Hemoglobin and Volume Replacement Therapies
Historical Aspects of Transfusion
The observation by Harvey in 1628 that blood circulates in a closed vascular system was pivotal in the practice of blood transfusion. As blood was recognized as vital to sustain life, Denis in Paris and Lower in Oxford attempted xeno-transfusion (animal blood to humans) with little success in the 17th century. The first documented transfusion of human blood was made in 1818 by Blundell, an obstetrician in London, who recognized the need for transfusion in women suffering from postpartum hemorrhage. His results were not reproducible owing to the lack of knowledge of blood types and anticoagulants.
Major advances were made in the beginning of 20th century when Landsteiner identified blood groups A, B, and C (later renamed group O ). Transfusion of fresh whole blood was reported as a treatment for epistaxis due to thrombocytopenia in 1910. Storage and distribution of donated blood became possible after sodium citrate was developed as an anticoagulant during World War I. The infrastructure of the modern blood banking system was established by World War II; use of fresh whole blood transfusion saved many wounded soldiers. However, the risk of pathogen transmission, including hepatitis via blood transfusion, was recognized later.
During World War II, Cohn and colleagues developed the cold ethanol method to separate albumin, γ-globulin, and fibrinogen from plasma, which became the basic principle for commercial plasma fractionation. Pooling of random donor plasma to manufacture factor (F) VIII concentrates without effective donor screening or virus inactivation steps in the late 1970s and early 1980s led to transfusion-related transmission of viruses, particularly human immunodeficiency virus (HIV) and hepatitis C to a large number of hemophiliac patients worldwide. Many precautions for blood-borne pathogens have been implemented, including vapor heat treatment and nanofiltration, since the mid-1980s; recombinant coagulation factors became available in the 1990s. The risk of infectious transmission also fueled efforts to develop synthetic oxygen carriers.
Over the years, clinicians have recognized the importance and potential harms of blood component therapies, fostering the creation of patient blood management programs. Preoperative anemia management is a critical part of patient blood management; iron supplementation and/or erythropoietin therapy can be used in appropriate patient groups. Perioperative cessation of antithrombotic agents, intraoperative blood salvage, and antifibrinolytic therapy are also utilized to minimize the need for allogeneic blood transfusion along with point-of-care coagulation monitors. The rational and cost-effective uses of blood components are now the subject of published transfusion guidelines.
Packed Red Blood Cells
Circulating red blood cells (RBCs) are anuclear, hemoglobin-carrying cells of about 7 to 8 µm in diameter. The biconcave and discoid shape of RBCs confers advantages in increasing surface area for gas exchange and flexibility in passing through capillaries. On average, RBCs remain in the circulation for 120 days. Senescent or abnormal RBCs are eliminated by the spleen. The synthesis of RBCs (erythropoiesis) is regulated by erythropoietin, which is elevated in anemia and hypoxia. Erythropoiesis is also affected by the availability of iron, an integral component of hemoglobin.
Packed RBCs (PRBCs) are prepared by separating most plasma components of donated whole blood by centrifugation. One unit of RBCs collected in anticoagulant-preservative solution is about 300 mL with a hematocrit of 50% to 70%. Dextrose is added to maintain glucose metabolism, and adenine and phosphate allow synthesis of adenosine triphosphate (ATP; Table 44.1 ). Additive solutions (adenine, glucose, mannitol, sodium chloride) are also used to extend shelf life up to 42 days. Depending on the storage solution, RBCs have a shelf life of 28 to 42 days at 1°C to 6°C. During storage, intracellular 2,3-diphosphoglycerate (DPG) is reduced to < 10% of normal at 5 weeks such that release of oxygen from hemoglobin is significantly impaired ( Fig. 44.1 ). Nonetheless, no differences in morbidity and mortality were detected between short-term storage (6–11 days) and long-term storage (21–28 days) in prospective randomized studies in premature infants, adult cardiac surgical patients, and critically ill adult subjects.
