FIG. 10.1 Left lateral view of the brain, showing the principal divisions of the brain and four major lobes of the cerebrum.
Motor Areas
No single area of motor control exists within the brain because the integration and control of muscle activity depends on the harmonious activities of several areas, including the cerebral cortex, the basal ganglia, and the cerebellum.
Primary Motor Area
The primary motor area of the cerebral cortex is located in the precentral gyrus of the frontal lobe, just in front of the central sulcus, and is concerned mainly with the voluntary initiation of finely controlled movements such as those of the hands, fingers, lips, tongue, and vocal cords. The amount of area in the primary motor cortex devoted to a particular muscle or muscle group is a reflection of the degree of fine motor control required for the proper functioning of these muscles. For example, the muscles that control speech or the use of the fingers are represented by many more neurons within the primary motor cortex than are the larger muscles of the legs or trunk. This disproportionate representation within the primary motor cortex is a reflection of the relative importance the brain places on the proper control of different muscles.
Axons from the primary motor cortex descend through the internal capsule, midbrain, and pons to the medulla. These axons are called pyramidal because of the shape of the structure they form with the medulla. Within the medulla, most of these axons decussate and continue down into the spinal cord via the lateral corticospinal tracts. Fibers that do not decussate in the medulla descend down the spinal cord via the ventral corticospinal tracts. Most of these fibers eventually decussate at lower levels within the cord. Pyramidal cell axons also connect within the brain with the basal ganglia, the brainstem, and the cerebellum. Generally, these pyramidal motor nerves constitute a direct pathway from the primary motor area to the muscles and are concerned mostly with control of discrete, detailed body movements.
Premotor Area
The premotor area of each hemisphere is located in the cortex immediately in front of the primary motor cortex in the frontal lobe. On the whole, this area is concerned with movement of the opposite side of the body, especially with control and coordination of skilled movements of a complex nature such as throwing or kicking a ball. In addition to its subcortical connections with the primary motor area, its neurons also have direct connections with the basal ganglia and related nuclei in the brainstem—for example, the reticular formation. Many of the axons from these subcortical centers cross to the opposite side before descending as extrapyramidal tracts in the spinal cord. Collectively, the connections from the premotor area to these related nuclei compose the extrapyramidal system, which coordinates gross skeletal muscle activities that are largely automatic and repetitive in nature. Examples are postural adjustments, chewing, swallowing, gesticulating during speech, and associated movements that accompany voluntary activities. Certain portions of the extrapyramidal tract also have an inhibitory effect on spontaneous movements initiated by the cerebral cortex and serve to prevent tremors and rigidity. Complete structural and functional separation of the pyramidal and extrapyramidal systems is impossible because they are so closely connected in the harmonious work of executing complex coordinated movements.
Of interest to the PACU nurse is that drugs used during the perioperative period can cause extrapyramidal reactions eliciting stereotypical neurologic reactions. More specifically, the neuroleptics such as the phenothiazines (of which chlorpromazine is the prototypal drug), the butyrophenones as typified by droperidol (Inapsine) and haloperidol (Haldol), and the antiemetic metoclopramide (Reglan) are known to produce extrapyramidal reactions. The following four types of extrapyramidal reactions exist: drug-induced parkinsonism, akathisia, acute dystonic reactions, and tardive dyskinesia.
Drug-induced parkinsonism, which can occur 1 to 5 days after the administration of the neuroleptic drug, is typified by a generalized slowing of automatic and spontaneous movements (bradykinesia) with a masklike facial expression and a reduction in arm movements. The most noticeable signs of drug-induced parkinsonism syndrome are rigidity and oscillatory tremor at rest. The treatment is an antiparkinsonian agent such as levodopa, trihexyphenidyl, and benztropine.
Akathisia, which can occur immediately or up to 60 days after the administration of a neuroleptic drug, refers to a subjective feeling of restlessness accompanied by a need on the part of the patient to move about and pace back and forth, acute anxiety, and a feeling of impending doom. Treatment requires a reduction in the dosage of the responsible drug and the administration of a benzodiazepine if encountered during the perioperative period.
Acute dystonic reactions may occur after the administration of some psychotropic drugs and are characterized by torsion spasms such as facial grimacing and torticollis. These reactions are occasionally seen when a phenothiazine is first administered, and they are associated with oculogyric crises (involuntary eye movements). Acute dystonic reactions may be mistaken for hysterical reactions or seizures and can usually be reversed with anticholinergic antiparkinsonian drugs such as benztropine or trihexyphenidyl.
