The Management of Sepsis
Paul E. Marik
Sepsis is among the most common reasons for admission to medical ICUs throughout the world. Over the last two decades, the incidence of sepsis in the United States has trebled and is now the 10th leading cause of death [1,2]. Advances in medical technologies, the increasing use of immunosuppressive agents, and the aging of the population have contributed to the exponential increase in the incidence of sepsis. In the United States alone, approximately 750,000 cases of sepsis occur each year, at least 225,000 of which are fatal [1,2]. Septic patients are generally hospitalized for extended periods, rarely leaving the ICU before 2 to 3 weeks. Despite the use of antimicrobial agents and advanced life support, the case fatality rate for patients with sepsis has remained between 20% and 30% over the last two decades [1,2]. This chapter provides an overview of this vast topic with particular emphasis on the management of severe sepsis and septic shock.
Definitions
Sepsis originally meant “putrefaction,” a decomposition of organic matter by bacteria and fungi. Since then, a wide variety
of definitions have been applied to sepsis, including sepsis syndrome, severe sepsis, septicemia, and septic shock [3]. In 1991, the American College of Chest Physicians/Society of Critical Care Medicine developed a new set of terms and definitions to define “sepsis” in a more precise manner [4]. The definitions take into account the findings that sepsis may result from a multitude of infectious agents and microbial mediators and may not be associated with actual bloodstream infection. Although the use of these criteria has been criticized and a “newer” diagnostic schema has been suggested (PIRO, which stands for predisposition, insult infection, response, organ dysfunction), these criteria still provide a useful framework to approach patients with infectious diseases [5]. The term “systemic inflammatory response syndrome” (SIRS) was coined to describe the common systemic response to a wide variety of insults. It is characterized by two or more of the following clinical manifestations: (a) a body temperature of > 38°C or < 36°C; (b) a heart rate greater than 90 beats per minute; (c) tachypnea, as manifested by a respiratory rate of greater than 20 breaths per minute; (d) an alteration of the WBC count of greater than 12,000 cells per mm3, less than 4,000 cells per mm3or the presence of greater than 10% immature neutrophils. When the SIRS is the result of a confirmed infectious process, it is termed “sepsis.” Severe sepsis is defined as sepsis plus either organ dysfunction or evidence of hypoperfusion or hypotension. Septic shock is best defined as systolic pressure less than 90 mm Hg (or a fall in systolic pressure of > 40 mm Hg) or a mean arterial pressure less than 65 mm Hg after a crystalloid fluid challenge of 30 mL per kg body weight (approximately 2,000 mL) in patients with sepsis and in the absence of other causes for hypotension [6]. In a patient previously known to have a low baseline blood pressure, septic shock is defined as a 30% or greater drop in the mean arterial pressure.
of definitions have been applied to sepsis, including sepsis syndrome, severe sepsis, septicemia, and septic shock [3]. In 1991, the American College of Chest Physicians/Society of Critical Care Medicine developed a new set of terms and definitions to define “sepsis” in a more precise manner [4]. The definitions take into account the findings that sepsis may result from a multitude of infectious agents and microbial mediators and may not be associated with actual bloodstream infection. Although the use of these criteria has been criticized and a “newer” diagnostic schema has been suggested (PIRO, which stands for predisposition, insult infection, response, organ dysfunction), these criteria still provide a useful framework to approach patients with infectious diseases [5]. The term “systemic inflammatory response syndrome” (SIRS) was coined to describe the common systemic response to a wide variety of insults. It is characterized by two or more of the following clinical manifestations: (a) a body temperature of > 38°C or < 36°C; (b) a heart rate greater than 90 beats per minute; (c) tachypnea, as manifested by a respiratory rate of greater than 20 breaths per minute; (d) an alteration of the WBC count of greater than 12,000 cells per mm3, less than 4,000 cells per mm3or the presence of greater than 10% immature neutrophils. When the SIRS is the result of a confirmed infectious process, it is termed “sepsis.” Severe sepsis is defined as sepsis plus either organ dysfunction or evidence of hypoperfusion or hypotension. Septic shock is best defined as systolic pressure less than 90 mm Hg (or a fall in systolic pressure of > 40 mm Hg) or a mean arterial pressure less than 65 mm Hg after a crystalloid fluid challenge of 30 mL per kg body weight (approximately 2,000 mL) in patients with sepsis and in the absence of other causes for hypotension [6]. In a patient previously known to have a low baseline blood pressure, septic shock is defined as a 30% or greater drop in the mean arterial pressure.
