Because critically ill children may occasionally present for emergency surgery, all anesthesiologists should have a working knowledge of the most common critical illnesses and their therapies. In this chapter, we review the clinical features of these illnesses, and also describe the most current Pediatric Advanced Life Support (PALS) guidelines and the use of inotropic medications and methods of providing invasive access in children.
Pediatric Advanced Life Support
Every few years, the American Heart Association (AHA) publishes updated Pediatric Advanced Life Support (PALS) guidelines, incorporating the latest findings in pediatric resuscitation science. At the time of this writing, the most recent version was published in 2020. Since the 2010 guidelines, the focus of resuscitation is to use the “CAB” (Circulation, Airway, Breathing) approach, with an emphasis on achieving and maintaining optimum cerebral and coronary perfusion via quality cardiopulmonary resuscitation (Q-CPR). Chest compressions should begin immediately upon recognition of cardiac arrest while trying to establish airway and breathing (simultaneous but secondary actions). It is easier and faster to establish vital organ blood flow through effective chest compressions during a no-flow state of cardiac arrest before gathering equipment and securing an airway.
The PALS update in 2020 focuses on CPR quality and postresuscitation care . CPR quality is assessed by the fraction, depth, and rate of chest compressions ( Table 38.1 ). These can be measured using technologically advanced defibrillators and defibrillator pads that provide real-time feedback on CPR quality. The use of end tidal CO 2 (EtCO 2 ) and arterial blood pressure monitoring are also useful guides of CPR quality. Postresuscitation care focuses on maintaining normal core temperature, adequate blood pressure for coronary and cerebral perfusion, and normal oxygenation.
|
Circulation
When encountering the pulseless child, chest compressions should be initiated immediately. In infants, the best way to perform chest compressions is to encircle the chest with your hands, with the thumbs placed one finger-width below the intermammary line over the midsternum. For older children, the chest is compressed by placing the heel of your hand two-finger breadths above the lower end of the sternum and with a depth of at least 1/3 the anterior-posterior diameter of the chest (approximately 2 inches [or 5 cm]). Avoid compressions over the lower third of the sternum, which may cause trauma to internal abdominal organs. Compressions are delivered at a rate of 100 to 120 times per minute. The most important aspect of pediatric CPR is to maintain the frequency and depth of the compressions while minimizing interruptions and allowing for full chest recoil. Rotate compressors every 2 minutes to minimize fatigue and ensure effective compressions.
During CPR, peripheral venous access should be obtained, and if unsuccessful after 3 attempts or 90 seconds, whichever comes first, intraosseous (IO) vascular access is indicated. Isotonic fluids, such as lactated Ringer solution or normal saline, should be rapidly administered in 10 to 20 mL/kg doses, and continued until blood pressure is normalized. If unable to establish normal-for-age hemodynamics after ~60 mL/kg isotonic fluid administration, vasoactive medications should be considered with choice of agent dependent on hemodynamic profile. Children with hemorrhagic shock should receive uncrossmatched type O, Rh-negative packed red blood cells or whole blood. (Type-specific blood can be administered if the patient’s blood type is already known) Medications that can be administered through the endotracheal tube and absorbed from the bronchial tree include lidocaine, atropine, naloxone, and epinephrine (mnemonic = LANE).
Pulseless electrical activity (PEA) may be seen during cardiac arrest in children and is often caused by a reversible process. Thus the differential diagnosis should be kept in mind by remembering the Hs and the Ts ( Table 38.2 ).
Hypoxemia | Tamponade (pericardial) |
Hypovolemia | Tension pneumothorax |
Hypothermia | Thromboembolism (myocardial ischemia, pulmonary embolus) |
Hyper/Hypokalemia | Toxins |
Hydrogen ions (acidosis) | Trauma |
Airway and Breathing
Assuming the child in cardiac arrest did not have previous airway management, it is addressed once chest compressions have begun. Proper airway positioning is important to maximize oxygenation and ventilation. Usual airway maneuvers (e.g., head tilt, chin lift, and/or jaw thrust) are performed to maintain airway patency with bag-mask ventilation; securing the airway with tracheal tube placement is preferred but a supraglottic airway may be acceptable when tracheal intubation is unobtainable or when attempts at tracheal intubation would compromise the provision of high-quality CPR. Hyperventilation is a known risk during CPR with an advanced airway and may increase intrathoracic pressure, impeding venous return and decreasing cardiac output. When an advanced airway is in place, breaths do not need to be coordinated with compressions and should be given at a rate of 20 to 30 breaths per minute (approximately one breath every 2–3 seconds). Before an advanced airway, compression and bag-mask breaths should be coordinated. In single-rescuer CPR, it should be a ratio of 30 compressions to 2 breaths; and for two-rescuer CPR in children, it should be 15 compressions to 2 breaths.
