KEY POINTS
Induced hypothermia has been shown to reduce mortality when applied after resuscitation from cardiac arrest.
Current guidelines recommend induced hypothermia for out-of-hospital cardiac arrest (OHCA) shockable rhythms and suggest consideration of induced hypothermia for OHCA nonshockable rhythms and in-hospital patients.
In unconscious adults after out-of-hospital cardiac arrest, mild hypothermia (36°C) appears to be as effective as more extreme hypothermia (33°C) with regard to survival and neurological function.
Induced hypothermia may have benefit for other disease processes such as myocardial infarction and stroke.
The mechanisms by which hypothermia acts are multifaceted and a focus of much current investigation.
The notion of cooling patients for medical benefit is quite old. In 1814, Baron Larrey, a French surgeon in the service of Napoleon’s army, reflected on soldiers who suffered major injuries on the frozen battlefields in Russia by commenting that “cold acts on the living parts … the parts may remain … in a state of asphyxia without losing their life.”1 A belated resurgence of interest in hypothermia has taken place in the past decade, expanding the possible medical indications for its use. Induced hypothermia, the intentional lowering of body temperature, has been explored in a number of acute critical care settings, including myocardial infarction, stroke, head trauma, and after cardiac arrest. While the optimal depth and timing of hypothermia are not yet established for these uses, most experts advocate a temperature goal of 32°C to 34°C because it seems to provide significant benefit while avoiding most of the adverse effects associated with the intervention. Timing of hypothermia, with respect to both time of induction and duration of therapy, is even more uncertain, although general consensus holds that cooling should be initiated as soon as possible after the morbid event and should be maintained for at least 12 to 24 hours. Regarding specific uses, there is particularly good evidence that hypothermia is protective for the resuscitated cardiac arrest patient after return of spontaneous circulation (ROSC).2,3 The use of hypothermia in other clinical scenarios remains promising but less clear at present.
This chapter addresses elements of the history of hypothermia, the laboratory and clinical data that have developed our understanding of its use, some of the various techniques used to cool patients, and the clinical syndromes for which hypothermia appears to offer the greatest advantage.
HISTORY OF INDUCED HYPOTHERMIA
The protective effects of hypothermia induction have been suggested since the time of Hippocrates, who advocated packing bleeding patients in snow.4 Hypothermic protection was also noted by Napoleon’s battlefield surgeon, Baron Larrey, during the French invasion of Russia. He observed improved survival of injured soldiers left in the snow compared with those treated with warm blankets and heated drinks.1 Induced hypothermia has been studied in a wide variety of illnesses, both ischemic and nonischemic in nature (reviewed in refs. 5 through 7). These include traumatic brain injury,8-10 status epilepticus,11 arrhythmia, sepsis, and the ischemic illnesses of myocardial infarction, stroke, and cardiac arrest.7,12 Interestingly, the first reported use of induced hypothermia was in the setting of malignancy. In 1939, Fay and colleagues treated patients with metastatic carcinoma, with the goal of both pain reduction and retardation of tumor growth.13 While hypothermia to 32°C for 24 hours did not prove effective for the stated goals, it was considered well tolerated.13
A decade later, Wilfred Bigelow studied the induction of hypothermia in the setting of cardiac surgery, with the goal of cerebral protection.14 Two other studies using hypothermia as therapy for cardiac arrest were also published. Both these early cardiac arrest studies used moderate hypothermia of 30°C to 34°C in patients after resuscitation from cardiac arrest. One of these pioneering papers presented a series of four patients, all of whom were cooled and survived arrest.15 In the other study, 12 patients were cooled with a survival rate of 50% compared with 14% survival in 7 normothermic control patients.16