Component | Volume | Shelf Life | Storage Temperature |
---|---|---|---|
Red blood cells | 220–340 mL | 42 days | 1–6°C |
Apheresis platelets | 250–300 mL | 5 days | 20–24°C |
Random donor platelets | 50–70 mL | 5 days | 20–24°C |
Plasma | 200–250 mL | 1 y | < −18°C |
Cryoprecipitate | 10–15 mL | 1 y | < −18°C |

Clinical Uses
Transfusion of RBCs is indicated to restore the oxygen-carrying capacity in patients with severe anemia or major blood loss. There is no absolute level of hemoglobin that indicates a threshold for transfusion (“transfusion trigger”); underlying clinical conditions and laboratory data for each patient should be considered. Acute anemia is less well tolerated compared to chronic anemia, in which peripheral oxygen delivery is compensated by elevated 2,3-DPG levels in RBCs and higher cardiac output (see Fig. 44.1 ). In patients with moderate to severe cardiovascular dysfunction, anemia might not be well tolerated. The extent and duration of clinical bleeding are also important criteria for transfusion in major trauma and surgery. According to the American Society of Anesthesiologists (ASA) guidelines for blood component therapy, transfusion of RBCs is almost always indicated for hemoglobin less than 6 g/dL, whereas it is rarely indicated for hemoglobin greater than 10 g/dL. For hemoglobin between 6 g/dL and 10 g/dL, transfusion should be considered if complications due to inadequate oxygenation are anticipated. It remains controversial as to whether liberal or restrictive transfusion strategy is preferable in various surgical and medical settings. Restrictive transfusion (hemoglobin triggers < 7–8 g/dL) appears to increase mortality and ischemic events relative to a liberal strategy (triggers < 9–10 g/dL) in cardiovascular surgery patients and elderly orthopedic surgical patients. There was no difference in adverse event rates between liberal and restrictive strategies in nonsurgical critically ill patients.
ABO and Rh blood group–specific packed RBCs should be administered whenever possible ( Fig. 44.2 ). In the case of life-threatening hemorrhage without time for formal cross-matching, either Rh-positive or Rh-negative group O RBCs can be transfused in principle. In Rh-negative women of childbearing age, Rh-negative group O RBCs should be preferred for reducing the risk of alloantibody (anti-D) development, which can cause anemia in an Rh-positive fetus. Transfusion of mismatched RBCs, typically related to ABO, can induce immunoglobulin M (IgM) antibody–mediated acute hemolysis, but in less serious mismatches of minor antigens such as Rh, Kell, and Duffy, survival of transfused RBCs is shortened.

Side Effects
Acute hemolytic transfusion reactions are usually caused by ABO incompatibility. This potentially fatal complication occurs in about 1 in 30,000 transfusions. As little as 20 to 30 mL of incompatible RBCs can cause agitation, nausea/vomiting, dyspnea, fever, flushing, hypotension, tachycardia, and hemoglobinuria. Two major complications of intravascular hemolysis include renal failure from acute tubular necrosis and disseminated intravascular coagulation (DIC).
Febrile nonhemolytic transfusion reaction is relatively common (0.1–1% of RBC transfusions). Other immunologic complications of transfusion include human leukocyte antigen (HLA) alloimmunization, graft-versus-host disease, and immunosuppression triggered by donor leukocytes. The risk of these complications can be partially reduced by use of leukocyte adsorption (leukoreduction) filters at blood collection and γ-irradiation after collection to prevent lymphocyte proliferation. The leukoreduction process presumably reduces virologic risks associated with leukocytes, including cytomegalovirus, Epstein-Barr virus, and human T-cell leukemia virus I and II. Transmission of emerging virus (e.g., Zika) through transfusion is possible; thus, careful donor screening and implementation of available pathogen reduction techniques are recommended. Although transfusion-related variant Creutzfeldt-Jakob diseases (vCJDs) and bovine spongiform encephalopathy (BSE) are rare, there is a theoretical advantage in leukodepletion to prevent prion transmission.
