Chapter 35
Thermal Injury and Anesthesia
The American Burn Association’s National Burn Repository of 2011 estimates approximately 450,000 individuals a year with burn injuries visited emergency departments in the United States (between 2001 and 2009).1 Of these, approximately 45,000 individuals were hospitalized for acute burn injuries and a little more than half were admitted to one of 126 specialized burn centers around the country. These burn centers undergo a rigorous review by the American Burn Association (ABA) and the American College of Surgeons (ACS) to become verified. The verification process insures the facility provides high-quality, specialized care of burned patients, maintaining the necessary resources and medical services mandated by ABA guidelines.2
There has been a decline in the incidence of burn injury the past 2 decades. This is in part due to an increased focus on burn prevention including increased use of smoke detectors, fire and burn prevention education, decreased smoking, water temperature regulations, and regulation of consumer products and occupational safety. An improvement in all aspects of medical care of these patients has played a major role in improving survival. These include increased access to emergency medical care, advanced ventilation and treatment of inhalation injuries, improved infection control practices, enhanced nutritional support, early burn wound excision and grafting, and the treatment of the hypermetabolic response. The development of evidence-based practice guidelines and multidisciplinary care models available at regional burn care centers have also contributed to improvements.3
Nonetheless, during the period 2001 to 2010 in the United States, more than 1.25 million people sustained burn injuries annually with 450,000 receiving medical treatment.4 Approximately 3500 die from the burn injury—3000 from residential fires and 500 from motor vehicle or aircraft crashes.5 Approximately 75% of deaths occur at the scene of the accident or during initial transport.6 Roughly 35% of all burn victims are younger than 17 years of age, with more than 15,000 children requiring hospitalization as a result of their burn injuries. Scald injuries predominate among small children, with a progressive increase in the frequency of thermal-related burns in the elderly. Major causes of death in burn patients are multiple organ failure and infection. Major risk factors for death are older age and a higher total percentage of burned surface area, as well as chronic diseases. The main causes of early death (less than 48 hours after injury) are shock and inhalation injury. Multiorgan failure and sepsis were the most frequently reported causes of death. Death after a burn injury is not related to the toxic biologic effects of thermally injured skin but to shock associated with metabolic and infectious consequences of a large open wound, sepsis, inhalation injury, and extensive malnutrition, which cumulatively set the stage for life-threatening bacterial sepsis.7,8
Classification of Burn Injury
Burn injuries, regardless of their etiology, are classified according to the depth and extent of skin and tissue destruction, as well as the total body surface area (TBSA) involved. First-degree (superficial) burns are limited to the epidermis, the outermost layer of skin. The epidermis is thin and avascular, usually healing spontaneously and seldom requiring medical intervention. Second-degree burns, also known as deep and superficial partial-thickness burns, extend to the dermis. In contrast to the epidermis, the dermis is very vascular and contains numerous blood vessels and nerves. Thus severity of the type of burn varies, depending on the amount and depth of the dermal tissues involved. If the epithelial basement membrane of the dermis is intact, the skin will regenerate, and grafting may not be required. Third-degree burns, or full-thickness burns, extend to the subcutaneous tissue lying below the dermis. The entire skin thickness is destroyed with third-degree burns.9 Skin grafting is required for these types of burns because the epithelium and the dermal appendages are destroyed. A fourth-degree burn classification is used by some to describe structures burned below the dermis, such as muscle, fascia, and bone. Table 35-1 classifies burns according to depth of skin layers affected, and Figure 35-1 illustrates the layers of burn injury.
