiac (PFO, ASD, VSD) or extracardiac (PDA, bronchopulmonary collaterals). Several factors affect the amount of intercirculatory mixing. The number, size, and position of anatomic communications are important. One large, nonrestrictive communication will provide better mixing than two or three restrictive communications. Reduced ventricular compliance and elevated
systemic and pulmonary vascular resistance (PVR) tend to reduce intercirculatory mixing by impeding flow across the anatomic communications.
systemic and pulmonary vascular resistance (PVR) tend to reduce intercirculatory mixing by impeding flow across the anatomic communications.
The position of the communication is also important. Poor mixing occurs even with large anterior muscular VSDs due to their unfavorable position. Finally, in the presence of adequate intercirculatory mixing sites, the extent of intercirculatory mixing is directly related to the total pulmonary blood flow. Patients with reduced pulmonary blood flow secondary to subpulmonic stenosis or PAH will have reduced intercirculatory mixing.
DiNardo JA. Anesthesia for congenital heart surgery. In: Jonas RA, ed. Comprehensive Surgical Management of Congenital Heart Disease. London: Arnold; 2004:45-65.
DiNardo JA. Transposition of the great vessels. In: Lake CL, Booker PD, eds. Pediatric Cardiac Anesthesia. 4th ed. Philadelphia, PA: Lippincott Williams & Wilkins; 2005:357-380.
A.6. What determines the oxygen saturation in patients with D-TGA?
In D-TGA, intercirculatory mixing is the result of anatomic right-to-left and anatomic left-to-right shunts that are equal in magnitude. The anatomic right-to-left shunt produces effective pulmonary blood flow, which is the volume of systemic venous blood reaching the pulmonary circulation. The anatomic left-to-right shunt produces an effective systemic blood flow, which is the volume of pulmonary venous blood reaching the systemic circulation. Effective pulmonary blood flow, effective systemic blood flow, and the volume of intercirculatory mixing are always equal. The systemic blood flow is the sum of recirculated systemic venous blood plus effective systemic blood flow. Likewise, total pulmonary blood flow is the sum of recirculated pulmonary venous blood plus effective pulmonary blood flow. Recirculated blood makes up the largest portion of total pulmonary and systemic blood flow, with effective blood flows contributing only a small portion of the total flows. This is particularly true in the pulmonary circuit where the total pulmonary blood flow (QP) and the volume of the pulmonary circuit (LA, LV, PA) is three to four times larger than the total systemic blood flow (QS) and the volume of the systemic circuit (RA, RV, aorta). The net result is production of a transposition physiology, where the PA oxygen saturation is greater than the aortic oxygen saturation. Figure 39.1 further elucidates these concepts.
Arterial saturation (SaO2) is determined by the relative volumes and saturations of the recirculated systemic and effective systemic blood flows reaching the aorta. This is summarized in the following equation:

This is illustrated in Figure 39.1 where:
SaO2 = [(50)(1.2)] + [(99)(1.1)] / 2.3 = 73%
Obviously, the greater the effective systemic blood flow (intercirculatory mixing) relative to the recirculated systemic blood flow, the greater the SaO2. For a given amount of intercirculatory mixing and total systemic blood flow, a decrease in systemic venous or pulmonary venous saturation will result in a decrease in SaO2.
DiNardo JA. Anesthesia for congenital heart surgery. In: Jonas RA, ed. Comprehensive Surgical Management of Congenital Heart Disease. London: Arnold; 2004:45-65.
DiNardo JA. Transposition of the great vessels. In: Lake CL, Booker PD, eds. Pediatric Cardiac Anesthesia. 4th ed. Philadelphia, PA: Lippincott Williams & Wilkins; 2005:357-380.
A.7. Why did this infant have reverse differential cyanosis?
In D-TGA with intact IVS, the anatomic mixing sites are usually a PDA and a PFO. The dynamics of intercirculatory mixing in D-TGA with intact IVS are complex. Anatomic shunting at the atrial level is ultimately determined by the size of the atrial communication and the cyclic pressure variations between the left and right atria. The volume and compliance of
the atria, ventricles, and vascular beds in each circuit as well as heart rate (HR) and phase of respiration influence this relationship. Shunting is from the RA to the LA during diastole as the result of the reduced ventricular and vascular compliance of the systemic circuit (RV and systemic arterial circuit). In systole, shunt is from the LA to the RA primarily because of the large volume of blood returning to the LA as a result of the high volume of recirculated pulmonary blood flow.
the atria, ventricles, and vascular beds in each circuit as well as heart rate (HR) and phase of respiration influence this relationship. Shunting is from the RA to the LA during diastole as the result of the reduced ventricular and vascular compliance of the systemic circuit (RV and systemic arterial circuit). In systole, shunt is from the LA to the RA primarily because of the large volume of blood returning to the LA as a result of the high volume of recirculated pulmonary blood flow.