Erythropoietin
Erythropoietin is a hormone produced in the kidney in response to hypoxemia, such as in chronic pulmonary disease and at high altitudes. Recombinant human erythropoietin products are available for intravenous (IV) or subcutaneous (SC) injection. The primary indication for recombinant erythropoietin is anemia associated with chronic renal insufficiency. Other indications for erythropoietin include treatment of anemia related to the antiviral zidovudine in HIV-infected patients and treatment of chemotherapy-induced anemia in patients with metastatic, nonmyeloid malignancies. Preoperative erythropoietin treatment of anemia has been shown to reduce allogeneic RBC transfusion, but its high cost hinders easy access for perioperative anemic patients. There are a variety of perioperative dosing regimens in conjunction with iron supplementation. One example is a weekly dose of 600 units/kg SC started 3 weeks before surgery and the 4th dose given on the day of surgery. A single dose of 80,000 IU SC dose 2 days before cardiovascular surgery reduced the overall transfusion rate from 39% to 17% in a prospective randomized placebo-controlled study involving 600 patients.
Side Effects
Erythropoietin therapy induces a marked expansion of erythroid cells, which can result in iron deficiency; thus, iron should be supplemented as appropriate. Target hemoglobin levels should not be set above 13 g/dL because the risk for cardiovascular events and stroke is increased. Hypertension can be worsened by erythropoietin, especially in patients with chronic renal failure. Erythropoietin therapy can adversely affect the survival of cancer patients, with progression or recurrence of certain tumors.
Blood Substitutes
The limited availability of blood and infection risks are the driving forces in developing oxygen-carrying blood substitutes. Two major classes of substitutes are hemoglobin-based oxygen carriers (HBOC) and perfluorocarbon (PFC) emulsions. Bovine-derived hemoglobin glutamer-200 (Oxyglobin, Biopure, Cambridge, MA) is currently approved for canine anemia in the United States. In South Africa, bovine hemoglobin glutamer-250 (Hemopure, Biopure, Cambridge, MA) is approved for treatment of human anemia. Free hemoglobin solutions have a higher affinity for oxygen compared to RBCs (P 50 of 12–14 mm Hg for HBOC vs. 27 mm Hg for RBCs). The modification of hemoglobin by pyridoxal phosphate increases P 50 to 32 mm Hg, improving release of oxygen. Potential benefits of HBOCs include sparing allogeneic RBC transfusion in anemia, trauma, and major surgery, and reduction of transfusion-related infection and other complications. Compassionate use of HBOC has been reported in a Jehovah’s Witness patient with acute lymphoblastic leukemia who survived severe anemia (lowest hemoglobin, 3.1 g/dL). However, vasoconstrictive properties of HBOC remain a major concern for further clinical development.
PFC emulsions consist of halogen-substituted hydrocarbons that enhance plasma oxygen solubility. Hydrophobic PFC molecules are dissolved in plasma using emulsifiers. Unlike hemoglobin, the oxygen-carrying capacity of PFC is directly proportional to oxygen partial pressure. Febrile reactions or flu-like symptoms can occur as PFC emulsion is taken up by the reticuloendothelial system. PFC is not clinically available at present, but potential perioperative uses include acute lung injury and acute normovolemic hemodilution to spare RBCs from extracorporeal circuits.
Albumin
Albumin is a 69-kDa plasma protein synthesized in the liver and present in plasma at 3 to 5 g/dL (~60% of plasma protein). Plasma-derived, pasteurized (60°C for 10 hours) fractions are available in iso-oncotic (5%) or hyper-oncotic (25%) solutions. Albumin exerts oncotic activity (colloid osmotic pressure) to retain intravascular water. The oncotic pressure of 5% albumin is equivalent to plasma. Albumin also serves to carry poorly water-soluble molecules (apoprotein, bilirudin, transferrin, and so on) and can bind a number of drugs. In hypovolemic patients, there is no evidence that albumin reduces mortality compared with crystalloid or colloid solutions. There are a few indications for albumin use, including fluid replacement for hypovolemic shock with lack of response to crystalloids or colloids, priming for extracorporeal circuits, and plasma exchange therapy. In acute liver failure, albumin can be used to restore oncotic pressure. The intravascular retention of albumin is affected by increased vascular permeability (e.g., first 24 hours of thermal injury) and excretion (e.g., nephrotic syndrome). Albumin does not affect hepatic or renal function.