Disability and Drugs
Disability
Once circulation and breathing are addressed, the child’s neurologic status should be evaluated. A Glasgow Coma Scale (GCS) score is calculated while assessing for focal neurologic injury. Abnormal pupillary responses (e.g., unilateral dilated pupil) may suggest impending cerebral herniation and should be promptly evaluated and addressed. Maintaining cervical spine immobilization, especially if there is a suspected traumatic mechanism of injury, is essential until the spine can be cleared clinically or radiographically. Cervical spinal cord trauma may cause neurologic deficits and neurogenic shock, which is characterized by vasodilation and hypotension without tachycardia. Hypoglycemia should immediately be treated as it can lead to additional neurologic injury.
Drugs
Epinephrine is the most important drug in pediatric cardiac arrest. The initial resuscitation dose for pediatric cardiac arrest is 0.01 mg/kg (0.1 mL/kg of standard 1:10,000 concentration) via the IV or IO route; alternatively, 0.1 mg/kg can be administered via an endotracheal tube if vascular access is not available. This dose can be repeated every 3 to 5 minutes if there is no response to the initial dose. Amiodarone (5 mg/kg) or lidocaine (1 mg/kg) may be used for ventricular tachycardia or ventricular fibrillation that is refractory to defibrillation and consultation with a pediatric cardiologist should be considered if an arrest situation is unresponsive to epinephrine doses and alternative agents are being considered. Atropine (0.02 mg/kg) may be helpful in cases of bradycardia, but when bradycardia is caused by hypoxemia, oxygen is the most important drug to address the underlying arrest physiology.
Exposure and Environment
Once ventilation and circulation are stabilized, all the child’s clothing should be removed to search for additional traumatic or toxic injuries. Hypothermia and hyperthermia should be aggressively treated to prevent clotting disorders and to prevent further focal neurologic injury. Profound hypothermia resulting in arrest may warrant extracorporeal rewarming; consultation with intensive care specialists should be pursued.
Post arrest Care
The foundation of postarrest care is focused on minimizing secondary injury and this may be required in the operative environment if the postarrest patient requires operative care or if they require anesthesia expertise to facilitate imaging or other diagnostic/therapeutic maneuvers.
After return of spontaneous circulation (ROSC) or after cannulation onto extracorporeal support during emergency CPR (E-CPR), efforts should be focused on ensuring age-appropriate physiology that minimizes metabolic demand and end organ injury. Hypertension and hypotension can both be injurious but the postarrest patient is most at risk for hypotension and blood pressure should be targeted to age-standardized norms that prevent end-organ hypoperfusion. Metabolic demand should be minimized by avoiding fevers, shivering, and seizures. This may require around-the-clock administration of antipyretic medications and temperature-regulating devices such as a thermoregulation blanket. (Pediatric studies of targeted temperature management [TTM] of the postarrest patient have not demonstrated benefit of empiric cooling) . The postarrest patient may require neuromuscular blockade to limit shivering, and for arrests where neurologic status is altered from baseline after arrest, continuous electroencephalography (EEG) monitoring should be considered to monitor subclinical status epilepticus to treat quickly and minimize metabolic demand. Hypo- and hyperglycemia should be avoided and routine glucose monitoring should be instituted to minimize effects from these metabolic derangements.
Treatment Algorithms
Because it is difficult to memorize the specific treatment algorithms for the various possible dysrhythmias, anesthesiologists should have immediate access to the most current AHA guidelines. We recommend that each anesthesia practitioner have a copy (or have access to a copy in each anesthetizing location) of a PALS Pocket Reference Card (AHA 20-1118) that can be easily obtained from many Internet retailers.
