Abstract
Each year, nearly 500,000 people in the United States seek treatment for burn injury, of whom 40,000 to 80,000 are hospitalized, and 3500 to 5000 die. Challenges in the initial care of patients with major burn injury include airway and ventilator management, vascular access, fluid resuscitation, and pain management. Major burns are further complicated by sepsis, frequently leading to multiorgan system failure (MOSF). The severity of burn injury is determined by the percentage of total body surface area (TBSA) involved; the depth of the burn; and the presence or absence of inhalational injury, acute lung injury, and respiratory failure. Aggressive early fluid resuscitation, nutrition, respiratory support, and serial burn wound excisional debridement and grafting are the mainstays of successful management of major burn injuries. The author presents a case of early management of 80% TBSA burn injury complications and discusses the pathophysiology, complications, and perioperative and critical care of major thermal injury.
Keywords
anesthesia of the burned patient, burn injury, complications of burn injury, inhalational injury, management of burn injury, thermal injury
Case Synopsis
You are an anesthesiologist on call, and a surgical resident covering the burn unit books an emergency extremity fasciotomy. The patient, a 40-year-old homeless man, was rescued from a burning garage and admitted to the burn unit the night before. He was found unresponsive at the scene, with a toxic blood ethanol level and deep burns covering about 80% of his total body surface area (TBSA). He is on ventilatory support in volume-control mode, with a fraction of inspired oxygen (Fi o 2 ) of 0.8, positive end-expiratory pressure (PEEP) of 15 cm H 2 O, and a tidal volume of 400 mL. As you prepare to transport the patient to the operating room (OR), you notice that his peak airway pressure is high. You are concerned about decreased chest compliance and your ability to effectively ventilate and oxygenate the patient during transport and in the OR. You share your concerns with the attending surgeon, who decides to perform chest and abdominal escharotomies.
Case Synopsis
You are an anesthesiologist on call, and a surgical resident covering the burn unit books an emergency extremity fasciotomy. The patient, a 40-year-old homeless man, was rescued from a burning garage and admitted to the burn unit the night before. He was found unresponsive at the scene, with a toxic blood ethanol level and deep burns covering about 80% of his total body surface area (TBSA). He is on ventilatory support in volume-control mode, with a fraction of inspired oxygen (Fi o 2 ) of 0.8, positive end-expiratory pressure (PEEP) of 15 cm H 2 O, and a tidal volume of 400 mL. As you prepare to transport the patient to the operating room (OR), you notice that his peak airway pressure is high. You are concerned about decreased chest compliance and your ability to effectively ventilate and oxygenate the patient during transport and in the OR. You share your concerns with the attending surgeon, who decides to perform chest and abdominal escharotomies.
Problem Analysis
Definition
Burn injuries are caused by heat, electric current, or caustic or corrosive chemicals. The severity of injury is determined by the extent and depth of tissue destruction. Thermal burns result from contact of the skin or mucous membrane and the underlying tissues with the heat source for the extent of time sufficient to produce injury. The severity of the thermal burn is directly proportional to the temperature of the heat source and the duration and surface area of the contact, and is inversely proportional to the thickness of the skin and the blood supply to the contact area. Chemical burns result from a chemical reaction of an offending agent with tissue components, dehydration, or corrosion. Electrical burns occur by arcing of electrical current across two points of body surface resulting in external injuries, or by conduction of heat along vessels and nerves to produce deep injuries. A secondary thermal burn may result from ignition of clothing by arcing of electrical current.
Recognition
The number of burn injuries occurring annually in the United States is estimated at between 1 million and 2 million. Each year, nearly 500,000 people seek treatment for burn injury, of whom 40,000 to 80,000 are hospitalized and 3500 to 5000 die. Most burned patients present in emergency departments of general hospitals without a dedicated burn center. Challenges in the initial care of patients with major burn injury include airway and ventilatory management, vascular access, fluid resuscitation, and pain management.