Synthetic Colloids
Synthetic colloid solutions include hydroxyethylstarches (HESs), in which rapid degradation by α-amylase is prevented by hydroxyethylation of glucose subunits ( Fig. 44.3 ). The molar replacement ratio indicates the proportion of glucose molecules replaced with hydroxyethyl units (e.g., 0.4 = 40% replacement). The C2/C6 ratio indicates the number of hydroxyethyl units at the C2 relative to the C6 position. HES with higher molar replacement and C2/C6 ratios is retained longer owing to slower metabolism ( Table 44.2 ). HES is excreted by the kidneys after degradation.

Type of HES | 600/0.7 | 670/0.75 | 260/0.45 | 130/0.4 |
---|---|---|---|---|
Product name | Hespan | Hextend | Pentaspan | Voluven |
Concentration | 6% | 6% | 10% | 6% |
Solvent | Saline | LR | Saline | Saline |
Oncotic pressure (mm Hg) | 25–30 | 25–30 | 55–60 | 36 |
Mean molecular mass (kDa) | 600 | 670 | 260 | 130 |
Molar substitution | 0.7 | 0.75 | 0.45 | 0.4 |
C2/C6 ratio | 5 | 4.5 | 6 | 9 |
Maximum daily dose (mL/kg) | 20 | 20 | 20 | 33 |
Differences between albumin and HES in efficacy and safety are controversial. HES products are available in iso-oncotic (6%) or hyper-oncotic (10%) solutions. The most commonly used are 6% HES solutions, with an average molecular weight of 600 to 670 kDa (Hespan, Hextend, B. Braun, Bethlehem, PA), but low-molecular-weight HES (130 kDa, Voluven, Fresenius Kabi, Halden, Norway) has recently become available in the United States. HES products are as effective as albumin as fluid replacements, but large doses of HES (particularly, Hespan) can adversely affect coagulation (fibrin polymerization) and exacerbate renal dysfunction in sepsis. Excess HES can falsely elevate turbidometric fibrinogen measurements. Recently, the European Medical Association has recommended suspending marketing authorization for HES colloids in patients with sepsis or at risk for renal insufficiency. Colloid replacement therapy might still be indicated in early volume resuscitation after acute blood loss (e.g., trauma).
Hemostatic Interventions and Coagulation Therapies
Platelet Concentrates
Platelets are anuclear, granulated cells 2 to 4 µm in diameter derived from bone marrow megakaryocytes. The normal half-life of platelets is 7 to 10 days. There are 150 to 350 × 10 9 /L platelets in the circulation, but their concentration near arterial vessel walls is significantly higher owing to the margination of platelets by larger RBCs. Platelets rapidly respond to disruption of normal endothelium, contributing to the initial arrest of bleeding (primary hemostasis), and support of localized thrombin generation and fibrin formation (secondary hemostasis or coagulation; Fig. 44.4 ).

Since the first platelet transfusion in the 1950s, platelet concentrate remains the mainstay therapy for thrombocytopenia. Platelet concentrates are prepared by centrifugation of citrated whole blood within 8 hours of collection. After separating RBCs from platelet-rich plasma, further centrifugation yields 1 unit of platelet concentrate and plasma. Each unit of platelets, referred to as random-donor platelets , contains 5.5 × 10 10 platelets in 50 to 70 mL of plasma. Four to 8 random-donor units are pooled to increase platelet count by 5 to 10 × 10 9 /L in the adult. In order to decrease multiple donor exposures, single-donor platelet apheresis is increasingly used. During the apheresis procedure, donor blood is placed in the extracorporeal circuit and centrifuged to separate platelets. One platelet apheresis unit contains 30 to 50 × 10 10 platelets in 250 to 300 mL of plasma. Platelet concentrates are agitated and stored at room temperature (20–24°C) for up to 5 days (see Table 44.1 ).