The direction of shunting across the PDA largely depends on the PVR and the size of the intra-atrial communication. When the PVR is low and the intra-atrial communication is nonrestrictive, shunting is predominantly from the aorta to the PA through the PDA (effective pulmonary blood flow) and predominantly from the LA to RA across the atrial septum (effective systemic blood flow). When PVR is elevated, shunting across the PDA is likely to be bidirectional that in turn encourages bidirectional shunting across the atrial septum. When PVR is high and PA pressure exceeds aortic pressure, shunting at the PDA will be predominantly from the PA to the aorta. This will create reverse differential cyanosis—physiology wherein the preductal arterial saturation (right arm) is lower than the postductal arterial saturation (legs). This physiology is usually the result of a restrictive atrial communication producing left atrial hypertension and is associated with low effective blood flows (poor mixing) and hypoxemia. A balloon atrial septostomy can be lifesaving in this setting. Decompression of the LA promotes mixing at the atrial level and also reduces PVR and PA pressure promoting mixing at the PDA. Other causes of reverse differential cyanosis
to be considered in D-TGA are the presence of an interrupted aortic arch or severe aortic coarctation.
to be considered in D-TGA are the presence of an interrupted aortic arch or severe aortic coarctation.
DiNardo JA. Anesthesia for congenital heart surgery. In: Jonas RA, ed. Comprehensive Surgical Management of Congenital Heart Disease. London: Arnold; 2004:45-65.
DiNardo JA. Transposition of the great vessels. In: Lake CL, Booker PD, eds. Pediatric Cardiac Anesthesia. 4th ed. Philadelphia, PA: Lippincott Williams & Wilkins; 2005:357-380.
A.8. What are the preoperative issues pertaining to the coronary arteries in D-TGA?
As in normally related great vessels, the coronary arteries in D-TGA arise from the aortic sinuses that face the PA. In normally related vessels, these sinuses are located on the anterior portion of the aorta, whereas in D-TGA, they are located posteriorly. In most D-TGA patients (70%), the right sinus is the origin of the right coronary artery, whereas the left sinus is the origin of the left main coronary artery. In the remainder of cases, there is considerable variability, with the most common variations being shown in Figure 39.2.
Most patients with D-TGA have a coronary anatomy that is suitable for the coronary reimplantation necessary in the ASO. Patients with certain types of coronary anatomy (intramural coronaries, single coronary artery) are at risk for postoperative myocardial ischemia and early mortality because reimplantation can result in the distortion of the coronary ostia or the narrowing of the artery itself. Patients with intramural coronaries generally require resuspension of the posterior leaflet of neopulmonary valve once the coronaries and a surrounding tissue cuff are excised.
The presence of a single coronary artery or intramural coronary arteries is a risk factor for mortality, and this risk has persisted over the last two decades.
DiNardo JA. Transposition of the great vessels. In: Lake CL, Booker PD, eds. Pediatric Cardiac Anesthesia. 4th ed. Philadelphia, PA: Lippincott Williams & Wilkins; 2005:357-380.
A.9. What are the clinical subsets of D-TGA?
Four clinical subsets based on anatomy, pulmonary blood flow, and intercirculatory mixing can be used to characterize patients with D-TGA. These are summarized in Table 39.1.
A.10. What is the differential diagnosis of D-TGA and how is the diagnosis made?
Figure 39.3 delineates the differential diagnosis for D-TGA in the cyanotic newborn.
D-TGA may be associated with either cyanosis or CHF. In patients in whom intercirculatory mixing is limited, cyanosis is severe with little evidence of CHF. CHF is the more common finding in patients with increased pulmonary blood flow, a large quantity of intercirculatory mixing, and mild cyanosis.
Chest radiographs may appear normal in the first few weeks of life in infants with D-TGA and intact IVS. Eventually, the triad of an enlarged egg-shaped heart (large RA and RV), narrow superior mediastinum, and increased pulmonary vascular markings evolve. In patients with D-TGA and VSD without LVOT obstruction, a large cardiac silhouette and prominent pulmonary vascular markings are seen at birth. Right axis deviation and right ventricular hypertrophy are the electrocardiographic (ECG) findings in D-TGA with intact IVS, whereas right axis deviation, LV hypertrophy, and RV hypertrophy are seen with D-TGA and VSD.