The severity of burn injury is determined by the percentage of total body surface area (TBSA) involved, depth of the burn, and the presence or absence of inhalational injury. TBSA burned is estimated using the “rule of nines” ( Fig. 78.1 ) or the Lund-Browder chart ( Fig. 78.2 ). The Lund-Browder chart is a diagram that accounts for age-specific changes in how the surface of various body parts contributes to TBSA, and thus it is better suited to estimate the size of burn injury in a child. The depth of a burn is defined as first, second, or third degree, based on whether there is superficial, partial-thickness, or full-thickness destruction of the skin.


First-degree burns (e.g., sunburn) are limited to the epidermis, appear as erythematous areas of otherwise intact skin, and may be painful, but heal without a specific intervention. Second-degree burns, or partial-thickness burns, partially involve the dermis, form blisters and moist, weeping lesions, blanch with pressure, and may be extremely painful. Second-degree burns require specific wound therapy including skin grafting. Third-degree or full-thickness burns involve the entire thickness of the dermis and may penetrate into the subcutaneous tissue.
The third-degree burn appears white, waxy, and is often speckled with red dots (i.e., heat-congealed hemoglobin). Sensory nerve endings are destroyed, so third-degree burns are insensate. Left untreated, third-degree burns heal by scarring that may result in severely disfiguring and crippling contractures; therefore third-degree burns should be excised and grafted early to preserve function. Inappropriate therapy, in particular, inadequate fluid resuscitation, may convert a second-degree burn injury to a full-thickness (third-degree) injury. Fourth degree is used to describe burns that involve deep structures, such as muscle, fascia, and bone.
Inhalation injury is present in 20% to 35% of hospitalized burn victims and is responsible for 60% to 70% of burn-related fatalities. It is important to recognize the inhalation injury as soon as possible. Inhalation injury should be suspected if the circumstances of the injury involve an exposure to fire and smoke in an enclosed space, especially in combination with loss of consciousness or intoxication. Findings on physical examination, including burns over the face, blisters on the lips and oral mucosa, singed nasal or facial hair, carbonaceous sputum, dysphagia, hoarseness, tachypnea, intercostal retraction, rales, and wheezing, point to the possibility of an inhalation injury. Early hypoxia may follow. Chest radiographs may be normal until the development of infection or atelectasis. Fiberoptic bronchoscopy may reveal carbonaceous debris, erythema, or ulcerations of the airway mucosa.
Risk Assessment and Implications
Burn Injury Pathophysiology
Major burns cause profound, generalized pathophysiologic changes. In the early postburn phase, tissue destruction at the site of the injury activates a cytokine-mediated systemic inflammatory response, characterized by increased capillary permeability and edema. When burn injury exceeds about 25% of TBSA, generalized edema develops. Loss of intravascular fluid due to generalized capillary leakage and weeping of fluids out of burned surfaces denuded of skin may quickly result in shock, with impaired tissue and organ perfusion. Burn shock occurs not only because of depletion of intravascular volume, but also because of increased systemic and pulmonary vascular resistance (due to release of catecholamines and vasopressin) and direct myocardial depression. Biochemical markers of the burn shock phase include hemoconcentration, lactic acidosis, and mixed venous desaturation. Shock leads to a decrease in glomerular filtration rate and stimulation of the renin–aldosterone–antidiuretic hormone axis, manifesting as acute oliguria. Hypothermia develops as a result of loss of thermoregulatory function of the dermis.
Within 48 to 72 hours after burn injury, the increased capillary leakage resolves, and edema formation significantly decreases. Fluid loss continues through open burn wounds. The shock phase is followed by the hypermetabolic phase, which starts a period of increased oxygen consumption, carbon dioxide production, increased protein catabolism, and insulin resistance. The hemodynamic picture during this phase is characterized by supranormal cardiac output, tachycardia, and low systemic vascular resistance. Patients with major burn injuries demonstrate increased requirements for most drugs, including sedatives, opioids, neuromuscular blocking agents, and antibiotics, owing to higher volume of distribution and increased clearance. The onset of sepsis, which may be extremely rapid and severe, aggravates the hyperdynamic state. Acute respiratory distress syndrome (ARDS) may develop.