Clinical Uses
Platelet transfusion is used to prevent or treat bleeding due to platelet dysfunction or thrombocytopenia. Hereditary platelet dysfunction is rare, but adhesion defects in Bernard-Soulier syndrome (GPIb/IX deficiency), aggregation defects in Glanzmann thrombasthenia (GPIIb/IIIa deficiency), and a secretion defect in Hermansky–Pudlak syndrome (lack of dense granules) are prototypical hemorrhagic conditions resulting from decreased primary hemostasis. Platelet dysfunction in perioperative patients is usually due to antiplatelet therapy (see Chapter 45 ). Platelet transfusion can be required even with a normal platelet count if platelet dysfunction is clinically suspected or identified by platelet function testing.
Perioperative platelet dysfunction is most commonly caused by acetylsalicylic acid (aspirin), thienopyridines, or GPIIb/IIIa inhibitors. For thrombocytopenia associated with bone marrow disorders, prophylactic platelet transfusion for a threshold below 10 × 10 9 /L appears to reduce the risk of serious bleeding events. The recommended thresholds for platelet transfusion for nonbleeding, critically ill patients are also in the similar range (10–20 × 10 9 /L). The trigger platelet count usually falls in the range of 50–100 × 10 9 /L in trauma or major surgery. The trigger values are generally higher for neurosurgical and cardiovascular procedures (> 100 × 10 9 /L). For other procedures, such as lumber puncture or central venous catheterization, a threshold of platelet count between 20 and 50 × 10 9 /L is used, but supporting evidence is rather weak.
Platelet transfusion is administered to mitigate microvascular bleeding due to thrombocytopenia or platelet dysfunction, but hypofibrinogenemia and hyperfibrinolysis need to be addressed separately as the latter are important causes of perioperative bleeding. It is often difficult to quantify hemostatic efficacy of platelet transfusion in the presence of multifactorial factor deficiency after major trauma or surgery. Platelet count does not reliably reflect functional recovery in the presence of platelet inhibitors and other pathologic conditions (e.g., extracorporeal circuit) that adversely affect platelet function. The timing and amount of platelet transfusion can be individualized using platelet function testing and/or thromboelastometry/thromboelastography ( Fig. 44.5 ). Modified thromboelastometry/thromboelastography assays suitable for monitoring antiplatelet agents have been developed, but further clinical validations are warranted for the standardization and optimal thresholds for transfusion.

Side Effects
The risk of bacterial contamination is higher with platelet concentrates than other blood components since they are stored at room temperature. An immunoassay kit is available to test for aerobic or anaerobic gram-positive and gram-negative bacteria (sensitivity, 10 3 –10 5 colony formation units/mL). The lack of virus reduction procedures for platelet concentrates has been a major concern, and transmissions of Zika virus via platelet transfusion were recently reported. A recently US Food and Drug Administration (FDA)–approved pathogen- reduction system (Intercept Blood System, Cerus Corp., Concord, CA) uses the combination of amotosalen and ultraviolet A light to block DNA and RNA replication of various pathogens in plasma and platelet concentrates. Further clinical validations are needed as to its indications and efficacies against emerging pathogens.
Alloimmunizations after platelet transfusion can result in antibodies against HLA class I antigens and platelet-specific antigens. Problems due to platelet alloimmunization include refractoriness to platelet transfusion and post-transfusion purpura. For those who require frequent platelet transfusions, the use of leukoreduced, HLA-matched, and ABO-compatible platelet units should be considered to reduce the risk of platelet alloimmunization. Hemolysis of RBCs can be caused by anti-A or anti-B antibody present in donor plasma of platelet concentrates. This typically occurs after group O platelet transfusion in non-group O recipients. The incidence of severe hemolysis is estimated to be in the range of 1 in 6600 (apheresis platelet transfusion) to 1 in 9000 (non–ABO-identical platelet transfusions).