Common Pediatric Critical Illnesses
Status Epilepticus
Continuous seizure activity lasting for more than 5 minutes or multiple seizures without return to baseline is considered status epilepticus and should prompt immediate intervention. Common causes in children include epilepsy, head trauma, hypoxic-ischemic encephalopathy, infection (e.g., meningitis, encephalitis), medication toxicity, genetic or metabolic disease, or electrolyte disturbance (e.g., hypoglycemia, hyponatremia). The cause is unknown in approximately 50% of cases. Empiric broad-spectrum antibiotics with or without antiviral therapy should be administered if an infection is suspected. Spinal fluid should ideally be collected before antimicrobial administration, if possible. Head CT is indicated unless the diagnosis is obvious and not related to structural brain abnormalities.
If untreated, status epilepticus may result in neurologic injury or death. Common causes of injury from status epilepticus include hypoventilation and pulmonary aspiration. Immediate treatment consists of ensuring airway patency, supplemental oxygen, and administration of an anticonvulsant. Neuromuscular blockade (NMB) will facilitate endotracheal intubation but may mask recognition of continued seizure activity; alternative approaches to assess ongoing seizure activity (e.g., EEG) should be considered if NMB has been administered. Hypotension may result from prolonged seizure activity or cardiodepressant effects of anticonvulsant drugs and should be treated with volume expansion and vasopressors. The possibility of neurologic damage is increased with persistent hypoxemia, hypotension, hypoglycemia, and metabolic acidosis. Hyperthermia should be aggressively treated to minimize cerebral metabolic oxygen consumption. Immediate EEG evaluation to evaluate for nonconvulsive status epilepticus may aid in diagnosis if a patient is not returning to baseline mental status.
Treatment guidelines from the American Epilepsy Society have been published. Initial treatment should consist of an intravenous benzodiazepine such as lorazepam, midazolam, or diazepam. If vascular or intraosseous access is not rapidly obtained, midazolam can be administered via the intramuscular, buccal, intranasal, or rectal route and diazepam can be administered via the rectal route.
If an initial dose of a benzodiazepine is not effective in quieting seizure activity, most guidelines call for repeat administration with a second dose. Next-line agents may be used if patients are not responding to the initial treatment with benzodiazepines. These include levetiracetam, valproic acid, or fosphenytoin. Levetiracetam is often used as the first next-line agent because of its favorable side effect profile and minimal respiratory depression, however, a recent study showed no difference between these three medications in effectiveness or adverse effects.
Phenobarbital, 15 to 20 mg/kg infused slowly, can be used as an adjunct anticonvulsant. Side effects include respiratory depression (especially when used in combination with benzodiazepines), hypotension, and prolonged sedation. In young infants, it may be more effective than other antiepileptic medications.
When status epilepticus remains unresponsive to conventional therapies, it should be aggressively managed using adjunct medication infusions in consultation with a pediatric neurologist. Common drug infusions for status epilepticus include: midazolam, pentobarbital, and ketamine; with dosing adjusted based on EEG and clinical seizure burden. Side effects of each agent can be significant and local formularies should be consulted before initiation to be vigilant about side effects such as cardiac depression (that may require inotropic therapy) and granulocytopenia. Inhalational anesthetics or propofol may also be used to suppress seizures in refractory cases, with guidance provided by pediatric neurology colleagues.
Shock
Shock describes a state of insufficient delivery of oxygen to meet the metabolic demands of the tissues. There are three progressive stages of shock: compensated, uncompensated, and irreversible.
In compensated shock , blood pressure and organ perfusion are maintained by activation of compensatory mechanisms, such as increasing heart rate and systemic vascular resistance (SVR). Because neonates and young infants cannot significantly increase stroke volume, cardiac output (stroke volume × heart rate) is augmented by increasing heart rate. In this initial stage, the patient may demonstrate cool, pale extremities with delayed capillary refill (>4 seconds), and decreased urine output. In uncompensated shock , cellular dysfunction, ischemia, and endothelial injury occur when compensatory mechanisms begin to fail. The patient will demonstrate an altered level of consciousness and decrease in urine output. The onset of tachypnea may signify the body’s attempt to compensate for a new metabolic acidosis. Bradycardia is an ominous sign in an infant because it significantly decreases cardiac output and may portend impending cardiac arrest. Hypotension is a late finding of pediatric shock and places the patient at risk for multiorgan system failure. Irreversible shock occurs when there is unrecoverable end-organ damage.
There are four major categories of shock described by PALS:
- 1.
