FIGURE 15.1 Variation in body composition with age. Neonates have elevated total body water content relative to adults, but reduced fat and muscle stores.
b. Muscle mass is less than 20% in the preterm infant, about 30% in the term infant, and over 50% in an adult. Drugs that redistribute to muscle tissue will have less uptake in infants, and thus a swifter washout.
c. Fat content is <5% in a preterm infant, 10% in a term infant, and peaks at 20% to 30% in the first year of life before reaching normal adult levels of 15% to 20%. Since the neonate has less fat, a drug like thiopental that depends on initial redistribution into fat for termination of effect may have a higher plasma concentration and prolonged clinical effect.
2. Hepatic function. In infancy, a smaller proportion of cardiac output reaches the liver than in older children and adults. Hepatocellular enzymatic function is also immature. These factors combine to slow the hepatic metabolism of many drugs in neonates. Older children, on the other hand, usually have rapid drug elimination because of increased hepatic blood flow and robust enzymatic activity.
CLINICAL PEARL
Lower hepatic perfusion in neonates limits the delivery of drugs to the liver, prolonging the elimination half-life of all medications metabolized by the liver. Those drugs that do successfully reach the liver are metabolized slowly because of limited hepatocellular enzyme function. This means that drugs administered to these patients may have prolonged clinical effect or require reduced dosing.
a. Many drugs require biotransformation by the liver to more water-soluble forms before they can be eliminated. Phase I reactions transform drugs through oxidation, reduction, or hydrolysis. The cytochrome P450 system is a key contributor to phase I metabolism, and its various enzymes are involved in the transformation of many anesthetic drugs. Components of the cytochrome P450 system are at significantly reduced capacity in neonates relative to adults.
b. Phase II reactions transform drugs through conjugation. Impaired ability to complete phase II reactions can lead to prolonged elimination times for drugs that rely on hepatic conjugation before renal excretion. Slow phase II conjugation of morphine to morphine 3- and 6-glucuronide is partially responsible for the prolonged clinical activity of morphine in the neonate. Conjugation pathways do not reach full maturity until several months of age.
c. Plasma levels of albumin and α-1-acid glycoprotein are lower in neonates than in older infants and adults. The limited capacity for protein binding in neonates (and especially preterm neonates) results in a greater free portion of acidic drugs (e.g., thiopental) and basic drugs (e.g., local anesthetics).
CLINICAL PEARL
Reduced protein binding in neonates results in increased free fractions of drugs, swifter clinical onset, and increased danger for toxicity. Certain drugs, including barbiturates and local anesthetics, may require dose reduction.
3. Renal function. Glomerular and tubular functions are immature at birth, and perfusion pressure to the kidneys is lower in infants than in adults because of increased renal vascular resistance. Together, these physiologic differences mean that glomerular filtration in the term infant is less than one-third of adult levels when indexed to size, and is almost nonexistent in the extremely premature infant. Glomerular filtration rates develop rapidly, and approach adult levels by 1 year of age, with full maturation of renal function by the age of 2 years.
CLINICAL PEARL
Reduced renal perfusion and immature glomerular function mean that very young children cannot readily excrete excess fluid and solutes through glomerular filtration. Immature tubular function impairs urine concentrating and diluting abilities. Limited modification of the glomerular filtrate can lead to obligate sodium losses, which cause hyponatremia if not balanced by appropriate intake.
a. The half-life of medications excreted by the kidneys will be prolonged. Morphine 6-glucuronide, an active metabolite of morphine, is dependent on renal elimination and thus its accumulation will lead to prolonged opiate effect in infants with immature renal function.
CLINICAL PEARL
Morphine has prolonged clinical effect in neonates for two reasons: (i) Decreased hepatic perfusion and reduced conjugation enzymes result in slow metabolism of the native drug, and (ii) renal excretion of the active metabolite morphine 6-glucuronide is impaired. Fentanyl is usually a better choice in the neonatal population.
