Chapter 35 The aim of this chapter is to arm the acute care surgeon/surgical critical care specialist who rarely cares for children with a fundamental understanding of the key differences between adult and pediatric surgical critical care, so such care can be provided in the absence of a pediatric surgical critical care specialist. The most common condition for which this specialist will be consulted is major pediatric trauma. Less common conditions include respiratory and circulatory failure, neonatal surgical emergencies and postoperative surgical emergencies. Thus, while emphasizing the care of the major trauma patient, the chapter will focus on important differences between neonates, children, and adults with respect to: 1. Bedside skills necessary to determine when a pediatric patient is critically ill. 2. Respiratory structure and function, and how they may affect ventilatory management. 3. Circulatory structure and function, and how they may affect hemodynamic support. 4. Basic neurologic assessment and determination of brain death. 5. Basic fluid, electrolyte, and nutritional management, including renal replacement therapy. 6. Critical management of respiratory and circulatory failure, as well as common life-threatening pediatric surgical conditions such as major pediatric trauma, neonatal surgical emergencies, postoperative surgical emergencies, and the use and misuse of analgesic and sedative agents. It is important to note that pediatric critical care is provided in the multidisciplinary environment of a pediatric intensive care unit (PICU) or neonatal intensive care unit (NICU) where specialists in pediatric critical care medicine or neonatology are typically available to collaborate in the management of critically ill and injured children. In such an environment, the acute care surgeon/surgical critical care specialist will be welcomed as a collaborating partner with special expertise in the intensive care of patients with surgical conditions who, instead of acting independently, will work as a member of a team of critical care specialists. Such collaboration fits well with the patient- and family-centered approach to critical care applied in the PICU and NICU.1 However, to optimally prepare for the moment when such collaboration is requested, the acute care surgeon/surgical critical care specialist who rarely cares for critically ill and injured children is strongly advised to participate in educational offerings such as the Pediatric Advanced Life Support Course of the American Heart Association and American Academy of Pediatrics, the Pediatric Fundamental Critical Care Support Course of the Society of Critical Care Medicine, and the standing course in Current Concepts in Pediatric Critical Care offered by the same organization at its annual meeting, and to take advantage of their associated textbooks as well as resources such as the Handbook of Pediatric Surgical Critical Care of the American Pediatric Surgical Association recently made accessible in the public domain as the February 2015 number of Seminars in Pediatric Surgery. The goals of pediatric and adult surgical critical care are fundamentally the same; in both instances, the aims of therapy are to support vital functions until the disease process responsible for their deterioration is treated or resolved. However, the spectrum of illness confronting specialists in pediatric surgical critical care is somewhat different from that confronting their adult counterparts; this is because a major proportion of the problems confronting the specialist in pediatric surgical critical care are diseases of immaturity or prematurity which can be expected to improve with the passage of time, in contrast to the degenerative diseases which are chiefly responsible for critical illness in the adult surgical patient. Even so, while there are a number of acquired diseases unique to the pediatric population which will also confront the specialist in pediatric surgical critical care, e.g., hemolytic-uremic syndrome (HUS) in the child, bronchopulmonary dysplasia (BPD) in the infant, there are far more which are not unique to childhood, e.g., major trauma, severe sepsis/septic shock, and multiple organ system failure (MOSF), including acute respiratory distress syndrome (ARDS). Fortunately, since the recuperative powers of the child are great, partly because most vital organs have not fully completed their growth, there is much to be gained by heroic effort: If the intervention is successful, the child may lead a normal life; yet, there is also a downside to such an aggressive approach: Precisely because of their remarkable recuperative powers, children who are saved but do not recover fully may be forced to live with severe disabilities. While the differences between infants (particularly neonates), children, and adults may seem overwhelming to the surgical critical care specialist who cares for children infrequently, it is important to remember that