TABLE 35-1
Classification | Tissue Level Involvement | Outcome and Treatment |
First-degree burn (Superficial) | Epidermis | Skin appears red and slightly edematous; heals spontaneously; mild pain and no scarring; barrier preserved; sunburn or flash flame |
Second-degree burn (Partial-thickness) | ||
Superficial dermal burn | Epidermis and upper dermis | Heals spontaneously; red and edematous; appears wet, hyperemic, and blistering; heals in 7-10 days; scar uncommon |
Deep dermal burn | Epidermis and deep dermis | Requires excision and grafting for rapid return of function; heals in 2-8 weeks; probable scar without surgery |
Third-degree burn (Full-thickness) | Destruction of epidermis and dermis | Epidermal and complete dermal loss; wound excision and grafting required; limitation of function and scar formation; waxy white leathery appearance; no pain due to nerve damage |
Fourth-degree burn | Skeletal muscle, fascia, bone | Complete dermal loss with injury down to tendon or bone; complete excision, limited function; muscle necrosis; electrical injuries; limb loss common |
The burn team assesses the extent of a burn and plans initial resuscitation efforts. Burn wounds can be readily quantified, but estimation of the burn size remains subjective and assessor related. The most widely used estimation is the “rule of nines,” which was first described by Lund and Browder (Figure 35-2). The body is divided into regions that represent 9% or a multiple of 9% of the TBSA. Specific modifications apply to children because the surface areas of their heads and trunks are proportionally larger than their extremities.10 The rule of nines is a quick method to visually estimate burn size; however, it may not be all inclusive. The extent of burn injury can be more specifically quantified using the Lund and Browder chart. Figure 35-3 demonstrates this method, which is more accurate in determining a burn victim’s injury but is also more time-consuming to use. This method is often used when determining the extent of burn in the pediatric patient.
According to the ABA’s injury severity grading system, a major burn is (1) a second-degree burn involving more than 10% of the TBSA in adults or 20% at extremes of age, (2) a third-degree burn involving more than 10% of the TBSA in adults, (3) any electrical burn, or (4) a burn complicated by smoke inhalation. Associated mortality estimates follow a burn formula derived from the National Burn Registry: if the age of the patient plus percentage TBSA of burn exceeds 115, the mortality is greater than 80%. Additionally, clinical observations estimate the mortality of a burn victim is doubled if inhalation injury is sustained in conjunction with a thermal burn.
Etiologies of Burn Injuries
Thermal burns, or burns sustained from any heat source, commonly occur in and around the home. Approximately 70% of burns sustained by those up to the age of 4 years old are the result of scald injuries, whereas flame burns are the most common pattern among children 5 years and older. In general, younger children are at higher risk for sustaining burn injuries, and abuse or neglect may account for as much as 15% to 20% of these cases.3
Inhalation burn injuries often accompany thermal burns and should be suspected until aggressively ruled out.11–13 Inhalation injury can be classified into three types, based on anatomic location. The first type includes upper-airway injuries caused primarily by thermal injury to the mouth, oropharynx, and larynx. The second type includes lower airway and parenchymal injuries to the trachea, bronchioles, and alveoli caused by chemical and particulate constituents of smoke. Inhalation injury usually means injuries of this type. The third type includes metabolic asphyxiation in which certain smoke constituents such as carbon monoxide or hydrogen cyanide impair oxygen delivery or use by the tissues. All three types may coexist in a given patient.
Damage to the airway can vary, depending on whether the upper airway or lower airway is affected. Upper airway injuries result from inhalation of superheated air or steam and toxic compounds found in smoke.15 Brief exposure of the epiglottis or larynx to either dry air at 300° C or steam at 100° C can lead to massive edema and rapid airway obstruction.16 The addition of an inhalation injury to a cutaneous burn doubles the mortality rate17 and is a greater determinant of death than size of the burn wound. Inhalation of heated air can result in direct injury to the face, oropharynx, and upper trachea, while sparing the lower airway. It is speculated that heat entrained is readily dissipated in the upper airway, and there is reflex closure of the vocal cords effectively protecting the lower airway from heat-related injury. However, true thermal injury from exposure to live steam can occur in the lower respiratory tract because heat-exchange mechanisms of the airway are unable to cool the gas sufficiently as it is inhaled. Obstruction of the upper airway is due to excessive edema, macroglossia, and swelling of the pharyngeal soft tissue. Lower airway injuries more commonly arise from the inhalation of soot particles and/or chemicals produced by a fire. In the lower airway, inhaled toxins react with the airway mucosa forming acidic and alkali substances. Capillary permeability is increased. Extensive alveolar and epithelial damage can occur, with the trachea and bronchi becoming necrotic. Warning signs of respiratory injury include hoarseness, sore throat, dysphagia, hemoptysis, tachypnea, the use of accessory muscles, wheezing, carbonaceous sputum, and elevated carbon monoxide levels.14,18,19
Treatment of the burn patient involves three distinct phases: the resuscitative phase, debridement and grafting, and the reconstructive phase. Each phase has its own unique set of challenges the anesthesia provider must consider when developing an anesthetic plan.