Other potential complications of platelet transfusion in surgical patients include transfusion-associated lung injury (TRALI) and thrombotic complications. Last, it is unclear whether there is a clinical difference in hemostatic efficacy and safety between pooled platelet concentrates and apheresis platelets. The increment in platelet count was higher after apheresis platelets compared to pooled concentrates, but without impacting clinical bleeding in hemato-oncology–related thrombocytopenia. Similarly, the clinical relevance of platelet preparation for bacterial contamination and donor exposure remains unclear.
Plasma
Plasma transfusion has been traditionally performed using fresh frozen plasma (FFP). FFP is prepared from whole blood (~500 mL) or apheresis donations and frozen at –18°C within 8 hours of collection. However, other plasma products have been increasingly used to make up the shortfall in plasma supply in the United States. Plasma frozen between 8–24 hours of phlebotomy (FP24) is a unit of plasma containing similar factor levels to FFP except for factor VIII (FVIII, up to 20% reduction). Each unit of FFP or FP24 is approximately 200 to 250 mL, and contains all components of donor plasma, including procoagulant and anticoagulant factors, albumin, and immunoglobulins. The recovery of coagulation factors after each plasma unit is about 2% to 3% in the adult but can vary with donors, clinical hemorrhage, and/or ongoing consumption. Plasma products can be stored frozen up to 12 months. Thawed plasma (FFP and FP24) are maintained in the liquid state for up to 5 days at 1°C to 6°C for clinical use, but some degradations of plasma factors do occur, particularly for FV and FVIII. Donor plasma should be compatible with recipients’ ABO types (see Fig. 44.2 ), but Rh types do not need to be considered. Blood group AB and A plasma have been used as universal donors for emergency.
Solvent-Detergent (S/D) Plasma
Octaplas LG (Octapharma AG, Lachen, Switzerland) is a commercial S/D plasma licensed in many countries, including the United States. Large pools of donor plasma are collected in a blood type–specific batch (280 L from ~1500 donors) from countries at low risk for vCJD. The plasma pool is subsequently treated for 1 to 1.5 hours at 30°C (previously 4–4.5 hours) with a combination of solvent, 1% tri(n-butyl)phosphate (TNBP), and a detergent (1% Octoxynol-9), inactivating enveloped viruses. After extraction of S/D reagents, the product solution is passed through an affinity ligand column for intended removal of prions. The processes are presumed to remove emerging virus (e.g., Zika). The filtered plasma is aliquoted in 200-mL bags, and frozen to below −65°C. Octaplas LG is stored at < 18°C, and a specific type (A, B, AB, O) of bag is thawed before transfusion. The production steps decrease the levels of protein S and α 2 -plasmin inhibitor in the final plasma batch, but the duration of S/D treatment has recently been reduced, resulting in less significant deviations of protein S and α 2 -plasmin inhibitor from normal ranges in Octaplas LG compared to the older products.
In a study comparing cryosupernatant (250 mL/bag) and Octaplas (200 mL/bag) in therapeutic plasma exchange (TPE) for thrombotic thrombocytopenic purpura (TTP), there was no statistical difference in the number of TPE treatments from admission to clinical remission between products. Citrate reactions (paresthesia, muscle cramps) were less frequent with Octaplas (6.9% vs. 18%) than with cryosupernant and, more important, allergic reactions were less common with Octaplas (3.1% vs. 9.3%). The risk of transfusion-related acute lung injury is also reduced with S/D plasma because anti-HLA/anti-granulocyte antibodies in donor units are significantly diluted by pooling of donor plasma.
Third-generation S/D plasma is not blood-type specific (Uniplas LG, Octapharma). Uniplas/UniplasLG can be transfused universally in any recipient because anti-A and anti-B antibodies are removed. Other features of Uniplas LG are similar to Octaplas LG.