there are far more similarities than there are differences. Doses of fluids and drugs must, of course, be calculated carefully on the basis of body mass, using either body weight or body surface area as a first approximation, but the effects of such interventions are generally quite similar. However, due to problems of size and scale, these doses must be determined precisely, for even small errors may have large effects when the doses are small to begin with. Use of a length-based, color-coded resuscitation tape (Broselow Pediatric Emergency Tape) or a hybrid age- and length-based, hand-aided memory aid (Handtevy Pediatric Resuscitation System) for estimation of drug dosages will minimize the chances for such error in emergencies, but it is now recognized that the tape-based and hand-based systems may underestimate drug dosages in morbidly obese children by as much as 20%, so reliance on measurement of body mass together with wall charts or published formularies, and a portable calculator, is preferred. Nevertheless, while the “ABCs” may be the same for children and adults, marked differences in anatomy can result in sharp differences in physiology. For example, due to the small size of the infant’s heart, cardiac output is more dependent upon heart rate than on stroke volume, while the decreased compliance of an infant’s lungs previously mandated the use of a pressure-controlled rather than a volume-controlled ventilator, to minimize the complications of barotrauma and volutrauma (although this practice may be changing as newer ventilators have become available). Even so, whether the patient is a child or an adult, optimal perfusion is achieved when both heart rate and filling pressures are appropriate for the patient’s size and condition, while adequate ventilation requires that an adequate volume of gas be exchanged even if supranormal pressures are required to achieve this. It is therefore not surprising that most treatment strategies used by specialists in pediatric surgical critical care are borrowed from their adult counterparts, although they may be adjusted to meet the particular anatomic and physiologic needs of the child. Yet, the most important difference between infants, children, and adults is also perhaps the most obvious one: They are smaller. It is therefore crucial that physicians for caring critically ill children have at their disposal an array of cannulas, catheters, and tubes of many different sizes (which must be carefully secured to prevent dislodgement by the child’s wandering hands), as well as different types and sizes of ventilators (with the smallest amount of tubing possible to minimize dead space), to allow proper monitoring as well as treatment to be performed. Of necessity, most such tools represent scaled-down versions of adult technology. Unfortunately, this sometimes leads to the erroneous conclusion that the child is nothing more than a small adult: For better, or for worse, this is most definitely not the case. While the late signs of physiologic distress in the neonate and infant are similar to those observed in older children and adults, the early signs may be subtle or absent. For example, the fever, lethargy, and irritability so characteristic of sepsis in the older infant and child are rarely, if ever, present in the neonate, especially if premature; instead, intolerance of feedings, clusters of apnea and bradycardia, episodes of oxygen desaturation, hypotonia, hypothermia, and hyperglycemia (or in particularly severe cases, hypoglycemia, the first indication of which, especially in the premature infant, may be a seizure), will be the earliest presenting signs. Unfortunately, ominous changes in vital signs, such as hypotension, may not occur until much later. Thus, even the slightest suspicion of sepsis, a major killer in both the PICU and the NICU, warrants an aggressive search for a source, and prophylactic treatment with antibiotics appropriate to the local epidemiology of bacterial organisms, until culture results become available. In contrast to the neonate, the clinical signs of physiologic distress in the infant are usually not so subtle. However, they are still not very specific, and, early on, may be apparent only to the child’s parents or nurse, who often may not be able to pinpoint exactly what is amiss, but know that something is wrong. Nevertheless, level of activity, particularly social activity, feeding behavior, degree of comfort, and skin color are useful parameters to follow. Thus, children who are irritable, lethargic, have lost interest in their surroundings, do not interact with their parents, do not react to invasive procedures, who feed poorly, who assume unnatural positions, or who are pale or mottled, are usually in some sort of trouble; careful physical examination will typically lead to recognition of the precise causes of their distress. One useful tool for rapid identification of the critically ill or injured child is the Pediatric