During the 1960s and 1970s, the field of induced hypothermia lay relatively dormant for reasons that remain unclear. Some have suggested that more dramatic therapies were developed that overshadowed cooling as a possible therapy, such as controlled ventilation, monitored ICU management, and cardiopulmonary resuscitation (CPR).5 Additionally, several adverse effects of hypothermia were described, which may have dampened enthusiasm.17,18
Interest in “resuscitative hypothermia” was rekindled by Peter Safar and others at the University of Pittsburgh, who demonstrated in a ventricular fibrillation dog model of cardiac arrest that mild to moderate hypothermia could be induced to improve outcomes.19,20 Trauma research also provided a motivation for the development of induced hypothermia. It was understood from military combat experience that definitive therapy for penetrating trauma was often delayed for practical reasons (eg, transportation and access to surgical care) and that measures were needed to preserve exsanguinating soldiers until appropriate care could be delivered.21 Given the animal data on exsanguination and cooling, it appeared that hypothermia might be a suitable approach.22
Safar went on further to describe “suspended animation,” a process that allows “rapid preservation of viability of the organisms in temporarily unresuscitable cardiac arrest, which allows time for transport and repair during clinical death and is followed by delayed resuscitation, hopefully to survival without brain damage.”12 Hypothermia has been a primary component of this concept of stasis. In this paradigm, victims of cardiac arrest may be cooled to some target temperature and maintained at that temperature for a specific period of time. With advanced medical interventions, which may include cardiopulmonary bypass, metabolic correction, and controlled reperfusion, the patient is stabilized and rewarmed, and “reanimation” is initiated. While many methodologies have been studied under the rubric of suspended animation, including cardiopulmonary bypass and pharmacologic interventions,23 these are used most often as adjuncts to the use of hypothermia.
Since these initial observations in the 1980s and early 1990s, much of the work pertaining to hypothermia and ischemic disease has focused on focal ischemia and reperfusion, for example, animal stroke and myocardial infarction models. A number of ischemia-reperfusion (IR) model systems have been developed over the last two decades, including cellular,24 isolated organ,25 and whole-animal models in which arterial supply to the organ under study is temporarily occluded.26,27 In this latter category are included experiences with human IR, for example, during coronary vascular procedures.28 More recently, two seminal papers were published describing the use of hypothermia to successfully treat resuscitated cardiac arrest patients.2,3 With these studies, hypothermia has moved from the laboratory to active clinical use.
MECHANISMS OF HYPOTHERMIC PROTECTION
The mechanisms by which induced hypothermia protects against cellular and tissue injury are poorly understood. Given the importance of temperature in a wide range of physiologic processes, it is reasonable to conclude that multiple mechanisms may be involved in any given tissue (reviewed in refs. 5 and 6). Some mechanisms implicated in hypothermic protection include modulation of transcription and/or translation, suppression of reactive oxygen species (ROS) production, and inhibition of programmed cell death, or apoptosis. The mechanisms by which cooling protects tissues may overlap as well. For example, the effect of hypothermia on cellular metabolism may lead indirectly to modulation of programmed cell death, and cooling may have a direct effect on cell death machinery itself (see Fig. 26-1). Most of the data pertaining to mechanism of hypothermic protection comes from studies of IR injury in models of stroke and myocardial infarction.