Three stages in the hierarchy of the host’s response to infection was therefore recognized, namely, sepsis, severe sepsis and septic shock, with sepsis having the best prognosis and septic shock the worst. Data from recently published trials support this postulate, with the mortality from sepsis ranging from 10% to 15%, severe sepsis from 17% to 20% and septic shock from 43 to 54% [6]. The distinction between severe sepsis and septic shock is critically important as it stratifies patients into groups with a low and high risk of dying respectively. It also suggests that a more aggressive treatment strategy may be indicated in patients with septic shock (see Fig. 159.1).
In patients with shock, the serum lactate has long been recognized to be a marker of disease severity and to be useful for disease stratification [7,8]. Septic patients with a lactate above 4 mmol per L are at an increased risk of death and warrant a more aggressive approach to resuscitation [9,10,11]. In addition the rate of lactate clearance has been demonstrated to be a good prognostic marker [12].
Sites of Infection and Bacteriology
The microbiology and primary sources of infection have undergone a remarkable transition over the past 30 years. The predominant pathogens responsible for sepsis in the 1960s and 1970s were Gram-negative bacilli; however, over the last few decades there has been a progressive increase in the incidence of sepsis caused by Gram-positive and opportunistic fungal pathogens [1]. Data from the large sepsis trials published during the last decade indicate that Gram-positive and Gram-negative pathogens are responsible for about 25% of infections each, with a further 15% due to mixed Gram-positive, Gram-negative organisms, with fungal pathogens accounting for between 5% and 10% of cases. This evolution in the spectrum of pathogens has been associated with an increase in the incidence of multiresistant organisms. Although the abdomen was the major source of infection from 1970 to 1990, in the last two decades pulmonary infections have emerged as the most frequent site of infection.
Pathogenesis of Sepsis
The pathogenesis of sepsis is exceeding complex and involves an interaction between multiple microbial and hosts factors. Indeed, after exposure to both Gram-negative and Gram-positive bacteria, macrophages upregulate the expression of over 1,000 genes (and proteins) and downregulate an excess of 300 genes, the net result depending on the complex interrelated interaction of these factors [13]. With advances in molecular biology many of the mysteries of sepsis are being unraveled; however, we have only just embarked on our journey along the “sepsis superhighway.” The reader is referred to many excellent reviews on this topic [14,15,16,17,18,19]. Essentially as noted by William Osler in 1921 “except on a few occasions the patient appears to die from the body’s response to infection rather than from it” [20]. Sepsis can be viewed as an excessively exuberant proinflammatory response with increased production of proinflammatory mediators with activation of leukocytes, mononuclear cells, and the coagulation cascade. The end result is widespread microvascular and cellular injury. The cellular injury results in alteration of cellular and subcellular membranes and receptors, activation of intracellular enzymes, increased apoptosis, mitochondrial dysfunction, and sepsis-related immunosuppression. The excessive proinflammatory responses together with activation of the coagulation cascade are believed to be fundamental events resulting in a systemic microvascular injury. The systemic microvascular injury is a defining characteristic of sepsis and is believed to play a major pathophysiologic role in the progressive organ dysfunction of sepsis.