Treatment of the Burn Patient
Airway Injury
It is essential to aggressively rule out upper airway injury in patients at risk (e.g., involving a fire that occurred in a closed space or the development of unconsciousness or stupor that prevented the patient from protecting his or her airway). Diagnosis is made by history, the circumstances surrounding the burn injury, and physical examination. Above the oropharynx, thermal injury can produce significant inflammation, occluding the airway. Heat rarely causes damage below the vocal cords because it is effectively dissipated. The other components of smoke such as particulate materials, systemic toxins, and respiratory irritants trigger a cascade of events, resulting in pulmonary edema and a ventilation/perfusion mismatch.20 Airway examination is best accomplished by direct visualization of the airway with a laryngoscope or fiberoptic bronchoscopy.21 Fiberoptic bronchoscopy is the gold standard for the diagnosis of the severity of inhalation injury. Table 35-2 has a commonly used classification of fiberoptic injury findings and associated mortality.22 The chest radiograph is usually normal in the early phase of inhalation injury (unless aspiration of gastric or pharyngeal contents occurred during the accident), becoming abnormal once pulmonary edema or infiltration develops. Treatment of upper airway injury involves early endotracheal intubation, even if the burn patient is not yet demonstrating signs of airway decompensation. Even in the absence of an inhalation injury, the lungs are at risk for compromise if the burn area is large.
TABLE 35-2
Grading Scheme for Fiberoptic Bronchoscopy Findings in Inhalation Injury
Adapted from Chou SH, et al. Fiber-optic bronchoscopic classification of inhalation injury: prediction of acute lung injury. Surg Endosc. 2004;18(9):1377-1379; Cancio LC. Airway management and smoke inhalation injury in the burn patient. Clin Plast Surg. 2009, 36(4):555-567.
Thermal damage to soft tissues of the respiratory tract and trachea can make intubation an incredibly difficult task because of the malalignment of structures, swelling, and bleeding of the tissues involved. Intubation of the trachea is much easier to perform earlier rather than later, when there may be glottic or facial edema, which worsens after fluid resuscitation.18–25 In the pediatric population, intubation should be performed with an uncuffed endotracheal tube, usually one size smaller than expected according to age and weight. Fiberoptic-assisted or laryngeal airway mask (LMA) guided intubation techniques are useful. The use of a retrograde wire, light wand, or GlideScope video-assisted techniques have all been used successfully.26 Nasotracheal intubation in children may be preferred because it is better tolerated, and tube displacement with movement is less likely. Cuffed endotracheal tubes are traditionally used for pediatric patients younger than 8 years old. They are commonly used in pediatric burns because of the added flexibility of not requiring replacement that can be necessitated by decreasing edema and air leaks, which is the case with uncuffed tubes.3,26
In the absence of an airway abnormality, early tracheal intubation can usually be achieved using a rapid-sequence technique with an intravenous (IV) induction agent and a rapidly acting muscle relaxant. There is general agreement that succinylcholine administration to patients more than 24 hours after burn injury is unsafe,27,28 although some authors extend this safe period to slightly longer.29 Receptor up-regulation occurs after a burn injury, with proliferation of acetylcholine receptors throughout the muscle membrane. Succinylcholine can cause potassium (K+) release from the entire muscle membrane rather than from discrete end-plate junctions, leading to hyperkalemia and possibly cardiac arrest.30 The magnitude of K+ elevation appears to be related to the size of the burn. This process of receptor up-regulation takes days to develop, allowing an initial 24-hour window of safety. It is wise to avoid the use of succinylcholine in a burn patient more than 24 hours after injury.31