Assessment Triangle, or PAT (Fig. 1).2 This tool provides adult oriented clinicians with a simple method to suspect that an infant or child is ill, seriously ill, or critically ill, based on rapid visual assessment at the bedside of Appearance, work of Breathing, and Circulation to skin: If one limb of the PAT is abnormal, the infant or child is ill; if two limbs, seriously ill; if three limbs, critically ill. Moreover, the limb of the PAT that seems most deranged usually indicates which vital system is the source of the problem: respiratory, circulatory, or metabolic/neurologic; primary assessment of the “ABCDEs” is then applied to confirm the clinician’s initial impression and guide initial resuscitation. Of note, the utility and accuracy of the PAT has been recently validated for use in triage by pediatric emergency nurses as well as paramedics. Measurement of vital signs is also a key step in determining an infant’s or child’s level of distress. However, it must be remembered that anxiety or stimulation can markedly affect vital signs; it is therefore important that the rapid “primary assessment” referred to above be completed before vital signs are actually measured. The ranges of normal for vital signs vary with age and size (Table 1). Again, it must be remembered that “normal” vital signs are not always appropriate for a specific patient, and that the blood pressure measurement, in particular, will not be accurate unless obtained with the proper-sized cuff, i.e., one which is two-thirds as wide as the distance from the axilla to the antecubital fossa, or in the lower extremity of infants and small children, from the groin to the knee, or the knee to the ankle. Fig. 1. The pediatric assessment triangle. (Adapted from Horeczko T, Enriquez B, McGrath NE, Gausche-Hill M, Lewis RJ. The Pediatric Assessment Triangle: Accuracy of its application by nurses in the triage of children. J Emerg Nurs. 2013; 39:182–189.) The tongue of the infant is larger than that of the adult, while the jaw is smaller; for this reason, and because of the need to breathe while nursing at the breast, the infant remains an obligate nasal breather until approximately six months of age. Moreover, the funnel-shaped larynx of the infant is more superior and anterior, while the trachea is shorter, narrower, and more membranous than that of the adult; as a result, the infant airway is both more susceptible to episodes of obstruction due to congenital causes such as laryngomalacia and tracheomalacia, and glottic and subglottic webs, and acquired causes such as stenosis and edema, and more difficult to manage. Oropharyngeal airways, as in the adult, are useful adjuncts in airway maintenance, perhaps especially so in infants, due to the higher frequency of upper airway obstruction by the soft tissues themselves, although care must be taken both to ensure that it is properly inserted (it must be inserted right-side up, not upside-down then rotated, to avoid injury to the soft palate) and that the proper size is used (its flange should lie against the incisive foramen while its tip should rest adjacent to the angle of the mandible when placed against the side of the face); nasopharyngeal airways, however, are better tolerated, can improve extrathoracic airway obstruction due to hypotonic pharyngeal musculature or edematous soft tissues, and may facilitate posterior pharyngeal suctioning, but they, too, must be sized correctly (the diameter should not exceed that of the naris, while the flange should lie upon the naris as its tip rests adjacent to the tragus). Indications for placement of an endotracheal tube, of course, are no different in infants and children than in adults; however, it is important to remember that the short length of the trachea in the infant (approximately 5 cm) and the small child (approximately 7 cm) makes positioning and fixation of the tube extremely crucial, while the narrow diameter of the tube predisposes to obstruction, both by kinking, and inspissated mucoid or secretions, thus requiring aggressive pulmonary toilet to avoid this potentially lethal complication. Table 1. Normal vital signs in pediatric patients Normal respiratory rates by age.
The Pediatric Surgical ICU Patient
Arthur Cooper, Pamela Feuer, Logeswary Rajagopalan,
Ranjith Kamity and Mary Joan Marron-Corwin
Overview
Introduction
Bedside Assessment
Airway, Lungs, and Ventilation
Age | Breaths/min |
Infant (<1 year) | 30–60 |
Toddler (1 to 3 years) | 24–40 |
Preschooler (4 to 5 years) | 22–34 |
School age (6 to 12 years) | 18–30 |
Adolescent (13 to 18 years) | 12–16 |
Normal heart rates (per minute) by age.
Normal blood pressures in children by age.
(Adapted from Pediatric Subcommittee and Special Contributors, Emergency Cardiovascular Care Committee, American Heart Association, Chameides L, Samson RA, Schexnayder SM, Hazinski MF, eds. Pediatric Advanced Life Support Provider Manual, Professional Edition. Dallas, TX, American Heart Association, 2011.)