Two-dimensional echocardiography is the diagnostic modality of choice in the diagnosis and assessment of infants with D-TGA. It accurately establishes the diagnosis of D-TGA and reliably identifies associated abnormalities such as VSD, mitral and tricuspid valve abnormalities, and LVOT obstruction. It also reliably delineates coronary artery anatomy. Echocardiographic analysis of the IVS position or LV geometry is also used to noninvasively assess the LV to RV pressure ratio and IV mass in neonates with D-TGA and IVS who are being evaluated as candidates for an ASO.
In institutions with high-level echocardiography, a comprehensive cardiac catheterization is no longer routinely performed in neonates with D-TGA. A limited catheterization may be performed in conjunction with a balloon atrial septostomy. In the rare instance where coronary anatomy cannot be clearly delineated by echocardiography, coronary angiography may be indicated. During catheterization of infants with PAH, a trial of ventilation at an FIO2 of
1.0 may be used to determine whether PVR is fixed or remains responsive to oxygen-induced pulmonary vasodilatation.
1.0 may be used to determine whether PVR is fixed or remains responsive to oxygen-induced pulmonary vasodilatation.
TABLE 39.1 Clinical Subsets of Transposition of Great Arteries | ||||||||||||||||||||||||
---|---|---|---|---|---|---|---|---|---|---|---|---|---|---|---|---|---|---|---|---|---|---|---|---|
|
DiNardo JA. Anesthesia for congenital heart surgery. In: Jonas RA, ed. Comprehensive Surgical Management of Congenital Heart Disease. London: Arnold; 2004:45-65.
DiNardo JA. Transposition of the great vessels. In: Lake CL, Booker PD, eds. Pediatric Cardiac Anesthesia. 4th ed. Philadelphia, PA: Lippincott Williams & Wilkins; 2005:357-380.
A.11. What preoperative interventions can help stabilize a patient with D-TGA?
Intact Ventricular Septum
Most neonates with D-TGA and intact IVS will be hypoxemic (arterial saturation ≤60%) within the first day of life. A proportion of these patients will have severely reduced effective pulmonary and systemic blood flow resulting in a PaO2 less than 20 mm Hg, hypercarbia, and an evolving metabolic acidosis secondary to the poor tissue oxygen delivery. Prostaglandin E1 (0.01 to 0.05 µg/kg/min) is administered to dilate and maintain the patency of the ductus arteriosus. This will be effective in increasing effective pulmonary and systemic blood flow and in improving PaO2 and tissue oxygen delivery if (1) PVR is less than systemic vascular resistance (SVR) and (2) there is a nonrestrictive or minimally restrictive atrial septal communication. In some centers, all neonates stabilized on prostaglandin E1 alone have a balloon atrial septostomy to enlarge the atrial septal communication so that prostaglandin E1 can be stopped and surgery scheduled on a semi-elective basis. Prostaglandin E1 infusion is associated with apnea, pyrexia, fluid retention, and platelet dysfunction.
If prostaglandin E1 does not improve tissue oxygen delivery, then an emergent balloon atrial septostomy is performed in the catheterization laboratory utilizing angiography or in the ICU utilizing echocardiography. These patients also require tracheal intubation and mechanical ventilation. This allows reduction of PVR through induction of a respiratory alkalosis and elimination of pulmonary ventilation/perfusion ([V with dot above]/[Q with dot above]) mismatch. Sedation and muscle relaxation reduce oxygen consumption, thereby increasing mixed venous O2 saturation. Recall that for a given amount of intercirculatory mixing and total systemic blood flow, an increase in systemic venous or pulmonary venous saturation will result in an increase in arterial saturation.

Full access? Get Clinical Tree
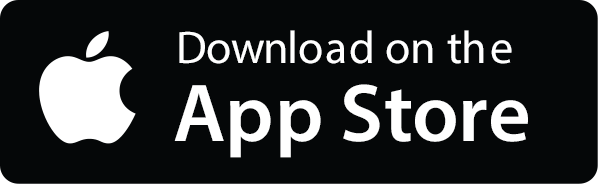
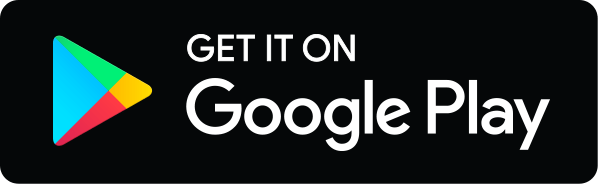