A number of gross physiologic changes have been observed in the setting of hypothermia that may contribute to decreased injury. As early as 1954 it was noted that hypothermia induced by ice water immersion could lower cerebral oxygen consumption in dogs by approximately 7% per 1°C drop in temperature.29 Other studies have demonstrated that mild hypothermia in rats improves postischemic cerebral blood flow disturbances.30 Another marker of general physiologic injury after reperfusion, brain edema, was also found to be reduced by hypothermia in a rat model of global ischemia.30,31 Finally, hypothermia has been shown to minimize damage to the blood-brain barrier, which in turn may protect against blood-borne toxic metabolites reaching brain tissues through the compromised barrier.30,32
Intracellular signaling can alter the array of gene transcription activity of a cell quickly and dramatically, and this, in turn, can trigger a variety of injury processes. In a cardiac arrest mouse model, a group of signaling pathway genes known as the immediate early genes was activated after resuscitation.33 A study of liver IR demonstrated a drop in c-jun terminal kinase activity at 25°C when compared with normothermic controls.34 An extracellular signaling molecule thought to protect against injury, BDNF, was increased in a rat model of cardiac arrest when animals were cooled to 33°C.35
A number of biochemical changes during IR can be modified by the induction of hypothermia. In a gerbil stroke model, animals subjected to mild hypothermia were found to have decreased arachidonic acid metabolism compared with normothermic controls.36 In a rat brain ischemia model, hypothermia to 32°C reduced nitric oxide production, as measured in jugular blood.37 Whether these attenuations are simply markers of hypothermic effects or actually relevant factors in reperfusion injury remains to be clearly established. Other biochemical phenomena seem more likely to be linked directly to damage processes, such as the observation that hypothermia slows ATP depletion during IR.38 ROS production also appears to be attenuated by hypothermic conditions in a rat cerebral ischemia model.39
Programmed cell death is a complex yet ubiquitous process by which cells actively chose or are chosen to die. This cellular program can be activated as part of normal physiology, such as during embryonic development, or as an abnormal response in a wide variety of disease states.40,41 Much evidence implicates the induction of apoptosis as a component of reperfusion injury.42,43 A recent report showed that the apoptotic pathway enzyme caspase 3 was upregulated in brain tissue after resuscitation from cardiac arrest, as measured at autopsy in patients who died within days of undergoing resuscitative measures.44 Widespread evidence from animals also supports the notion that apoptosis is activated after reperfusion.25,45 Hypothermia may inhibit this process. Proteolysis of the cytoskeletal protein fodrin, a characteristic step in the apoptotic pathway, is inhibited by hypothermia to 32°C in a rat brain IR model.46 The process of apoptosis is an active one, requiring protein synthesis and enzymatic activity, both of which may be inhibited by lower temperatures. While some data suggest that the degree of apoptosis can be reduced by hypothermia, the topic certainly deserves more investigation in animal models.
HYPOTHERMIA IN CARDIAC ARREST
Cardiac arrest is a highly mortal condition that leads to at least 300,000 deaths each year in the United States alone.47 Survival from cardiac arrest remains dismal some 50 years after introduction of chest compressions and electrical defibrillation, with only 1% to 11% of patients surviving until hospital discharge after out-of-hospital cardiac arrest.48-50 While initial survival from in-hospital cardiac arrest ranges from 25% to over 50%, subsequent survival until hospital discharge is much lower, from 5% to 22%, suggesting that a high mortality rate is seen shortly after initial ROSC. Some of this mortality after return of normal circulation may be due to events related to reperfusion injury (see Fig. 26-2). For further discussion of cardiac arrest and resuscitation therapies, see Chap. 25.
Cardiac arrest remains a major medical challenge despite research efforts over the past few decades. There is little time after arrest to defibrillate the heart and thereby stop ongoing ischemic injury to key organs such as the heart and brain. Few therapies are proven to be useful during the postresuscitation phase of cardiac arrest—when up to 90% of patients go on to die despite successful defibrillation. New approaches are desperately needed to improve cardiac arrest survival, and induced hypothermia may be one of the most promising new approaches.51
Hypothermia may be helpful during three periods of time in a cardiac arrest (Fig. 26-3): (1) prearrest, (2) intraarrest, and (3) postarrest. Prearrest cooling can only be used practically as a preoperative intervention when the heart is stopped in a controlled fashion during cardiac surgery. Postarrest cooling to 32°C to 34°C was found to be protective in some human cardiac arrest trials, induced by applying cooling blankets or ice packing in the subset of cardiac arrest patients having ROSC who remained unresponsive.2,3 The goal of this level of hypothermia was to prevent neurologic injury and decrease mortality while maintaining a temperature warm enough to prevent the other adverse effects of more profound cooling (eg, cardiac arrhythmia, coagulopathy, and infection—see discussion of adverse effects later in this chapter). This cooling was protective despite taking 4 to 8 hours to reach target temperature after ROSC (Fig. 26-4). Intraarrest cooling, when cooling is induced after failed initial CPR, has the potential to induce a protective state long enough for more definitive circulation (eg, cardiac bypass) to be established and life restored. Little is known about the optimal depth or clinical potential of such hypothermia owing in large part to the technical difficulties involved in inducing hypothermia during the low-flow states of sudden cardiac arrest.