There are also significant differences in the anatomy of the chest which have important effects on the mechanics of breathing. The increased pliability of the chest wall, which results from the cartilaginous nature of the infant skeleton and the rudimentary development of the intercostal muscles, results in a relatively elastic thoracic bellows which is exquisitely sensitive to the extremes of positive and negative intrathoracic pressure and positive intra-abdominal pressure, while the more horizontal alignment of the ribs increases the overall contribution of the diaphragm to the movement of gas; the net effect of these anatomic peculiarities, when the infant is nursed supine, is that the diaphragm tends to pull the costal margins inward, rather than pull itself downward, as is the case in a more upright position. For this reason, infants who are breathing spontaneously should be nursed in a semi-erect position whenever this is feasible; moreover, because the infant is chiefly a diaphragmatic breather, anything that compromises diaphragmatic excursion, e.g., major abdominal surgery, diaphragmatic paralysis, gastric distention, or increased abdominal pressure of any other cause, will significantly affect alveolar ventilation. The presence of breath sounds, unfortunately, does not guarantee that air entry is adequate, for they are easily transmitted through the thin chest wall of the child, even if the lung directly beneath the chestpiece of the stethoscope is atelectatic; this problem may be compounded if a pediatric- or adult-sized stethoscope is used instead of an infant-sized model, but may be avoided to some extent by listening for breath sounds just below the axillae, and by always checking to be sure that breath sounds are bilaterally symmetric.
The lungs themselves are not fully mature at the time of birth; while branching is largely complete, alveoli continue to increase in number until well into childhood years. The lungs of the neonate are therefore far less compliant than those of older children and adults; unfortunately, the decreased compliance of the lungs may result in the generation of extremely high transthoracic pressures. This can lead, especially in the presence of edema and/or obstruction of the terminal airways, to “shearing” injuries which can result, in turn, in the development of barotraumatic complications such as pneumothorax, pneumomediastinum, pneumopericardium, and/or the air block syndrome, which, in its severe form, may also result in the development of pulmonary interstitial emphysema (PIE), and so-called “acquired lobar emphysema” (ALE). These problems are obviously compounded when positive pressure is required to maintain effective ventilation; of the two, high mean airway pressure, which is chiefly a function of peak inspiratory pressure, appears to be a more important etiologic factor than high end expiratory pressure. The signs of respiratory failure in the infant, of whatever cause, are tachypnea, flaring, retracting, and grunting, and in its terminal stages, cyanosis and apnea, followed if uncorrected by hypoxia, bradycardia, and death. If due to acute obstruction, severe rocking chest motions may be apparent, indicative of the infant’s violent struggle for life, although neurologic or peripheral muscle disease causing hypotonia may preclude this increased work of breathing. Infants will, however, often without apparent effort or distress, tolerate much greater levels of chronic hypoxia and/or hypercarbia than older children or adults. Finally, the presenting signs of ARDS, which occurs in infants and children as it does in adults, either independently following acute lung injury (ALI) or infection, or in tandem with MOSF resulting from sepsis or trauma, are similar to those observed in the adult, although the disease may progress somewhat more rapidly; as in the adult, ARDS is defined using criteria established at the 2012 Berlin Consensus Conference: Respiratory failure not explained by cardiac failure or fluid overload occurring within one week of a known clinical insult or new respiratory symptoms; characterized by bilateral opacities on chest imaging not explained by effusions, lobar or lung collapse or nodules; and manifested by an oxygen deficit, categorized by the PaO2/FiO2 as mild (200–300), moderate (100–200), or severe (<100).
Critical Care of Life Threatening Respiratory Compromise
Indications for tracheal intubation and mechanical ventilation (hypoxemic or hypercarbic respiratory failure that cannot be successfully treated by less invasive means), and criteria for weaning (resolution of the disease process, improving pulmonary function, progressive withdrawal of support), are similar in children and adults (Table 2); the techniques are equally straightforward. Enough airway pressure must be generated to assure adequate gas insufflation, as judged not only by auscultation, but also by direct observation of the chest wall such that it is seen just to begin to rise; enough tidal volume must be generated to assure adequate minute ventilation, so as to avoid both alveolar hypoventilation and hyperventilation, recognizing that in infants, small airways close at higher lung volumes than they do in children (a process that may be mitigated by surfactant preparations in preterm infants, for rescue in those <34 weeks gestational age, for prophylaxis in those <27 weeks gestational age), such that higher than usual inflating pressures may be required to maintain adequate lung expansion. In large infants and children, synchronized intermittent mandatory ventilation (SIMV) combined with pressure support (PS) has been most frequently used in recent years; however, pressure-regulated, volume-controlled (PRVC) ventilation is increasingly commonly employed, particularly for long term mechanical ventilation, either in controlled ventilation (CV) or SIMV mode, so as to minimize the development of ALI and forestall its progression to ARDS by limiting both barotrauma and volutrauma. By contrast, while recent studies indicate that PRVC ventilation may also achieve excellent results even in neonates and small infants, due to the availability of state-of-the-art ventilators that are designed to facilitate accurate delivery even of very small breaths to these tiny patients, time-cycled, pressure-limited (TCPL) ventilation in SIMV mode combined with PS is still most often used; ventilatory strategies that seek to limit both airway pressure and tidal volume are most likely to forestall the progression to chronic lung disease, chiefly BPD.