Organ System Involvement in Sepsis
The Hemodynamic Derangements of Sepsis
Sepsis is characterized by a complex combination of cardiovascular derangements, including vasodilation, hypovolemia, myocardial depression, and altered microvascular flow. In volume resuscitated patients with septic shock, systemic vascular resistance is usually low, contractility and biventricular ejection fractions are reduced while ventricular dimensions and heart rate are increased. Despite these changes, volume resuscitated patients typically have a hyperdynamic circulation with a high cardiac output. However, recent data suggest that up to 60% of patients with septic shock may have a hypodynamic circulation with a deceased ejection fraction (< 45%) and global left ventricular (LV) hypokinesia [21]. Furthermore, increasing evidence suggests that patients with sepsis develop structural injury to the contractile apparatus of the heart that may contribute to the myocardial dysfunction in sepsis. This is evident by elevated levels of troponin and B-type natriuretic peptide in patients with sepsis [22,23,24]. Estimates of LV ejection fraction correlate negatively with increased levels of cardiac troponin in patients with septic shock. These data suggest that all patients with sepsis should undergo serial echocardiography to characterize the hemodynamic pattern, as this impacts on the approach to the use of vasopressor and inotropic agents [21]. In addition, cardiac troponin should be measured to assess the degree of myocardial injury.
Coagulation Activation
Activation of the coagulation cascade with the generation of fibrin is a pathologic and physiologic hallmark of sepsis that occurs in both the intravascular and extravascular compartments [25]. Intravascular coagulation is characterized by diffuse microvascular thrombosis that contributes to widespread ischemic organ damage. Activation of coagulation during sepsis is primarily driven by the tissue factor pathway. Fibrin formation in sepsis likely results from both increased fibrin generation and impaired fibrin degradation. Inhibition of fibrinolysis
is primarily due to increases in plasminogen activator inhibitor-1 (PAI-1). Downregulation of the anticoagulant Protein C pathway also plays an important role in the modulation of coagulation and inflammation in sepsis. Because activation of the coagulation cascade almost all septic patients are thrombocytopenic (or have a falling platelet count), and indeed a normal platelet count makes the diagnosis of sepsis unlikely. An elevated D-dimer, thrombin–antithrombin complexes and a prolonged prothrombin time are found in the majority of patients with severe sepsis while antithrombin, protein C, and protein S levels are significantly decreased. Replacement of coagulation factors with fresh frozen plasma ([FFP] and cryoprecipitate if the fibrinogen is less than 100 mg per dL) is only indicated in patients with clinical evidence of bleeding. Although it had previously been assumed that such therapy “fuels the fire of DIC,” there is no evidence that the infusion of plasma products stimulates the ongoing activation of coagulation [26].
is primarily due to increases in plasminogen activator inhibitor-1 (PAI-1). Downregulation of the anticoagulant Protein C pathway also plays an important role in the modulation of coagulation and inflammation in sepsis. Because activation of the coagulation cascade almost all septic patients are thrombocytopenic (or have a falling platelet count), and indeed a normal platelet count makes the diagnosis of sepsis unlikely. An elevated D-dimer, thrombin–antithrombin complexes and a prolonged prothrombin time are found in the majority of patients with severe sepsis while antithrombin, protein C, and protein S levels are significantly decreased. Replacement of coagulation factors with fresh frozen plasma ([FFP] and cryoprecipitate if the fibrinogen is less than 100 mg per dL) is only indicated in patients with clinical evidence of bleeding. Although it had previously been assumed that such therapy “fuels the fire of DIC,” there is no evidence that the infusion of plasma products stimulates the ongoing activation of coagulation [26].
Pulmonary
Sepsis is by far the most common cause of the acute respiratory distress syndrome (ARDS) [27,28,29]. The mortality rate for patients with sepsis complicated by ARDS has been reported to be as high as 60%. The pathophysiology and management of patients with ARDS has been extensively reviewed in the literature.
Renal
Acute renal failure is a serious complication in patients with sepsis. Despite improvements in the support of these patients, the mortality rate remains consistently above 50%. It is, therefore, essential that all patients with sepsis be aggressively resuscitated in an attempt to prevent this complication. The pathogenetic mechanisms leading to ARF in patients with sepsis are unclear; however, mediator-induced cytotoxicity, alterations in renal perfusion and apoptosis have been suggested [30,31].
Gastrointestinal
The most important gastrointestinal complications occurring in patients with sepsis include gastric stress ulceration, a diffuse splanchnic mucosal injury with increased intestinal permeability and intrahepatic cholestasis.