Hypovolemic shock is the most common cause and results from decreased intravascular volume. It can be caused by water and electrolyte loss (e.g., vomiting, diarrhea, renal losses, or heat stroke), blood loss (e.g., trauma), or plasma losses (e.g., burns, nephrotic syndrome). Initial management is focused on replacing lost fluids after the initial CAB (circulation-airway-breathing) assessment of the primary survey. Fluid resuscitation is guided by normalization of heart rate, peripheral perfusion, and urine output (goal > 0.5–1 mL/kg/h). If blood loss is obvious, blood products should be the initial replacement fluid. Hypovolemic shock from blood loss is classified into four categories based on the estimated blood volume deficit. When available, fresh whole blood (<48 hours old) should be transfused because it contains red blood cells, platelets, and clotting factors. When whole blood is unavailable, a balanced resuscitation with 1:1:1 packed red blood cells (PRBCs): fresh frozen plasma (FFP): platelets should be considered in alignment with institutional massive transfusion practices, as patients transfused with large amounts of blood products will usually develop a dilutional coagulopathy if coagulation factor and platelet losses are not also corrected. If blood is needed immediately, type O, Rh-negative blood is the “universal donor” and should be used until type-matched products are available. In nonhemorrhagic, hypovolemic shock, isotonic crystalloid fluids are used as the initial resuscitation fluid, commonly normal (0.9%) saline or Lactated Ringers (LR). Patients should be given replacement of their estimated fluid losses (current weight [kg] – prior weight [kg] = estimated liters of fluid loss) with resuscitation guided by normalization of heart rate and markers of end-organ perfusion.
- 2.
Distributive shock is caused by abnormalities in vasomotor tone, such as vasodilatation, and results in relative hypovolemia. Common causes include sepsis, neurogenic shock, and anaphylaxis. Septic shock is caused by overwhelming infection and manifests as a combination of hypovolemia, altered vascular tone, cardiac pump failure, and cellular metabolic derangements, resulting in a profound metabolic acidosis. Initially, the extremities can be warm and well-perfused or cold with high systemic vascular resistance and delayed capillary refill. In warm septic shock, patients have vasodilation which is caused by inflammatory mediators (e.g., endotoxin, tumor necrosis factor, and interleukin-1) and is manifested clinically as diastolic hypotension and a wide pulse pressure. In cold septic shock, patients present with vasoconstriction, cool extremities, normal to elevated blood pressures, and may have myocardial dysfunction. If untreated, persistent septic shock will rapidly lead to multiorgan system failure and possibly death. Septic shock requires identification and treatment of the sources of infection. These patients usually require rapid administration of isotonic fluids (>60 mL/kg) and may require vasopressors. Vasopressor selection is dictated by the clinical presentation and may require an agent that preferentially targets vascular tone (e.g., phenylephrine, norepinephrine), cardiac function (e.g., low-dose epinephrine, dobutamine), or both. For patients with vasopressor-refractory shock and those with adrenal insufficiency, consider use of hydrocortisone 2 mg/kg IV bolus (max 100 mg). Further management includes monitoring for end-organ perfusion abnormalities and supporting physiology to improve cardiac output and reverse the shock state. Neurogenic shock may be seen in spinal cord injury, usually at the cervicothoracic area. Loss of sympathetic vascular tone causes bradycardia, vasodilation, and hypotension. Anaphylaxis may manifest as an acute allergic response resulting in venodilation, arterial vasodilation, increased capillary permeability, and pulmonary vasoconstriction. Epinephrine is the firstline vasopressor in the treatment of anaphylaxis. In distributive shock, Trendelenburg positioning (head down with cervical spine immobilized if indicated) and administration of isotonic fluids may restore circulatory stability. Vasopressor drugs with direct alpha-1-adrenergic activity may be necessary to improve vascular tone and decrease risk for hypotension and myocardial ischemia.
- 3.
Cardiogenic shock can be caused by congenital heart disease, prolonged arrhythmias (e.g., supraventricular tachycardia), acquired cardiomyopathies (Kawasaki disease with coronary aneurysms), infection, and drug intoxications. Central venous pressure monitoring and physical examination will guide cautious fluid administration. These patients will usually benefit from inotropic support and afterload reduction. Severe cases may require mechanical circulatory support with implanted cardiac support devices and/or extracorporeal support.
- 4.