Lyophilized Plasma
Lyophilized (freeze-dried) plasma (LyoPlas N-w) is available in several countries. This blood type–specific, single-donor plasma is produced by the German Red Cross (DRK-Blutspendedienst West). Donor plasma is tested for viral hepatitis and parvovirus B19, and type-specific plasma (A, B, AB, O) is kept frozen in quarantine until the donor is retested negative after a minimum of 4 months. After the quarantine, frozen single plasma units are thawed and filtered to eliminate blood cells and cell debris, reducing the risk of leukocyte-associated cytomegalovirus (CMV) transmission. The bottles of plasma are sealed and refrozen (< −30°C) and subsequently dehydrated under vacuum and gradually rising temperature.
The French Military Blood Institute (FMBI) has manufactured French lyophilized plasma (FLyP) since 1994. For the production of FLyP, apheresis plasma is collected from male/female donors who fulfill rigorous medical criteria. Leukoreduced plasma undergoes pathogen reduction steps, including amotosalen and ultraviolet light (Intercept Blood System). Mixing of 3000 mL of pathogen-inactivated plasma from a maximum of 10 donors (mixture of A, B, and AB blood types) results in neutralization of anti-A and anti-B hemagglutinins). The plasma is subsequently aliquoted in an individual flask and freeze-dried over 4 days.
The major advantage of this lyophilized plasma is that it can be kept at room temperature (range, 2–25°C) for 15 months (LyoPlas N-w) or 2 years (FLyP). Each bottle of powdered plasma is reconstituted with 200 mL of sterile water before transfusion. These plasma products can be transported to locations where a steady supply of safe plasma is not feasible. Type AB or universal lyophilized plasma can be stocked for emergencies. One study reported that FV and FVIII are 25% and 20% lower in FLyP than in FFP, respectively, but other factor levels, endogenous thrombin generation potential, and thromboelastometry profile are comparable between the two.
Clinical Uses
Plasma transfusion is mainly indicated for treatment of complex coagulopathies in which multiple coagulation factors and inhibitors are depleted. For congenital factor deficiency, plasma transfusion should be considered only if recombinant or plasma-derived factor concentrate is not available ( Table 44.3 ). Plasma products are sometimes transfused prophylactically before invasive procedures when risk of bleeding is high, but there is limited evidence and no cut-off level for any coagulation test that clearly indicates the need for plasma. When the international normalized ratio (INR) is < 1.5, excessive bleeding is unlikely. Plasma transfusion is frequently used to treat bleeding conditions when prothrombin time (PT) is greater than 1.5 times the midpoint of the normal range or activated partial thromboplastin time (aPTT) is greater than 1.5 times the upper limit of normal.
Concentration (µM) | Half-Life (h) | Available Concentrate(s) | |
---|---|---|---|
Fibrinogen | 7.6 | 72–120 | pd-fibrinogen, cryoprecipitate |
Prothrombin | 1.4 | 72 | PCC, FEIBA |
Factor V | 0.03 | 36 | None |
Factor VII | 0.01 | 3–6 | pd-FVII, r-FVIIa, PCC, FEIBA |
Factor VIII | 0.00003 | 12 | pd-FVIII, r-FVIII |
Factor IX | 0.09 | 24 | pd-FIX, r-FIX, FEIBA |
FX | 0.17 | 40 | pd-FX, PCC, FEIBA |
Factor XI | 0.03 | 80 | pd-FXI |
Factor XIII | 0.03 | 120–200 | pd-FXIII, r-FXIII, cryoprecipitate |
vWF | 0.03 | 10–24 | pd-vWF, r-vWF, cryoprecipitate |
Protein C | 0.08 | 10 | pd-Protein C, PCC |
Protein S | 0.14 | 42.5 | PCC |
Antithrombin | 2.6 | 48–72 | pd-antithrombin, r-antithrombin |
The most commonly used plasma dose is 2 units for adults, which amounts to 5 to 8 mL/kg. However, factor increments are generally 10% even after 12 mL/kg of plasma transfusion, and 15 to 30 mL/kg might be necessary to keep coagulation factors above 50% of normal. Plasma products need to be administered early rather than late to avoid fluid overload in cases of massive hemorrhage. However, larger plasma and platelet doses relative to RBCs (1 : 1 : 1 ratio) failed to improve the survival of major civilian trauma victims (n = 338) compared to lower doses (n = 342).