Nervous and Musculoskeletal
Septic encephalopathy is an acute, reversible, generalized disturbance in cerebral function [32,33]. Septic encephalopathy is essentially a diagnosis by exclusion as many factors such as sedative drugs, encephalitis, liver or renal failure, hypoperfusion, fever, adrenal insufficiency, cerebral vascular accidents, and drug fever either alone or in combination may result in disturbed cerebral function. Electroencephalography is useful in confirming the diagnosis of septic encephalopathy and allows assessment of the severity of the encephalopathy. Treatment is essentially supportive.
Critical illness polyneuropathy (CIP), as initially described by Bolton et al. in 1984, is a sensorimotor polyneuropathy that is often a complication of sepsis and multiorgan failure, occurring in 70% of such patients [34,35,36]. Postmortem examination of peripheral nerve specimens from patients with CIP has shown primary degeneration of motor and sensory nerves that supply the limbs and respiratory system. Although this denervation is more widespread and severe in the distal muscle groups, the phrenic nerve, diaphragm, and intercostals muscles are also involved. Classically, CIP is associated with a symmetric predominantly distal paresis, with legs involved worse than arms, along with impaired sensory testing in the feet and hyporeflexia. CIP is difficult to diagnose clinically and is often suspected when critically ill patients are otherwise improving yet continue to have difficulty in weaning from mechanical ventilation.
In addition to neuropathy, weakness in critically ill septic patients may stem from disturbances in the structure or function of muscle per se. According to biopsy and neurophysiologic studies, myopathies occur much more frequently during critical illness than was previously recognized. Myopathic changes have been demonstrated by electromyographic examination and biopsy in many septic ICU patients. The changes are often mild and usually accompany CIP. In other patients however, myopathy is the predominant finding. This myopathy has been called critical illness myopathy.
Sepsis and Multisystem Organ Dysfunction
The ultimate cause of death in patients with sepsis is multiple organ failure. Typically, patients will first develop a single organ failure and then, if the disease remains unchecked, will progressively develop failure/dysfunction of other organ systems. There is a close relationship between the severity of organ dysfunction on admission to an ICU and the probability of survival. The pathogenesis of organ dysfunction is multifactorial and incompletely understood. Tissue hypoperfusion and hypoxia are dominant factors. Multisystem organ dysfunction has an extraordinarily high mortality and, for many patients, the support of this syndrome does not improve survival but rather prolongs the dying process.
Clinical Features and Diagnosis of Sepsis
Sepsis is a systemic process with a variety of clinical manifestations. The initial symptoms of sepsis are nonspecific and include malaise, tachycardia, tachypnea, fever, and sometimes hypothermia. Although most patients with sepsis have an elevated white cell count, some patients present with a low white cell count, which in general, is a poor prognostic sign. A band count in excess of 10% has been reported to have a high specificity (92%) but low sensitivity for the diagnosis of sepsis (43%) [37]. Other clinical manifestations include altered mental status, hypotension, respiratory alkalosis, metabolic acidosis, hypoxemia with acute lung injury, thrombocytopenia, consumptive coagulopathy, proteinuria, acute tubular necrosis, intrahepatic cholestasis, elevated transaminases, hyperglycemia, and hypoglycemia. Patients may present with clinical features of a localized site of infection, such as cough, tachypnea and sputum production due to pneumonia; flank pain and dysuria with urinary tract infection and abdominal pain with intra-abdominal infection.
The manifestations of sepsis can sometimes be quite subtle, particularly in the very young, the elderly, and those patients with chronic debilitating or immunosuppressing conditions. These patients may present with normothermia or hypothermia. The failure to generate a temperature greater than 99.6°F (37.5°C) in the first 24 hours of clinical illness, has been associated with an increased mortality rate. An altered mental state or an otherwise unexplained respiratory alkalosis may be the presenting feature of sepsis.