Table 2. Indications for mechanical ventilatory support in pediatric patients.
Ventilation abnormalities | Respiratory muscle dysfunction |
1. Respiratory muscle fatigue | |
2. Chest wall abnormalities | |
3. Neuromuscular disease | |
4. Decreased ventilatory drive | |
5. Increased airway resistance and/or obstruction | |
Oxygenation abnormalities | 1. Refractory hypoxemia |
2. Need for positive end expiratory pressure | |
3. Excessive work of breathing |
(Adapted from Pediatric Fundamental Critical Care Support Planning Committee, Society of Critical Care Medicine, Madden MA, ed. Pediatric Fundamental Critical Care Support, 2 ed. Mount Prospect, IL: Society of Critical Care Medicine, 2013.)
The goal in all patients is to achieve tidal volumes that are very slightly above normal (6–8 mL/kg) so as to compensate for the mismatch in ventilation and perfusion which results from the redirection of blood flow to the posterior aspects of the lung (by gravity) and air flow to the anterior aspects of the lung (by abnormal diaphragmatic motion) when the patient is nursed supine; higher volumes (10–12 mL/kg) may be needed in patients with neuromuscular disease, lower volumes (4–6 mL/kg) in patients with ARDS. Frequency (f) and inspiratory time (TI) are matched to the patient’s size, but may be increased or decreased as necessary to optimize alveolar ventilation; inspiratory:expiratory (I:E) ratios (normally 1:2), adjusted by increasing or decreasing TI above or below normal values for children (0.8–1 sec) and neonates (0.3–0.5 sec) may need to be higher (1:1) in patients with difficulty oxygenating such as ARDS, lower (1:3–1:4) in patients with outflow obstruction such as asthma or BPD. The fraction of inspired oxygen (FiO2) and positive end expiratory pressure (PEEP), starting respectively at 1.0 and 5 cm H2O, should be quickly adjusted to achieve oxygen saturations (SPO2) of 94–99%, i.e., oxygen tensions (PaO2) of 90 –100 Torr, in the child, first by lowering the former; oxygen tensions in the newborn infant should be no more than the normal 70–80 Torr, as higher levels may predispose to the development of retinopathy of prematurity (ROP), chronic lung injury (BPD), and MOSF, especially in the premature infant. Use of inspired oxygen fractions greater than 0.4–0.6, peak inspiratory pressures (PIPs) that exceed 25–30 cm H2O, and end expiratory pressures more than 8–10 cm H2O, is discouraged, but may occasionally be required to achieve adequate oxygen saturation, recognizing that airway pressure release ventilation (APRV) or high frequency oscillatory ventilation (HFOV) should be considered to achieve adequate alveolar ventilation and oxygenation and minimize barotrauma and volutrauma whenever PIPs approach 25 cm H2O; in select circumstances, however, it may be preferable to accept slightly lower than ideal oxygen saturations and slightly higher than ideal carbon dioxide concentrations (permissive hypercapnia) to avoid development and perpetuation of ALI, and ultimately its progression to ARDS. Obviously, whether using a volume-controlled or pressure-controlled ventilator, attempts should be made to use the minimal settings needed to obtain the desired results; weaning should be accomplished as soon as feasible, most often via SIMV, and concluding with a spontaneous breathing trial (SBT), the likely failure of which is best predicted by a rapid shallow breathing index (RSBI) of >105 breaths/min/L.