Obstructive shock is caused by extracardiac causes of circulatory failure, often caused by poor right ventricular cardiac output. Obstructive shock can occur as a result of tension pneumothorax, pulmonary embolism, pulmonary hypertension, cardiac tamponade from pericardial effusion, and restrictive pericarditis. Treatment for obstructive shock requires relief of the obstruction (e.g., needle decompression and chest tube placement for tension pneumothorax; anticoagulation and/or thrombectomy for pulmonary embolism, etc.). In the interim, treatment with fluid (additional preload) may help overcome the obstruction unless the right ventricle is volume overloaded and failing as seen with pulmonary hypertension. In those situations, inotropic support for the right ventricle may be useful while treating the etiology of obstructive shock.
Neonates presenting in shock in the first few days of life are unique because it may indicate undiagnosed congenital heart disease with ductal-dependent systemic circulation. These babies are asymptomatic at birth because aortic blood flow is provided by the patent ductus arteriosus (PDA). However, when the PDA closes, shock develops from inadequate systemic output. These patients usually have left-sided obstructive lesions, such as hypoplastic left heart syndrome, aortic valve stenosis, interrupted aortic arch, or coarctation of the aorta. Prostaglandin E1 (0.05–0.1 μg/kg/min) will keep the ductus open until a palliative or definitive surgical procedure is performed.
Inotropic and Vasoactive Agents in Children
Dopamine
Dopamine is mainly a central neurotransmitter but is also found peripherally in the sympathetic nervous system and in the adrenal medulla. Dopamine stimulates dopaminergic, alpha and beta receptors in the brain and peripheral vascular beds, and depending on its concentration, can produce vascular dilatation or constriction.
Dopamine is used to treat hypotension and oliguria in children with distributive, septic, or cardiogenic shock when volume resuscitation has been ineffective. Low infusion rates (1–5 μg/kg/min) stimulate dopamine receptors and increase glomerular filtration and renal blood flow. Moderate doses (5–10 μg/kg/min) increase heart rate and improve myocardial contractility via beta receptor stimulation and release of norepinephrine from nerve terminals, resulting in an increase in systolic blood pressure and minimal change in diastolic pressure. Higher doses (>10 μg/kg/min) increase SVR by alpha receptor stimulation and vasoconstriction.
Adverse effects of dopamine include tachycardia, hypertension, dysrhythmias, and increased myocardial oxygen consumption. Dopamine decreases Pao 2 by inhibiting hypoxic pulmonary vasoconstriction, and it can depress the ventilatory response to hypoxemia by as much as 60%. More recent data suggests sequelae on innate immune responses and impacts on the hypothalamic-pituitary-adrenal axis, thus it has been used sparingly at some institutions as a first-line vasoactive agent.
Dopamine clearance decreases by about 50% during the first 20 months of life, and then continues to decrease by lesser amounts throughout childhood. All studies of dopamine pharmacokinetics in seriously ill children show substantial inter individual variation in pharmacokinetic parameters. Thus there is a large variation in the dose required to achieve a desired clinical response.
Dobutamine
Dobutamine has greater selectivity for beta-1 and beta-2 receptors than dopamine. At a dose range of 5 to 20 μg/kg/min, it enhances myocardial contractility and increases stroke volume, with less increase in heart rate than dopamine. It decreases SVR and PVR, and hypotension may occur if the patient is volume depleted or has an elevated sympathetic imbalance. Dobutamine is used to treat cardiac dysfunction, as seen for example with congenital heart disease or myocarditis. Because it increases myocardial oxygen demand, it may predispose to arrhythmias.
Ephedrine
Ephedrine acts indirectly by enhancing the release of norepinephrine from sympathetic neurons. It increases heart rate and cardiac output with a variable increase in SVR. Ephedrine is used (0.2–0.3 mg/kg/dose) to treat hypotension related to administration of general or regional anesthesia.
Epinephrine
Epinephrine stimulates alpha, beta-1, and beta-2 receptors. It is useful in treating shock from all causes, and is the drug of choice to treat anaphylaxis. At low doses (0.05–0.1 μg/kg/min), epinephrine stimulates beta-1 receptors, and increases heart rate and inotropy. Beta-2 receptor stimulation causes relaxation of arterioles, which decreases SVR and diastolic blood pressure. Higher doses (0.1–0.3 μg/kg/min) cause activation of alpha receptors resulting in vasoconstriction with a resultant increase in SVR that may compromise blood flow to end organs. Epinephrine clearance rates in critically ill children are lower than clearance rates in healthy adults.