Tardive dyskinesia is a late-appearing neurologic syndrome characterized by stereotypic, involuntary, rapid, and rhythmically repetitive movements such as continual chewing movements and darting movements of the tongue. Treatment is not always satisfactory because antiparkinsonian drugs sometimes exacerbate tardive dyskinesia. Tardive dyskinesia often persists despite discontinuation of the responsible drug.2,3
Two important structural aspects of the premotor area are worth noting for those who care for neurosurgical patients. First, the fibers from both the primary motor and the premotor areas are funneled through the narrow internal capsule as they descend to lower areas of the CNS. This action is significant because the internal capsule is a common site of cerebrovascular accidents that can result in a variety of motor deficits. Second, lesions within one side of the internal capsule result in paralysis of the skeletal muscles on the opposite side of the body because of the crossing of fibers within the medulla.4
Motor Speech Area
This area is only one point in the complicated network needed to form spoken and written words. The motor speech area lies at the base of the motor area and slightly in front of it in the inferior frontal gyrus and is also known as the Broca area. In right-handed people, the language and speech areas are usually located in the left hemisphere. In those who are left-handed, these areas may lie within the right or the left hemisphere.
Prefrontal Area
This area of the frontal lobe lies anterior to the premotor area, has extensive connections with other cortical areas, and is believed to have an important role in complex intellectual activities such as mathematic and philosophic reasoning; abstract and creative thinking; learning; judgment and volition; and social, moral, and ethical values. The prefrontal area also influences certain autonomic functions of the body with the conduction of impulses directly or indirectly through the thalamus to the hypothalamus, which makes possible certain physiologic responses to feelings such as anger, fear, and lust.
Sensory Areas
Sensory information from one side of the body is received by the somatic sensory area of the opposite hemisphere, which is located in the parietal lobe in the area of the postcentral gyrus. Crude sensations of pain, temperature, and touch can be experienced at the level of the thalamus, but true localization and discrimination of these sensations are functions of the parietal cortex. The activities of the somatic sensory area allow for proprioception; for the recognition of the size, shape, and texture of objects; and for the comparison of stimuli as to intensity and location.
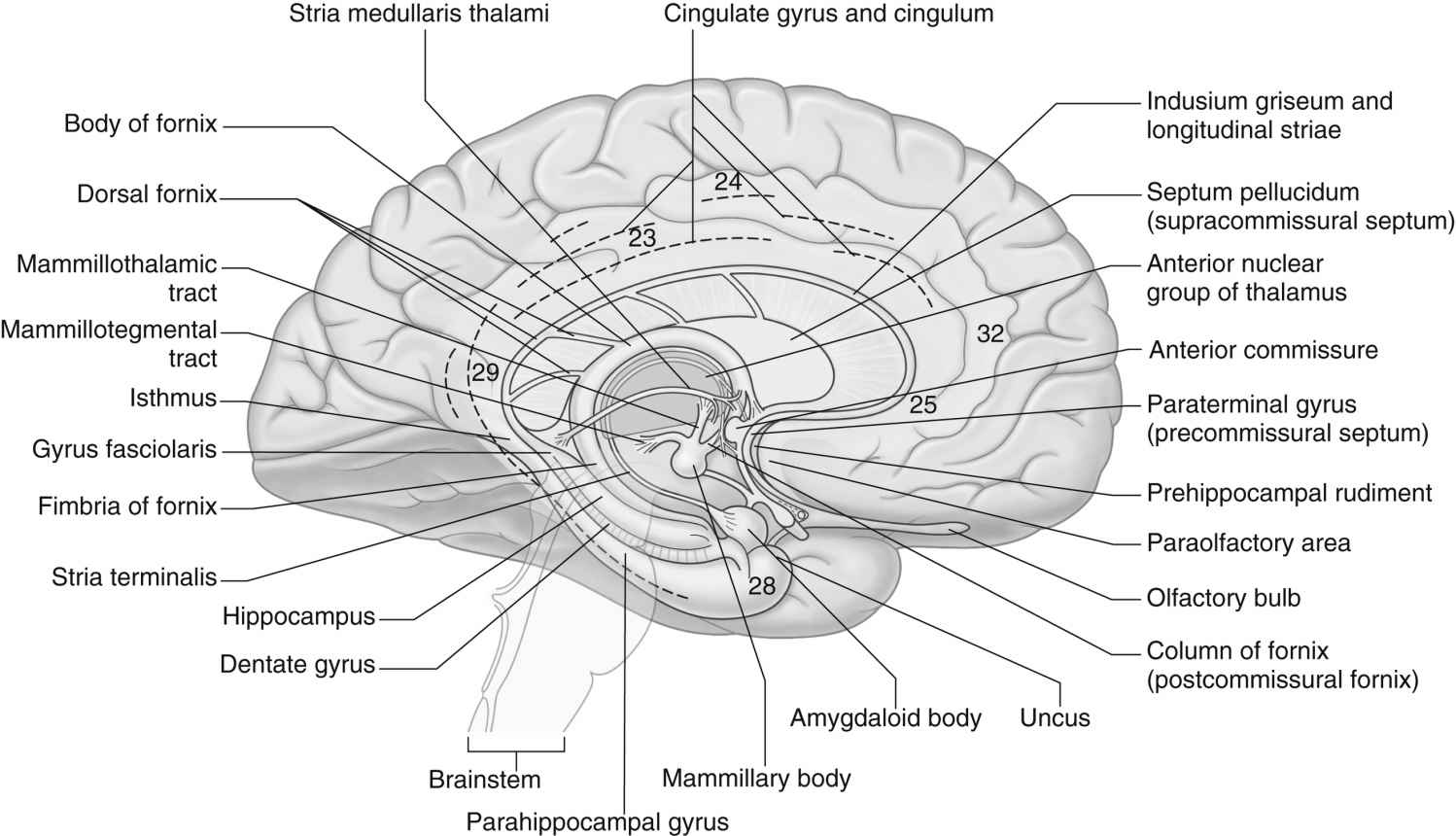
FIG. 10.2 Components of the limbic system. Medial aspect of the left cerebral hemisphere. The approximate locations of some Brodmann areas are indicated. (From Standring S: Gray’s anatomy: the anatomical basis of clinical practice, ed 41, London, UK, 2016, Churchill Livingstone.)