4. Minimum alveolar concentration. The minimum alveolar concentration (MAC) for most volatile agents increases from birth through early infancy, peaks at 3 to 6 months of age, and then gradually decreases throughout life (Fig. 15.2). The exception is sevoflurane, which actually has a slightly higher MAC in neonates than in infants 3 to 6 months old. The MAC for all agents is lower in preterm neonates than in term neonates.
Physiologic explanations for the lower anesthetic requirement of desflurane and isoflurane in neonates relative to older infants include: CNS immaturity, higher levels of endorphins, and the persistence of maternal progesterone. It is unknown why the MAC pattern of sevoflurane differs from that of the other agents.
CLINICAL PEARL
Children have elevated MAC requirements relative to adults, especially around 6 months of age. When choosing a maintenance dose, higher anesthetic requirements in children must be balanced against the deleterious effects of volatile anesthetics in neurosurgery, particularly increased CBF.
FIGURE 15.2 Minimum alveolar concentration (MAC) of common volatile anesthetic agents throughout childhood. For desflurane and isoflurane, MAC peaks at approximately 6 months of age. Sevoflurane differs from the pattern and has an elevated MAC in neonates. (Data from references 5–7.)
5. Anesthetic uptake. Inhalation induction is much faster in infants than in adults for several reasons (Table 15.1). The rapidity of anesthetic induction is determined by the rate of rise of the alveolar concentration of volatile agent relative to inspired concentration. Alveolar concentration is determined by the balance between anesthetic delivery and uptake.
Table 15.1 Causes of faster inhalation induction in children compared to adults
a. As in adults, volatile anesthetics cause a dose-dependent increase in CBF in children, and with swift anesthetic uptake it is easy to create rapid increases in CBF during inhalation induction.
II. Anesthetic management. A carefully planned anesthetic for pediatric neurosurgery starts with a thorough preoperative evaluation. Special attention should be paid to anything that might compromise airway access and to pre-existing comorbidities. Intraoperative management requires attention to maintenance agents, fluid and glucose administration, and thermoregulation.
A. Preoperative evaluation
1. Airway considerations. Airway assessment in a child is limited by patient cooperation, but at a minimum should include an evaluation of neck range of motion, mandibular size, and dentition. Unique features of the infant airway include large tongue-to-oral cavity ratio, cephalad positioning of the larynx, slanted vocal folds, and a narrow omega-shaped epiglottis.
CLINICAL PEARL
Infants have high minute ventilation-to-functional residual capacity (FRC) ratios, high metabolic demands for oxygen, and high closing capacities. Together, these features ensure that airway obstruction will result in swift desaturation and potentiation of neurologic injury.
When planning airway management, keep in mind that infants have high minute ventilation-to-FRC ratios, high oxygen demands, and high closing capacities. Together these factors ensure that airway obstruction will result in swift desaturation and possible secondary neurologic injury.
2. Neurologic disease. Children presenting for neurosurgery have many types of intracranial pathology, all with unique anesthetic considerations (Table 15.2). Knowledge of the type and extent of neurologic compromise is necessary before a child proceeds with corrective surgery. A preoperative neurologic examination should include assessment of level of consciousness, motor and sensory function, reflexes, and cranial nerve function. If symptoms of increased ICP are present, extra caution must be exercised during induction to avoid worsening the situation.
Table 15.2 Key elements of preoperative assessment for common neurosurgical diseases and their anesthetic considerations
a. In neonates and infants, intracranial hypertension may present with irritability, lethargy, refusal to feed, or bulging fontanelles.
b. In older children, signs of elevated ICP include headache, nausea or vomiting, confused speech, and depressed consciousness.
3. Other comorbidities. A thorough history and physical examination to uncover relevant comorbidities is essential. This is especially true in very young children, whose high risk of perioperative arrest may be partially related to failed recognition of coexisting congenital heart defects or airway syndromes [8].