The signs and symptoms of systemic inflammation are not useful in distinguishing infectious from noninfectious causes of SIRS. Furthermore, a bacterial pathogen is not isolated in
all patients with sepsis. Consequently, a number of biomarkers have been evaluated as more specific indicators of infection, including procalcitonin (PCT) and triggering receptor expressed on myeloid cells (TREM-1). PCT, a propeptide of calcitonin, is normally produced in the C-cells of the thyroid. In healthy individuals, PCT levels are very low (< 0.1 ng per mL). In patients with sepsis, however, PCT levels increase dramatically, sometimes to more than several hundred nanograms per milliliter. The exact site of PCT production during sepsis is uncertain; however, mononuclear leukocytes and the liver seem to be the major sources of PCT. TREM-1 is a monocyte receptor that is upregulated by bacterial and fungal pathogens [38]. The ligand for TREM-1 is unknown. A soluble form of TREM-1 (sTREM-1) is released from activated phagocytes and can be found in body fluids. The use of these biomarkers has not gained widespread acceptance presumable due to the cost of the tests and the uncertain diagnostic accuracy.
all patients with sepsis. Consequently, a number of biomarkers have been evaluated as more specific indicators of infection, including procalcitonin (PCT) and triggering receptor expressed on myeloid cells (TREM-1). PCT, a propeptide of calcitonin, is normally produced in the C-cells of the thyroid. In healthy individuals, PCT levels are very low (< 0.1 ng per mL). In patients with sepsis, however, PCT levels increase dramatically, sometimes to more than several hundred nanograms per milliliter. The exact site of PCT production during sepsis is uncertain; however, mononuclear leukocytes and the liver seem to be the major sources of PCT. TREM-1 is a monocyte receptor that is upregulated by bacterial and fungal pathogens [38]. The ligand for TREM-1 is unknown. A soluble form of TREM-1 (sTREM-1) is released from activated phagocytes and can be found in body fluids. The use of these biomarkers has not gained widespread acceptance presumable due to the cost of the tests and the uncertain diagnostic accuracy.
Blood cultures are considered to provide the clinical gold standard for the diagnosis of bacterial infections. However, blood cultures are only positive in between 20% and 30% of patients with sepsis; moreover, it takes 2 to 3 days before the results become available. Molecular methods based on polymerase chain reaction (PCR) technology have been developed for infection diagnosis and pathogen identification. These methods offer a new approach based on detection and recognition of pathogen DNA in the blood, or indeed other clinical samples, with the potential to obtain results in a much shorter time frame (hours) than is possible with conventional culture. PCR based pathogen detection depends on the ability of the reaction to selectively amplify specific regions of DNA, allowing even minute amounts of pathogen DNA in clinical samples to be detected and analyzed. This technique holds great promise and may revolutionize our approach to the diagnosis of bacterial, fungal, and viral infections.
Management of Sepsis
The management of patients with severe sepsis and septic shock is complex requiring multiple concurrent interventions with close monitoring and frequent re-evaluations. These patients are best managed in intensive care units by physicians experienced in the management of critically ill septic patients. The reader is referred to the “Surviving Sepsis Campaign guidelines for the management of severe sepsis and septic shock”; these guidelines were developed by a number of international critical care organizations and should serve as the framework for the management of patients with sepsis [10].
The current strategy for the management of patients with sepsis is largely based on treating or eliminating the source of infection, timely and appropriate usage of antimicrobial agents, hemodynamic optimization, and other physiologic organ supportive measures (see Table 159.1). Attempts at downregulating the proinflammatory response with novel agents directed at specific proinflammatory mediators has uniformly met with failure. However, both activated protein C (APC) and glucocorticoids (low dose) are immunomodulators that have been demonstrated to improve the outcome of patients at high risk of death.
Table 159.1 Suggested Fluid Resuscitation Algorithm for Hemodynamic Instability of Severe Sepsis and Septic Shock | |
---|---|
|
It has become increasingly apparent that in many patients there is a long delay in both the recognition of sepsis and the initiation of appropriate therapy. This has been demonstrated to translate into an increased incidence of progressive organ failure and a higher mortality. Kumar et al. investigated the relationship between the duration of hypotension prior to antimicrobial administration in 2600 patients with sepsis induced hypotension [39]. They reported that the risk of dying increased progressively with time to receipt of the first dose of antibiotic. Furthermore, there was a 5% to 15% decrease in survival with every hour delay over the first 6 hours. In the ENHANCE study, the mortality was 33% if drotrecogin alpha-activated (APC) was given within the first 24 hours of admission as compared to 52% if it was given on day 3 of hospitalization [40].