FIGURE 26-4
Data from the Hypothermia After Cardiac Arrest (HACA) study, in which hypothermia was induced via circulated-air cooling blankets. Temperatures shown are taken via bladder monitoring. Mortality differences between the hypothermia patient group and normothermic groups are shown at right. (Adapted with permission from the Hypothermia After Cardia Arrest Study Group. Mild therapeutic hypothermia to improve the neurologic outcome after cardiac arrest. N Engl J Med. February 21, 2002;346(8):549-556.)
The 2010 Advanced Cardiac Life Support (ACLS) guidelines strongly recommend cooling out-of-hospital (OOH) ventricular fibrillation or pulseless ventricular tachycardic postarrest patients who remain comatose. The guidelines for in-hospital and OOH nonshockable rhythm arrests encourage providers to consider this therapy for these patients.52 See Table 26-1 for sample exclusion criteria. It is recommended that ICU physicians and staff establish protocols for cooling after cardiac arrest. Development of such protocols will require consideration of a number of hospital-specific technical issues (eg, how to cool, who will do the cooling, how to monitor temperature).
Post-Cardiac Arrest Therapeutic Hypothermia Exclusion Criteria
|
Cooling in these patients should be achieved as rapidly as possible using internal catheter devices, external cooling blankets, or ice. When ice is used, cloth or other material should be placed between the skin and the ice to avoid frostbite. Temperature should be measured via bladder probe, esophageal probe, pulmonary artery catheter, or tympanic probe if invasive temperature monitoring is not available. Temperature should be taken every 15 to 30 minutes during the cooling protocol and until a stable cooled temperature state is achieved.
There is some debate regarding whether a temperature of 36°C or 33°C is optimal. A large multicenter trial in 2013 showed no difference in survival or neurological recovery in out-of-hospital cardiac arrest patients cooled to 33°C, compared to those kept at 36°C.53
Current literature suggests that patients should be cooled for approximately 24 hours at a temperature between 32°C and 34°C (with the 24-hour interval starting at the achievement of goal temperature, not the initiation of the cooling process). Avoidance of temperatures below 32°C is important to minimize potential adverse effects from reduced body temperature. Cooling may induce shivering and could result in patient discomfort if sedation is not adequately established. In the published studies of hypothermia after cardiac arrest, patients uniformly received neuromuscular blockade and sedation, and it is likely most patients will require such pharmacologic treatment. The disadvantages of this management strategy is that neurologic examination is not interpretable, and paralytic agents carry the risk of long-term neuromyopathy, though post-cardiac arrest patients undergoing hypothermia will generally only be paralyzed for approximately 36 to 48 hours. Special attention should be placed on monitoring these patients for electrical evidence of seizures, as seizures are common in the post-cardiac arrest population (occurring in as many as 20%-25% of postarrest patients).
Patients should be rewarmed slowly at a rate of 0.25°C to 0.5°C per hour. Rewarming often requires active intervention, which can include warmed intravenous fluids and warming blankets. Rebound pyrexia is common in this population for up to 48 to 72 hours after rewarming54

Full access? Get Clinical Tree
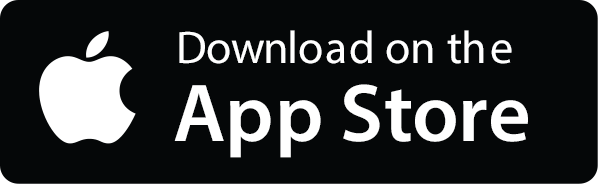
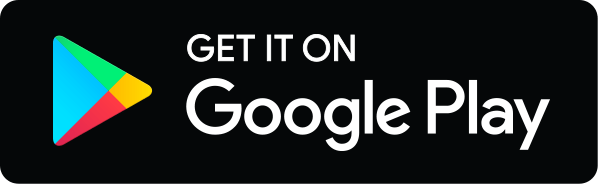