The auditory area lies in the cortex of the superior temporal lobe. Each hemisphere receives impulses from both ears. The visual area is located in the posterior occipital lobe where extremely complex transformations in the signals conveyed by the optic nerve occur. The right occipital cortex receives impulses from the right half of each eye, and the left occipital cortex receives impulses from the left half of each eye. The olfactory area is believed to be located in the medial temporal lobe, and the gustatory area is located nearby at the base of the postcentral gyrus.
Association Areas
Large areas of the cortex remain for which no discrete function is known. These areas are called association areas. They play a major role in the integration of the sensory and motor phases of cortical function by providing complex connections between them.
Limbic System
The principal structural and functional units of the limbic system are the two rings of limbic cortex and a number of related subcortical nuclei, the anterior thalamic nuclei, and portions of the basal nuclei (Fig. 10.2). In general, the limbic system is concerned with a wide variety of autonomic somatosensory and somatomotor responses, especially those involved with emotional states and other behavioral responses. Within the limbic system, the benzodiazepine and opiate receptors have been identified (see Chapters 19, 21, and 22).
The limbic system, which acts in close concert with the hypothalamus, can evoke a variety of autonomic responses, including changes in heart rate, blood pressure, and respiratory rate. This system plays an intimate role in the creation of emotional states, particularly anxiety, fear, and aggression. Stimulation of the limbic system also evokes complex motor responses directly related to feeding behavior. The limbic system has been shown to have major relationships with the reticular formation of the brainstem and is presumed to have a role in the alerting or arousal process. The system is also implicated in the hypothalamic regulation of pituitary activity and may be associated somehow with the memory process for recent events as well. In addition, it is intimately concerned with complex phenomena such as the control of various biologic rhythms, sexual behavior, and motivation.
Basal Ganglia
A cerebral nucleus is a group of neuron cell bodies within the CNS. Five of these deep-lying masses of gray matter are located within the white matter of each hemisphere and are collectively known as the basal ganglia. These masses are the caudate nucleus, the putamen, the globus pallidus, the substantia nigra, and the subthalamic nucleus. Together, they exert a steadying influence on muscle activity. The basal ganglia are an important part of the extrapyramidal motor pathway that connects nuclei with each other, with the cortex, and with the spinal cord. The ganglia also connect with areas in the hindbrain (the red nucleus and the substantia nigra) to assist in the role of smoothing and coordinating muscle movements. Disturbances in these ganglia result in tremor, rigidity, and loss of expressive and walking movements as seen in Parkinson syndrome.5
Diencephalon
The second major division of the forebrain is the diencephalon (Fig. 10.3), which consists of the thalamus and the hypothalamus. The diencephalon also contains the third ventricle and is almost completely covered by the cerebral hemispheres. This portion of the brain has a primary role in sleep, emotion, thermoregulation, autonomic activity, and endocrine control of ongoing behavioral patterns.