Delayed consequences of burn injury include a persistent inflammatory state, immunosuppression, and severe protein catabolism, which continues until the closure of the burn wound. Burn wound infection is a leading cause of death in patients with burns exceeding 60% of TBSA. Early and repeated debridement of infected burn wounds is mandatory to maximize the patient’s chances of surviving the injury.
A full-thickness burn forms a rigid eschar over the burned area. Circumferential eschars of extremities may lead to compartment syndrome when edema forms under an eschar during an early phase of injury. Aggressive fluid resuscitation, essential in the management of the burn shock, may precipitate the development of the compartment syndrome. Unrecognized compartment syndrome may result in extensive muscle necrosis, rhabdomyolysis, secondary acute kidney injury, and the loss of affected extremities. Close monitoring of at-risk extremities and timely escharotomies are necessary to prevent secondary ischemic injury. Circumferential eschars of the trunk may result in a dramatic decrease in chest compliance and interfere with ventilatory mechanics. Such eschars also may require early escharotomies to enable effective ventilatory support.
Electrical injury often results in extensive, deep soft tissue damage that significantly increases resuscitative fluid requirements. Bone has the highest resistance to flow of electrical current, so the high heat produced by electrical current reaching the bone causes extensive damage of deep muscle surrounding the bone. Deep muscle injury may result in edema, compartment syndrome, muscle necrosis, and rhabdomyolysis. Myoglobinuria resulting from extensive muscle necrosis may lead to acute renal failure. Electrical shock often causes arrhythmia and myocardial damage similar to myocardial contusion.
Abdominal Compartment Syndrome
Massive fluid resuscitation in a setting of increased capillary permeability (sepsis, trauma, burn) results in retroperitoneal, mesenteric, and bowel edema and intraabdominal hypertension (IAH), manifesting as a progressive decrease in lung compliance, oliguria unresponsive to fluid resuscitation, hypotension, and acidosis. If untreated, it may be rapidly fatal by progressing to abdominal compartment syndrome (ACS) with acute renal failure, ventilatory compromise, bowel ischemia, and multiorgan system failure (MOSF). ACS may be prevented by maintaining a high suspicion index in the appropriate clinical setting (such as major burn injury), monitoring of intraabdominal pressure, and limiting the total volume of intravenous fluid resuscitation.
An increase in intraabdominal pressure above 20 mm Hg signals the onset of IAH. Management of IAH consists of deep sedation, permissive hypercapnia, chest and abdominal escharotomies, neuromuscular blockade, and percutaneous drainage of ascites. If conservative measures fail to prevent the progression of IAH, an emergency decompressive laparotomy may be necessary to prevent ACS.
Inhalation Injury
Inhalation injury occurs as a combination of (a) scalding of the face and upper airway by inhalation of steam and/or hot gases; (b) chemical injury to the trachea, bronchi, and pulmonary interstitium by the toxic components of the smoke; (c) impairment of oxygen transport processes by carbon monoxide poisoning; and (d) inhibition of oxygen utilization by inhalation of cyanide. Inhalation injury significantly contributes to morbidity and mortality related to burn injury. Scalding of the face and upper airway results in massive edema of the face, tongue, epiglottis, and vocal cords, and may rapidly lead to airway obstruction. Airway edema may be delayed by several hours. It may be precipitated by aggressive fluid resuscitation. A high index of suspicion and frequent assessment of respiratory function may help prevent a catastrophic airway obstruction.
Inhalation of smoke results in chemical injury of the tracheobronchial tree. Combustion products of the smoke from a house fire, such as hydrochloric acid, sulfuric acid, formaldehyde, acrolein, ammonia, and phosgene, are damaging to the trachea, bronchi, and alveoli. Paralysis of mucociliary transport impairs clearance of bacteria, mucus, and tissue debris. Atelectasis may rapidly occur because of loss of surfactant production and from plugging of small airways. ARDS may develop, especially if burn injury is complicated by MOSF. Mechanisms include inflammatory mediators from the burned area, effects of fluid resuscitation, and infection.