Isoproterenol
Isoproterenol is a nonselective beta-adrenergic agonist with low affinity for alpha-adrenergic receptors. It increases heart rate, enhances myocardial contractility, and decreases SVR, thus resulting in an increase in cardiac output. Isoproterenol may be used to treat hemodynamically significant bradycardia and has been used in infants after cardiac surgery to improve cardiac index.
Norepinephrine
Norepinephrine has potent alpha activity with some beta-1 activity and little beta-2 activity, thus increasing SVR. Norepinephrine improves perfusion in children with hypotension and a normal or elevated cardiac index that has not responded to volume resuscitation. Treatment with norepinephrine is beneficial in the setting of tachycardia, because it can increase SVR, arterial blood pressure, diastolic blood pressure, and urine flow without an increase in heart rate. The usual starting infusion dose is 0.05 to 0.1 μg/kg/min, which is then titrated to effect. Norepinephrine administration may improve blood pressure without improving perfusion. This is most commonly seen in children with a low cardiac index and stroke volume.
Phenylephrine
Phenylephrine is an alpha-adrenergic agonist used to treat hypotension when SVR is low. Phenylephrine increases blood pressure and causes a vagally mediated sinus bradycardia, which is less pronounced in children than adults. A typical starting dose of phenylephrine in children is 0.1 mcg/kg/min, titrated to effect.
Milrinone
Milrinone is a phosphodiesterase inhibitor that produces inotropy and vasodilatation (usually without tachycardia). It has been used in children to treat low-output states after cardiac surgery and in the management of shock when catecholamine infusions alone are unsuccessful. Children demonstrate a higher volume of distribution of milrinone and a more rapid clearance rate than adults. A loading dose of 50 to 75 μg/kg over 15 to 60 minutes is followed by a continuous infusion of 0.375–1 μg/kg/min, titrated to effect. Milrinone is associated with atrial or ventricular arrhythmias in adults but usually not in children. Children with renal dysfunction have decreased clearance and may develop hypotension due to accumulation of drug and low SVR.
Vasopressin
Vasopressin acts on vasopressin receptors to promote water reabsorption in the kidney and a direct vasoconstrictor on arterioles to increase SVR. Vasopressin can be used in settings of catecholamine-resistant hypotension (e.g., septic shock). Our usual infusion doses for catecholamine-resistant shock start at 12 milliunits/kg/h and we increase by 12 milliunits/kg/h every 10 to 15 minutes to a maximum of 240 milliunits/kg/h. Dosing of vasopressin is challenging because of the nonuniformity of units used between different institutions and publications.
Vasopressor Refractory Shock
For patients that are refractory to fluid resuscitation and vasoactive medications, providers should consider giving steroids for suspected adrenal insufficiency. Hydrocortisone is the steroid of choice giving both glucocorticoid and mineralocorticoid effects. The usual initial pediatric dose is 100 mg/m 2 bolus dose followed by 100 mg/m 2 /day divided every 4 hours. The use of extracorporeal membrane oxygenation (ECMO) should be considered for refractory shock.
Hepatic Failure
Hepatic failure results from necrosis of liver cells that leads to impairment of hepatic function and possibly, hepatic encephalopathy. It can occur as a primary process in a healthy person, as an exacerbation of a chronic liver disease, or as part of multiorgan system failure. Hepatic failure can be classified into three types based on the time interval between the onset of jaundice and the development of encephalopathy: hyperacute (0–7 days), acute (1–4 weeks), and subacute (4–12 weeks). Main causes of fulminant hepatic failure in children include viral hepatitis, drug-induced liver injury, and inherited metabolic diseases.
Clinical features of hepatic failure include jaundice, enlarged liver, and encephalopathy, in conjunction with abnormal biochemical data such as hypoalbuminemia, hyperbilirubinemia, hyperammonemia, lactic acidemia, hypoglycemia, and coagulopathy with prolongation of the prothrombin time. There is no specific therapy or antidote for acute hepatic injury, with the exception of early administration of N-acetylcysteine to treat acetaminophen toxicity and as a potential antiinflammatory agent. Diagnostic tests are necessary to determine etiology and eligibility for liver transplant.
Hepatic failure can adversely affect other organ systems. Encephalopathy may result from diminished hepatic synthesis of an unknown substance(s) needed for normal brain function or diminished hepatic metabolism of an unknown substance(s) that confers neurotoxicity or promote neural inhibition. As liver function deteriorates, hepatic encephalopathy worsens. Serum ammonia levels do not correlate with the severity of encephalopathy. Advanced encephalopathy is associated with severe cerebral edema and life-threatening cerebral herniation.