It is feasible to implement targeted coagulation therapy after the first round of blood products in major trauma patients when rapid viscoelastic coagulation tests are used (see Fig. 44.5 ). Guidelines of the American Association of Blood Banks and the European task force recommend early plasma transfusion without a specific FFP:RBC ratio. For acute reversal of vitamin K antagonist (e.g., warfarin) therapy, FFP is used in conjunction with vitamin K, but plasma-derived, virus-inactivated prothrombin complex concentrate (PCC) is more effective for this indication, as described later.
For congenital factor deficiencies, plasma-derived or recombinant factor concentrates are often available and are preferable to plasma transfusion in terms of safety and efficacy ( Table 44.4 ). Plasma can be used as a replacement fluid (plasma exchange) in patients undergoing therapeutic plasma exchange (apheresis). It is the first-line therapy to reduce mortality from thrombotic thrombocytopenia purpura (TTP) by providing metalloprotease (ADAMTS13) that cleaves high-molecular von Willebrand factor (vWF). Plasma exchange has also been used to remove antibodies relating to ABO-incompatible or donor-specific HLA antibodies in pretransplant patients and heparin-induced thrombocytopenia before cardiac surgery. Large amounts of plasma are required to achieve sufficient antibody removal, and 5% albumin is used as an exchange fluid to reduce unnecessary donor exposures and the risk of allergic reaction.
FII IU/mL | FVII IU/mL | FIX IU/mL | FX IU/mL | PC IU/mL | PS IU/mL | Heparin U/mL | |
---|---|---|---|---|---|---|---|
4-Factor PCC | |||||||
Beriplex/Kcentra (CSL Behring, Germany) | 20–48 | 10–25 | 20–31 | 22–60 | 15–45 | 13–26 | 0.4–2 |
Octaplex (Octapharma, Austria) | 11–38 | 9–24 | 25 | 18–30 | 7–31 | 7–32 | <15 |
Prothromplex Total (Baxter, Austria) | 24–45 | 25 | 30 | 30 | >20 | NQ | <15 |
Cofact (Sanquin, Netherlands) | 14–35 | 7–20 | 25 | 11–39 | 11–39 | 1–8 | None |
3-Factor PCC | |||||||
Bebulin (Baxter, USA) | 30 | 3–5 | 25 | 35 | N.Q. | N.Q. | 3.75 |
Profilnine (Octapharma, Austria) | 37 | 3 | 25 | 16 | N.Q. | N.Q. | None |
Uman Complex DI (Kedrion, Italy) | 25 | N.Q. | 25 | 20 | N.Q. | N.Q. | N.Q |

Full access? Get Clinical Tree
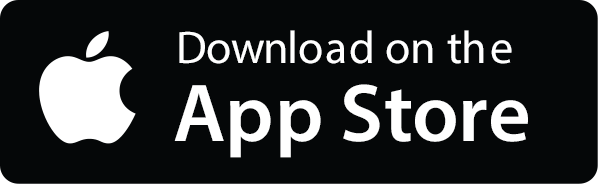
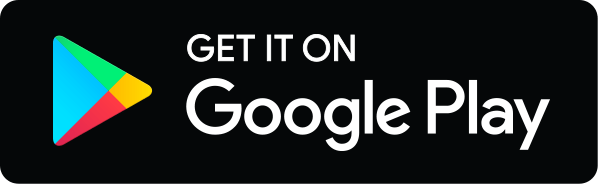