Non-invasive positive pressure ventilation (NPPV) methods are increasingly being used in the pediatric population; bilevel positive airway pressure (BiPAP) ventilation may avoid the need for intubation in cooperative older children with mild to moderate acute respiratory failure due to asthma, neuromuscular conditions, obstructive sleep apnea, and end stage cystic fibrosis, among others. In neonates, both term and preterm, use of continuous positive airway pressure (CPAP), both at bedside and during transport, most commonly in the former via an underwater seal apparatus (using an acetic acid solution to retard the growth of Pseudomonas aeruginosa and reduce the risk of ventilator-associated infections) to generate end expiratory pressure (“bubble” CPAP), using specially-designed, large-bore, low-flow nasal prongs (Hudson RCI cannula, Teleflex, Wayne, PA), less commonly in the latter via a hand-held, T-piece resuscitator (NeoPuff, Fisher & Paykel Healthcare, Irvine, CA; Neo-Tee, Mercury Medical, Clearwater, FL), has revolutionized the management of mild to moderate forms of respiratory distress syndrome (RDS) in this patient population, as bubbling is known to have an oscillatory effect that promotes alveolarization, hence lung development; in selected cases in which bubble CPAP (or its alternative, ventilator driven CPAP, which is still being utilized in many NICUs) is insufficient to support oxygenation upon weaning from mechanical ventilation, nasal intermittent mandatory ventilation (NIMV) in a TCPL mode, using a different specially-designed nasal cannula (NeoTech RAM cannula, Valencia, CA), may be considered. Advanced modes of ventilatory assistance, such as neurally-adjusted ventilatory assistance (NAVA), in which a transesophageal detector device recognizes and transmits electromyographic evidence of diaphragmatic motion to a specially augmented ventilator, allowing earlier initiation, hence improved synchrony, of a mechanically delivered breath, are being explored, but as yet are insufficiently studied for routine use; detailed discussions of HFOV and inhaled nitric oxide (iNO) use are beyond the scope of this chapter, as patients requiring such support should be treated in tertiary, ideally extracorporeal membrane oxygenation (ECMO) capable, pediatric or neonatal centers, but are most often considered for severe (persistent or worsening) hypoxemic respiratory failure (PaO2 <60 Torr on FiO2 >0.6), such as intractable ARDS in the child, or the “pulmonary injury sequence (PIS) of prematurity” in the preterm infant (RDS which progresses to PIE or pulmonary air leak syndrome), persistent pulmonary hypertension (PPHN), and lung hypoplasia deemed compatible with life. Finally, while a detailed discussion of ECMO itself is also beyond the scope of this chapter, it too is increasingly being employed with encouraging results in selected cases of severe, intractable (unresponsive to HFOV and iNO) acute respiratory failure; consensus criteria for initiation of ECMO in neonates (most often for PPHN associated with congenital diaphragmatic hernia) are (1) gestational age >34 week, (2) weight >2.2 kg, (3) no lethal or major cardiac malformation, (4) no significant coagulopathy or uncontrollable bleeding, (5) no major intracranial hemorrhage, (6) potentially reversible lung injury, (7) duration of mechanical ventilation <10–14 da, and (8) severe, intractable respiratory failure manifested by an oxygenation index (OI) > 25 (which corresponds to a mortality rate of 50%), and in children (most often for ARDS), although still in evolution, respiratory failure despite optimal therapy as manifested by (1) a PaO2 <60 Torr on FiO2 1.0 or an alveolar-arterial oxygen difference (A-aDO2) >500 Torr, (2) persistent respiratory acidosis (pH <7.25), (3) plateau pressure (Pplat) >30 cm H2O, and (4) a physiologic shunt fraction > 30%.
Heart, Vessels, and Circulation
While the actual cardiac outputs of the infant and child differ significantly from those of the adult, their cardiac indices are roughly the same. However, both because the neonatal myocardium is less compliant, and because the relatively small myocardial mass precludes significant increases in stroke volume with volume loading, heart rate is far and away the most important determinant of cardiac output. Thus, with the exception of the rare supraventricular or ventricular tachycardia so rapid that it precludes adequate ventricular filling, bradycardia, of which the most common cause in the infant or child is hypoxia, is the most immediate threat to the integrity of the circulation. Unfortunately, the immaturity of the autonomic nervous system in the neonate results in exquisite sensitivity to vagal stimulation such as tracheal suctioning or gastric dilatation, dampening the effectiveness of the tachycardia response to hypoxia.