Acute renal failure develops in 30% to 50% of patients with acute liver failure (hepatorenal syndrome). Contributing factors include intravascular volume depletion caused by excessive diuresis or untreated gastrointestinal hemorrhage and nephrotoxic drug administration.
Hemorrhagic complications in liver failure result from inadequate synthesis of clotting factors, thrombocytopenia from disseminated intravascular coagulation (DIC), and splenic sequestration of platelets. Blood loss may occur from stress-induced gastritis or from esophageal or gastric varices that develop secondary to portal hypertension. Vitamin K administration augments production of factors II, VII, IX, and X. Fresh frozen plasma and/or cryoprecipitate is administered when patients are bleeding or before an invasive procedure.
Hypoxemia can develop in acute liver failure for several reasons. Failure of the damaged liver to clear vasodilating humoral substances can cause intrapulmonary shunting and ventilation/perfusion mismatch. Pulmonary edema results from low oncotic pressure and fluid overload associated with antidiuretic-like activity. Atelectasis can develop from massive ascites that impedes full respiratory excursion or hypoventilation caused by encephalopathy. Ascites develops as a result of increased hepatic vascular resistance, decreased oncotic pressure, and altered aldosterone secretion creating abdominal competition.
Patients with chronic liver failure develop a high cardiac output in conjunction with a low SVR, again because of vasodilating humoral substances. Oxygen delivery is increased, but oxygen consumption is decreased because of microcirculatory disturbances that lead to tissue hypoxia.
Orthotopic liver transplantation is the most important therapy for acute liver failure. There is no clear set of indications for liver transplantation in pediatric patients, but without hepatic transplantation, acute hepatic failure carries a poor prognosis with an 80% to 85% mortality rate. Death is usually caused by cerebral herniation from intracranial hypertension or septicemia. At present, some artificial liver support systems are used in clinical practice as a bridge to liver transplantation such as molecular adsorbent recirculation system (MARS) and continuous veno-venous hemodiafiltration (CVVHDF) with or without plasmapheresis.
Renal Failure
Acute renal failure is the sudden inability of the kidney to regulate fluid, electrolyte, and solute balance, which can occur with or without a change in urine volume. Causes of acute renal failure can be categorized into three categories: prerenal disease, intrinsic renal parenchymal damage, and postrenal disease.
The prerenal type is caused by inadequate perfusion as a result of systemic hypovolemia, poor cardiac output, or vascular obstruction. Most intrinsic renal failure is caused by ischemia, nephron damage from toxins, or inflammation. Postrenal renal failure is caused by obstruction of the urinary collecting system. When the obstruction occurs at the level of the bladder or urethra, renal function is more likely to be severely affected because both kidneys will be injured. Unilateral obstruction does not result in renal failure if the other kidney is healthy.
The diagnosis of acute renal failure requires a stepwise approach that includes analysis of urinary sediment: white cell casts suggest interstitial nephritis; red cell casts are found in glomerulonephritis; and heme-positive urine without red blood cells suggest myoglobinuria. Analysis of urine osmolality, urine creatinine (Cr) and electrolytes can also aid diagnosis. Calculation of the fractional excretion of sodium (FE Na ) will help distinguish prerenal from renal causes:
FENa=100×(UrineNa×Plasmacreatinine)(PlasmaNa×Urinecreatinine)

Full access? Get Clinical Tree
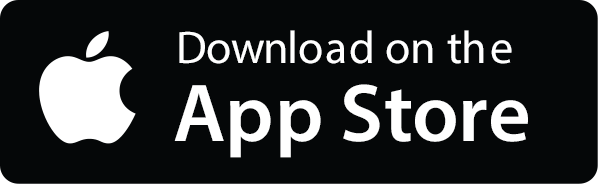
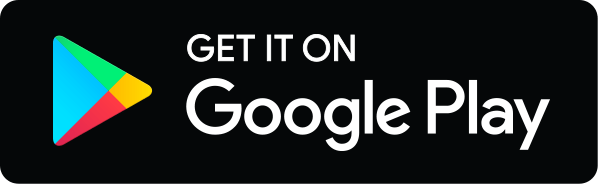