The thalamus consists of right and left egg-shaped masses, which compose the greatest bulk of the diencephalon and form the lateral wall of the third ventricle. Each thalamus serves as a relay center for all incoming sensory stimuli except for taste. These impulses are then grouped and transmitted to the appropriate area of the cerebral cortex. Because of its interconnections with the hypothalamus, the limbic system, and the frontal, temporal, and parietal lobes, the thalamus is also integrally involved with emotional activities, instinctive responses, and attentive processes.
The hypothalamus is a group of bilateral nuclei that forms the floor and part of the lateral walls of the third ventricle. Extremely complex in function, the hypothalamus has extensive connections with the autonomic nervous system and with other parts of the CNS. It also influences the endocrine system by virtue of direct and indirect connections with the pituitary gland and the release of its own hormones. In association with these other structures, the hypothalamus participates in the regulation of appetite, water balance, carbohydrate and fat metabolism, growth, sexual maturity, body temperature, pulse rate, blood pressure, sleep, and aspects of emotional behavior. Because of the connection of the hypothalamus with the thalamus and cerebral cortex, emotions can influence visceral responses on certain occasions.6
Midbrain
The midbrain, or mesencephalon, is a short narrow segment of nervous tissue that connects the forebrain with the hindbrain. The midbrain is vital as a conduction pathway and as a reflex control center. Passing through the center of the midbrain is the cerebral aqueduct, a narrow canal that serves to connect the third ventricle of the diencephalon with the fourth ventricle of the hindbrain for the circulation of CSF. In addition, cranial nerves III (oculomotor) and IV (trochlear) originate in the ventral aspect of the midbrain.7
Hindbrain
The hindbrain, or rhombencephalon, consists of the pons, the medulla oblongata, the cerebellum, and the fourth ventricle (Fig. 10.4).
Pons
The pons is literally the bridge between the midbrain and the medulla oblongata as it lies in front of the fourth ventricle and separates it from the cerebellum. It receives many ascending and descending fibers en route to other points in the CNS. The pons also contains the motor and sensory nuclei of cranial nerves V (trigeminal), VI (abducens), VII (facial), and VIII (acoustic). The roof of the pons contains a portion of the reticular formation, and the lower pons assists in the regulation of respiration.
Medulla Oblongata
The medulla oblongata is an expanded continuation of the spinal cord and is located between the base of the skull, the foramen magnum, and the pons. It is anatomically complex and not usually amenable to surgery. Many of the white fiber tracts between the brain and spinal cord decussate as they pass through the medulla. Centers for many complex reflexes are located in the medulla oblongata and include those for swallowing, vomiting, coughing, and sneezing. The originating nuclei of cranial nerves IX (glossopharyngeal), X (vagus), XI (accessory), and XII (hypoglossal) are found in the medulla oblongata (Table 10.1). Because of these originating nuclei, the medulla has an essential role in the regulation of cardiac, respiratory, and vasomotor reflexes. Injuries to the medulla, such as those that accompany basal skull fracture, often prove fatal.
Cerebellum
The cerebellum overlaps the pons and the medulla oblongata dorsally and is located just below the occipital lobes of the cerebrum. It is separated from the cerebrum by the tentorium, a folded layer of the dura mater. Structurally, the cerebellum comprises two hemispheres with a constricted central portion with a bilayered cortex composed of gray matter. Beneath the gray matter are white fiber tracts that extend like branches of a tree to all parts of the cerebellar cortex. Deep within the white matter are masses of gray matter called the cerebellar nuclei. These nuclei connect the cerebellar hemispheres with each other and with areas in the cerebrum, the hindbrain, and the spinal cord.
The cerebellum has no sensory function and does not initiate movement as the cerebrum does. Functionally, it coordinates muscle tone and voluntary movements through important connections via the spinal cord with the proprioceptor nerve fibers in skeletal muscles, tendons, and joints. In addition, the cerebellum is involved in reflexes necessary for the maintenance of equilibrium and posture through its connections with the vestibular apparatus of the inner ear. Damage to the cerebellum does not result in paralysis or sensory loss. The outcome of damage depends on which portion of the structure is involved. Damage to one part can result in loss of balance, nystagmus, and a reeling gait (cerebellar ataxia). Damage to another area can cause disturbances in the postural reflexes. Posterior lobe disturbances result in changes in voluntary movements such as discrepancies in force, direction, and range of movements; lack of precision in movements; and, possibly, intention tremors.