The immaturity of the autonomic nervous system is also such that incomplete myocardial innervation may make it more difficult for the neonate to respond appropriately to situations which demand sustained increases in cardiac output. Fortunately, the neonate does respond well to exogenously administered catecholamines, in doses which are generally similar, per kilogram, to those used in adults. The neonate also seems to respond better to afterload reduction than the adult. However, the reverse is also true: Increases in systemic or pulmonary vascular resistance may result in significant myocardial dysfunction.
The signs of circulatory failure in the infant, fortunately, are fairly similar to those seen in the adult. Even so, the remarkable ability of the child to vasoconstrict peripheral, and in end stages, splanchnic vascular beds is such that mean arterial pressure and core organ perfusion, but not peripheral or splanchnic organ perfusion, are preserved until late in the downward spiral, thus predisposing to earlier development of metabolic acidosis, with its deleterious effects upon myocardial function. Biventricular failure is also more common in infants and small children than in adults. However, while signs of left heart failure are usually obvious, classic signs of right heart failure, such as jugular venous distention, hepatojugular reflux, peripheral edema, or ascites, are usually absent; indeed, tiring during feeds, forehead diaphoresis, and hepatomegaly may be the only physical signs of right heart failure in infants or small children.
In part for this reason, and because the more easily obtained hemodynamic variables, e.g., pulse, blood pressure, and urine output, are often not specific enough to allow therapy to be targeted at reversal of the particular mechanism which is deranged, there is continuous emphasis upon the selective use of invasive monitoring techniques. The child who is in cardiogenic, septic, or neurogenic shock may therefore benefit from central venous pressure monitoring; so does the child in hemorrhagic or hypovolemic shock who does not respond appropriately to volume resuscitation. In highly select circumstances, measurement of pulmonary artery occlusion pressure may also be obtained; however, as placement of flow-directed balloon catheters, i.e., Swan–Ganz catheters, in infants and small children carries a greater degree of risk that in adults, it behooves the critical care specialist to use this device only when necessary, and then to obtain as much information as possible from the intervention. Models equipped with thermistors for determination of cardiac output are preferred, as measurement of this variable using the thermal dilution technique is probably the least operator-dependent of the available methods, provided reasonable precautions are taken to assure the saline tracer is maintained at the correct temperature (0°C) until the moment of infusion; with this device, one can generate an entire hemodynamic profile, i.e., oxygen consumption, systemic and pulmonary vascular resistance, by measuring mixed venous oxygen tension and saturation simultaneously with arterial values, cardiac output, and the blood hemoglobin concentration. Development of less invasive methods of cardiac output monitoring in infants, children, and adults continues to be explored; pulse contour-based, Doppler-based, and lithium dilution-based techniques have all been applied in the clinical arena, and while all have been tested in adults, results have been inconsistent to date, and little if any data are yet available in pediatric populations; fortunately, bedside echocardiography can be a highly useful tool for the evaluation of cardiac anatomy, myocardial contractility, signs of pulmonary hypertension, and/or pericardial effusion or cardiac tamponade.
Critical Care of Life Threatening Circulatory Compromise
As always, proper management of circulatory failure, of whatever cause, depends upon an understanding of the basic pathophysiology as well as careful interpretation of available data; hypovolemic shock, and to a lesser extent septic shock, are far and away the most common forms of shock encountered in the pediatric patient, and as in adults, both are treated initially with volume resuscitation. Cardiogenic shock, common in pediatric cardiac surgical patients, is uncommon in pediatric general surgical patients; regardless, by using the hemodynamic profile as a guide to therapy, it is possible to “optimize” filling pressures, cardiac work, and systemic vascular resistance in such a way as to achieve the most reasonable cardiac index for the lowest possible oxygen consumption, hence the least additional stress for an already overburdened system. Fortunately, normal values are similar for adults and children; in general, one should attempt to keep oxygen consumption at approximately 160 mL/m2, as this value has been associated with the greatest survival. Regardless of the type of shock that may be encountered, however, its prevention by rapid recognition and treatment of the underlying cause remains the most effective therapy; it is therefore vital that the critical care specialist never forget that the presentation of circulatory failure in the child is deceptive, as the physical signs may not become manifest until late in the course of the disease, by which time shock may be irreversible, unless proper treatment has been instituted.