Levy et al. retrospectively analyzed the Sequential Organ Failure Assessment scores during the first 48 hours in 1,036 severely septic patients [41]. From baseline to day 1, the direction of change in cardiovascular, renal, respiratory, hematologic, and hepatic functions independently predicted 28-day mortality. The implications of this study is that if organ dysfunction is not improving during the first day of severe sepsis, the mortality risk is significantly increased, underscoring the importance of early recognition and therapeutic intervention to prevent sequential organ dysfunction [42]. Similarly, Rivers et al. demonstrated that early (within 6 hours) clearance of lactate is associated with improved outcome in severe sepsis and septic shock [12].
The concept that early aggressive treatment (within the first 6 hours of admission to hospital) of patients with severe sepsis and sepsis shock reduces sequential organ failure and improves survival has been demonstrated in the “landmark” study by Rivers et al. [43]. In this study, early aggressive therapy that optimized cardiac preload, afterload, and contractility in patients with severe sepsis and septic shock improved survival. The patients in the early-therapy group received, on average, approximately 1,500 mL more in total fluids in the first 6 hours of treatment than did the standard-therapy group and had a significantly higher mean arterial pressure (mean [±SD], 95 ± 19 vs. 81 ± 18 mm Hg; p < 0.001). Mortality was 30.5% in the group receiving early goal-directed treatment, as compared with 46.5% in the control group (p = 0.009). This strategy for managing patients with severe sepsis and septic shock has been called “early goal-directed therapy (EGDT).”
While the concept of early, as opposed to delayed, volume resuscitation and the timely initiation of appropriate antibiotics in patients suffering from severe sepsis and septic shock is a scientifically sound concept, the author believes that the major pillars on which EGDT is based (central venous pressure [CVP] > 8 mm Hg, ScvO2 > 70% and blood transfusion) may be flawed (see later) [44]. A more evidence-based approach is provided in Figure 159.1.
Identification and Eradication of the Source of Infection
One of the most challenging features of the sepsis syndrome is that of identifying and eradicating, as early as possible, the source of infection. The majority of patients presenting with severe sepsis usually have a pulmonary, genitourinary, primary blood stream, intra-abdominal, or intravenous catheter as a source of infection. Recent studies have demonstrated that in approximately 75% of patients with presumed sepsis, an
etiological agent can be isolated, these being equally divided amongst Gram-positive and Gram-negative organisms. It has been known for centuries that, unless the source of the infection is controlled, the patient cannot be cured of his or her infective process and that death will eventually ensue. Surgical control or percutaneous drainage of the infective process is therefore essential in most patients with severe intra-abdominal infections; recovery will not occur without them. Infected central venous catheters must be removed from patients with catheter related sepsis [45].
etiological agent can be isolated, these being equally divided amongst Gram-positive and Gram-negative organisms. It has been known for centuries that, unless the source of the infection is controlled, the patient cannot be cured of his or her infective process and that death will eventually ensue. Surgical control or percutaneous drainage of the infective process is therefore essential in most patients with severe intra-abdominal infections; recovery will not occur without them. Infected central venous catheters must be removed from patients with catheter related sepsis [45].

Full access? Get Clinical Tree
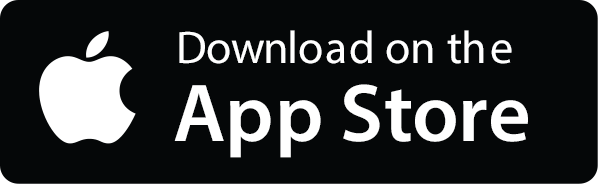
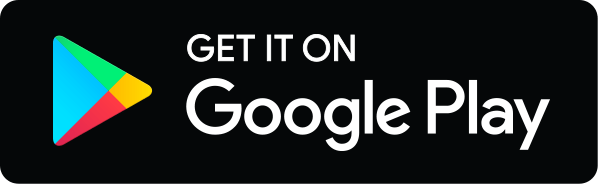