Fourth Ventricle
The fourth ventricle is a diamond-shaped space located between the cerebellum posteriorly and the pons and medulla oblongata anteriorly. The ventricle contains CSF.
Brainstem
Authors disagree to some extent as to what structures collectively constitute the brainstem. All agree that it includes the midbrain, the pons, and the medulla oblongata. Some believe that the diencephalon rightly belongs in the group. Whichever grouping is used, all functions of each structure within it may be considered to be basic activities of the brainstem. All the cranial nerves are attached to the brainstem (if the diencephalon is included) with the exception of the olfactory nerve and the spinal portion of the accessory nerve.
Reticular Formation
The reticular formation lies within the brainstem (including the diencephalon). An important function of the reticular formation is its action as an intermediary between the upper and lower motor neurons of the extrapyramidal system. In this way, the reticular formation facilitates or augments reflex activity and voluntary movements. Its motor neurons can be excitatory or inhibitory in action.
Every pathway carrying information to the brain also contributes afferent fibers to the reticular formation so that it is kept well informed about conditions of both the outside world and the internal organs. Efferent impulses that leave the reticular formation travel to the cerebral cortex and to the spinal cord. By virtue of its location in and connections with the brainstem and diencephalon, the reticular formation participates integrally in their activities.
Another important function of the reticular formation is the activation and regulation of those brain activities related to attention arousal and consciousness. For this reason, it is often called the reticular activating system.
Damage to the reticular formation results in greatly decreased levels of consciousness. When the cerebral cortex is isolated from the reticular activating system by disease or injury of the upper portion of the midbrain, decerebrate posturing occurs. This stereotypical posturing involves the arms and legs held straight out, the toes pointing downward, and the head and neck arching backward. This abnormal posturing results from the dominant effect of the extensor muscles and a lack of inhibition from opposing motor neurons and flexor muscles. The rigidity is accompanied by a profoundly reduced level of consciousness.
Protection of the Brain
Cranial Bones
Eight cranial bones encase the brain and support and protect it from most ordinary bumps and jarring. In the adult, immovable fibrous joints or sutures fuse these bones together to form the rigid walls of the box known as the cranium. The base of the cranium is both thicker and stronger than its roof or walls.
The bones of the cranium are the frontal, right and left parietal, occipital, sphenoid, ethmoid, and right and left temporal bones. The frontal bone forms the anterior roof of the skull and the forehead. Within the frontal bone are the frontal sinuses, which communicate with the nasal cavities. The parietal bones form much of the top and sides of the cranium. The occipital bone forms the back and a large portion of the base of the skull. The two temporal bones are complicated and form part of the sides and a part of the base of the skull. Their inner surfaces are not as smooth and regular as the bones previously mentioned. Parts of the temporal bones articulate with the condyles of the lower jaw, and air cells in the mastoid portions of the temporal bones communicate with the middle ear. The sphenoid bone occupies a central portion of the floor of the skull. It alone articulates with each of the other cranial bones. Its middle portion contains the sphenoid sinuses, which open into the nasal cavity. The upper portion of the sphenoid bone has a marked saddlelike depression, the sella turcica, which holds the pituitary gland. The ethmoid bone is light and has a spongy structure. It is located between the orbital cavities and is a cribriform plate that forms the roof of the nasal cavity and part of the base of the cranium. The ethmoid sinuses open into the nasal cavities.

Full access? Get Clinical Tree
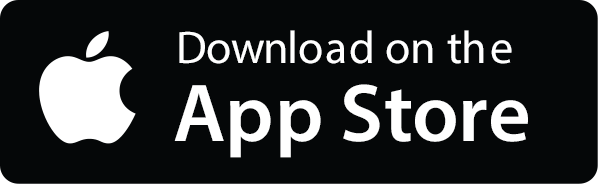
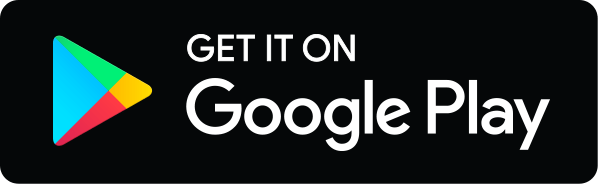