The circulating blood volume of the preterm infant is approximately 90–105 mL/kg, of the term infant approximately 80–85 mL/kg, and of the child approximately 70 mL/kg. While these values represent a larger proportion of circulating blood volume than in the adult, in absolute numbers these volumes are quite small; volume resuscitation should therefore proceed in small but rapidly infused aliquots (“boluses” or “pushes”) of no more than 20 mL/kg (to avoid the development of acute fluid overload), repeated as necessary to restore tissue perfusion, supported in septic and neurogenic shock (once central venous pressure has been normalized) by a vasopressor agent (norepinephrine in the child, dopamine in the infant) if the patient remains hypotensive. Of course, given the relatively smaller absolute blood volume of the child, every attempt should be made to minimize the amount of blood which is drawn for laboratory tests, while the volume of each specimen obtained should be accurately recorded. Finally, because of the high concentration of hemoglobin F, which unloads oxygen suboptimally under extrauterine conditions, it is usually necessary to maintain the hematocrit of the neonate above 40%.
The treatment of severe sepsis and septic shock in pediatric patients has undergone profound change in recent years; in the pediatric surgical patient, these conditions will most likely be encountered in patients with uncontrolled intrathoracic or intra-abdominal infection from an incompletely drained empyema or complicated perforative appendicitis associated with generalized peritonitis and multiple interloop and subdiaphragmatic abscesses for which source control, if possible, is the key element of treatment. Even so, the international Surviving Sepsis Campaign, sponsored in part by the Society of Critical Care Medicine, has resulted in substantial reductions in mortality from severe sepsis and septic shock; the case fatality rate for these conditions, which once approximated 50%, has been halved in most centers, due to the introduction of what has been termed “early goal-directed therapy” (EGDT), consisting of early and aggressive fluid administration, timely antibiotic therapy, administration of a vasopressor agent for refractory shock, and reliance on diagnostic tests and prognostic indicators including blood cultures and serum lactate measurements. While the adult algorithm calls for intravenous fluids, blood cultures, and serum lactate within three hours of emergency department (ED) triage, and additional fluids, pressor agents (if needed), and repeat lactate within six hours, for adult patients with severe sepsis or septic shock, the pediatric algorithm mandates that intravenous fluids and intravenous antibiotics be given within one hour of ED triage for pediatric patients with these conditions; of note, the definitions of severe sepsis and septic shock are age-specific (Table 3).3 The recent publication of two prospective, randomized adult trials of EGDT (ProCESS and ARISE) has dampened enthusiasm for these algorithms somewhat4,5; however, it is important to recognize that the enrollment criteria for both of these trials incorporated certain key elements of EGDT, while their “standard therapy” arms were provided by adult intensivists in academic centers, suggesting that EGDT was being provided informally rather than by protocol, leading the Society of Critical Care Medicine to reaffirm the Surviving Sepsis Guidelines.6

Full access? Get Clinical Tree
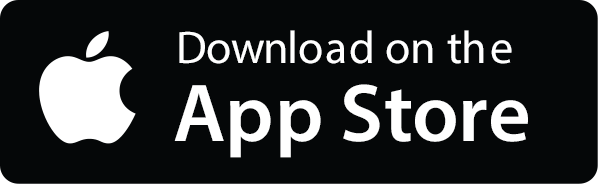
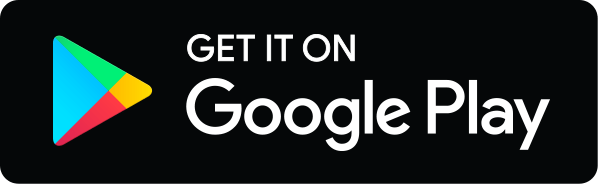