Intubation with a nondepolarizing relaxant or without a relaxant via the techniques mentioned above allow for safe airway control. Several factors converge to produce an increased dose requirement of nondepolarizing relaxants in burn patients. These include up-regulation of acetylcholine receptors, massive fluid shifts producing significant changes in volume of distribution, and a qualitative decrease in receptor sensitivity. Plasma protein-binding alterations do not effect relaxants clinically because they are not highly bound. Rocuronium, for example, is only 30% protein bound. Both the dose administered and the serum concentrations required in the burn patient may be increased three- to five-fold to achieve the desired paralysis with a nondepolarizer.32–34
For the most part, after airway management, the burn patient is taken to the intensive care unit and placed on ventilatory support.35 Inspired gases should be humidified to aid clearing of tracheobronchial debris and prevent drying of secretions. The endotracheal tube must be kept in place until the surrounding laryngeal edema has subsided. A progressive air leak around the endotracheal tube, especially in uncuffed pediatric sizes, may be an indication that edematous tissue is returning to normal.14,18–20,36
Administration of nebulized heparin and N-acetylcysteine with massive burn and smoke inhalation injury results in a decreased incidence of re-intubation for progressive pulmonary failure, decreased atelectasis, and reduced mortality. The Shriners Burns Hospital formula includes 5000 units of heparin and 3 mL of a 20% solution of N-acetylcysteine aerosolized every 4 hours for the first 7 days after the injury.20
Carbon Monoxide Poisoning
Any burn victim rescued from an enclosed-space fire should be considered at high risk for carbon monoxide poisoning. It is estimated that 50% to 60% of all fire victims die from carbon monoxide poisoning.37 Symptoms depend on the carboxyhemoglobin level, although it is actually the tissue carbon monoxide level that determines the toxicity of carbon monoxide (Table 35-3).
TABLE 35-3
Carboxyhemoglobin (%) | Symptoms |
0-10 | Normal |
10-20 | Headache, confusion |
20-40 | Disorientation, fatigue, nausea, visual changes |
40-60 | Hallucination, combativeness, convulsion, coma, shock state |
60-70 | Coma, convulsions, weak respiration and pulse |
70-80 | Decreasing respiration and stopping |
80-90 | Death in less than 1 hour |
90-100 | Death within a few minutes |
From Herndon DN. Total Burn Care. 4th ed. Edinburgh: Saunders; 2012:411.
Carbon monoxide binds to the hemoglobin molecule with 200 times greater affinity than oxygen, leading to a fall in oxyhemoglobin saturation as tissues become unable to extract oxygen.14 The end result is a disruption in mitochondrial oxidative phosphorylation, which produces metabolic acidosis at the cellular level. Analysis of blood gases reveals a normal arterial oxygen tension but a decreased total oxygen content, indicating that the hemoglobin oxygen saturation is markedly reduced. Carbon monoxide increases the stability of the oxyhemoglobin molecule, decreasing the release of oxygen to the tissues and producing a leftward shift in the oxyhemoglobin curve (see Figure 17-6). Pulse oximeters do not detect carboxyhemoglobin (CoHgb) in blood and give falsely elevated readings for oxygen saturation in its presence. The diagnosis of carbon monoxide poisoning requires measurement of arterial CoHgb levels using a co-oximeter. The half-life of CoHgb is variable. In patients treated with 100% oxygen, it ranged from 26 to 148 minutes.38 Treatment continues with 100% oxygen until the CoHgb level is less than 5% or for 6 hours.39 A co-oximeter measures concentrations of all hemoglobin moieties (i.e., oxyhemoglobin, deoxyhemoglobin, carboxyhemoglobin, and methemoglobin).40
Hyperbaric oxygen therapy (HBOT) is an alternative treatment and may be helpful if the CoHgb level exceeds 25% or if clinical signs of toxicity are evident. Hyperbaric oxygen accelerates the clearance of carbon monoxide beyond that achieved using 100% oxygen at 1 atmosphere. The main rationale for its use is prevention of delayed neurocognitive syndrome. It can be difficult to transport and care for patients in the chamber, and debate continues over its effectiveness.41