Carbon monoxide (CO) and cyanide present in inhaled smoke result in systemic toxicity. CO binds to oxygen-binding sites on hemoglobin with a 200-fold higher affinity. Binding of CO decreases oxygen-carrying capacity of hemoglobin and leads to tissue hypoxia. It also shifts the oxyhemoglobin dissociation curve to the left. Clinical symptoms of CO poisoning, including agitation, confusion, mental status depression, and coma, are nonspecific. A high index of suspicion helps with accurate diagnosis. A standard pulse oximeter does not distinguish carboxyhemoglobin from oxyhemoglobin. In a patient breathing supplemental oxygen, the Pa o 2 may be normal in the presence of CO poisoning. Diagnosis of CO poisoning is confirmed by measuring the level of carboxyhemoglobin in arterial blood, or by measuring oxygen-carrying capacity of hemoglobin by cooximetry. Carboxyhemoglobin levels greater than 15% are toxic. Levels above 40% result in severe neurologic dysfunction and coma. Levels exceeding 60% result in fatal cerebral hypoxic injury. The half-life of carboxyhemoglobin is 4 hours for a person breathing room air. Breathing 100% oxygen reduces the half-life of carboxyhemoglobin to 1 hour. Hyperbaric oxygen therapy has been suggested to reduce the neurologic sequelae of CO toxicity. However, no convincing benefit has been demonstrated for hyperbaric oxygen therapy in CO poisoning.
Cyanide is produced by the burning of nitrogenous materials. Cyanide poisoning should be suspected in a burned patient with anion gap metabolic acidosis, a high serum level of lactic acid, and increased mixed venous oxygen saturation in the presence of apparently adequate oxygen delivery. Cyanide blocks the final step in the oxidative phosphorylation by binding to mitochondrial cytochrome oxidase and preventing the use of oxygen for metabolism of pyruvate to adenosine triphosphate. Adenosine triphosphate can only be generated by anaerobic metabolism, which results in lactic acidosis. The increased mixed venous oxygen saturation suggests mitochondrial poisoning and the inability of the cells to utilize oxygen. Concentrations of cyanide greater than 20 ppm are considered toxic. Concentrations of 100 ppm can lead to seizures, coma, respiratory failure, and death.
Mortality related to burn injury has improved over the past decades. The rate of survival for major burns has increased because of higher awareness and advances in resuscitation; early excision and grafting of burn wounds; progress in methods of wound coverage, anesthesia, and critical care; early diagnosis and aggressive treatment of infections; and the recognition of the role of aggressive nutritional support, particularly early and uninterrupted enteral feeding. Available data support linking of improved outcomes for major burns with early referral to specialized burn units. Specialized expertise, personnel, and equipment required for effective care of patients with major burn injury are not available in low-volume centers and general hospitals. Mortality persists at a rate of approximately 4% for patients admitted to burn centers. A large analysis identified three risk factors predictive of death from burn injury: age older than 60 years, burn size greater than 40% TBSA, and inhalation injury. Mortality is a function of the number of risk factors present.

Full access? Get Clinical Tree
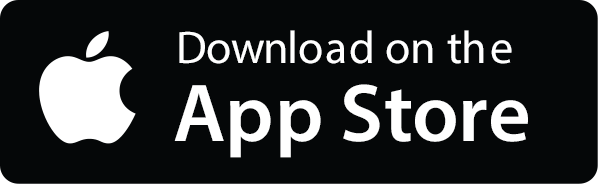
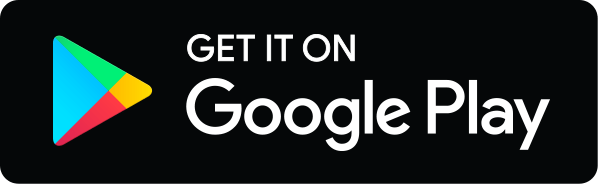