Cyanide Poisoning
Hydroxocobalamin has a rapid onset of action, neutralizes cyanide without interfering with cellular oxygen use, and has a good safety profile. It is safe for use with smoke-inhalation victims, easy to administer, and is the preferred antidote to this type of cyanide poisoning. A hydroxocobalamin dose of 50 mg/kg is recommended. In addition, aggressive stabilization of cardiopulmonary function augments the hepatic clearance of cyanide via rhodanase, and can resolve cyanide poisoning (blood levels, 5.6-9 mg/L), without the use of antidotes.14,20,42
Hypovolemic Shock Associated with Thermal Injury
After the airway has been secured and other life-threatening injuries have been managed, the burn patient must be resuscitated with large volumes of fluid. Aggressive fluid administration and restoration of blood volume are critical for improving chances of patient survival and preventing renal failure. Burns cause a form of hypovolemic shock. These changes are reflected in loss of circulating plasma volume, hemoconcentration, massive edema formation, decreased urine output, and depressed cardiovascular function.25,43 Fluid losses are greatest in the first 12 hours after burn injury and then begin to stabilize after 24 hours. Fluid losses occur secondary to direct transudation of plasma and plasma proteins from the wound and from diffuse capillary leakage that shift fluid from the intravascular space to the interstitium of unburned tissue. Capillary leak results from a loss of endothelial integrity and from reduction of intravascular oncotic pressure as plasma proteins are lost through the burn wound and incompetent capillary beds. The end result of changes in microvasculature caused by thermal injury is disruption of normal capillary barriers separating intravascular and interstitial compartments and rapid equilibration of these compartments. This causes severe depletion of plasma volume and a marked increase in extracellular fluid, clinically manifested as hypovolemia and burn-induced edema.10,25,43
Inflammatory mediators are released from burned tissues after injury, causing localized inflammation and burn-wound edema. Localized mediators include oxygen radicals, arachidonic acid metabolites, histamine, prostaglandins, leukotrienes, products of platelet activation, and the complement cascade.23,37,44 Most edema occurs locally at the burn site and is maximal at 24 hours after injury. The edema itself results in tissue hypoxia and increased tissue pressure with circumferential injuries. Aggressive fluid therapy can correct the hypovolemia but will accentuate the edema process. In minor burns, the inflammatory process remains sequestered in the wound itself. However, in major burn insults, this local injury signals the release of systemic circulatory mediators resulting in a systemic response.
Fluid Resuscitation
Within seconds after an acute burn injury, massive fluid shifts begin to occur. Therefore, fluid resuscitation and airway management are the hallmarks of initial therapy and should be instituted by the first-response emergency medical providers. There are many formulas for calculating a burn patient’s initial fluid resuscitation requirements. Crystalloid resuscitation often provides substantial volumes of fluid, often in excess of that predicted by current formulas, resulting in numerous edema-related complications. This phenomenon is coined “fluid creep,” and fluid therapy after the initial 24 hours is constantly being refined. In most centers, two formulas are accepted as guidelines for the resuscitation of severely burned patients, the Parkland and modified Brooke formulas.45,46 The need for blood transfusion is usually not a major concern during the immediate resuscitation phase in acutely burned patients unless other coexisting trauma exists. Transfusion management is a prominent issue during subsequent surgical intervention.
The current ABA consensus formula for fluid resuscitation and urine output in burn patients is given in Box 35-1. Table 35-4

Full access? Get Clinical Tree
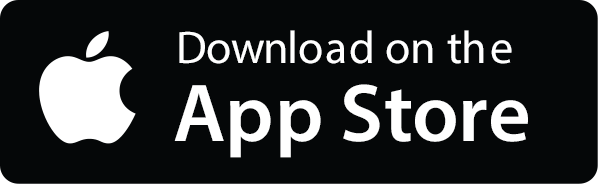
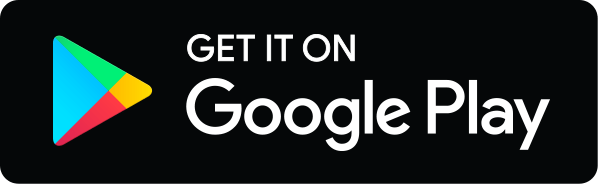