4. Fasting status. Appropriate fasting intervals should be observed for elective surgery (Table 15.3). The guidelines published by the ASA are based on healthy patients undergoing elective surgery [9], so fasting intervals may need to be extended if there is reason to suspect delayed gastric emptying.
Table 15.3 Appropriate fasting intervals for children having elective surgery
CLINICAL PEARL
Gastrointestinal motility disorders, metabolic disorders (e.g., diabetes), fever, infection, trauma, pain, narcotic use, and anxiety can all delay gastric emptying. Children with nausea, vomiting, and intracranial hypertension may also require extended fasting intervals or a rapid sequence induction (RSI).
B. Premedication
1. Children with normal ICP may benefit from a sedative to reduce anxiety during the induction of anesthesia. The most popular choice is oral midazolam, although other medications and routes can be considered (Table 15.4).
Table 15.4 Choice of agents for premedication, with doses and routes of administration
CLINICAL PEARL
Sedatives are best avoided in children with symptoms of intracranial hypertension, as they can lead to respiratory depression, hypercarbia, hypoxia, and further elevation of ICP.
2. If the benefits of sedation are thought to outweigh the risks of further neurologic compromise, dexmedetomidine is an excellent choice because it has minimal effects on respiratory drive. There are studies to support the use of dexmedetomidine as an oral or nasal premedication [10,11].
3. In situations where pharmacologic anxiolysis is contraindicated, behavioral interventions may be helpful. Child life specialists can meet with the child preoperatively to explain the process of induction through visual aids, thereby building rapport and reducing anxiety. Clowns, video games, or low-stimulation environments during induction have also been shown to be helpful. Currently available evidence does not support the routine use of parental presence to reduce a child’s anxiety [12], but may be considered at the discretion of the anesthesiologist.
4. In children having epilepsy surgery with intraoperative seizure monitoring, midazolam is contraindicated because of its tendency to suppress seizure foci and interfere with electroencephalography. Dexmedetomidine is an acceptable alternative.
CLINICAL PEARL
In many situations in pediatric neurosurgery, premedication is avoided either because of the risk of respiratory depression and elevated ICP, or because of potential interference with neurologic examination and EEG monitoring. Anxious children with unsecured aneurysms are an important exception to this general principle. Sedatives should be given as needed in this population to prevent agitation, hypertension, and aneurysm rupture with anesthetic induction.
C. Induction techniques
1. In children with increased ICP, it is critical to prevent episodes of hypoventilation, hypoxemia, hypotension, coughing, or straining during induction of anesthesia.
2. IV techniques. Many children with intracranial lesions already have an IV in place, and proceeding with an IV induction can be a safe and simple option. A variety of induction agents can be used, including propofol, thiopental, and etomidate. Induction can be supplemented with a muscle relaxant, fentanyl (2 to 5 μg/kg), remifentanil (1 to 5 μg/kg), and/or lidocaine (1 to 1.5 mg/kg) to provide optimal intubating conditions.
a. With the exception of ketamine, all IV induction agents will cause a decrease in CBF and cerebral metabolic rate for oxygen (CMRO2).
CLINICAL PEARL
Ketamine should be avoided in children with intracranial hypertension because of its tendency to raise CBF and ICP.
b. Keep in mind that dosage requirements of commonly used anesthetic agents will vary with age. For example, the effective dose of propofol is 2 to 3 mg/kg in older children and adults, but increases to 3 to 5 mg/kg in infants.
FIGURE 15.3 Methods of anesthetic induction in a child who presents for neurosurgery without an intravenous line.

Full access? Get Clinical Tree
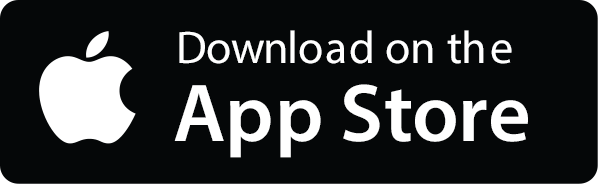
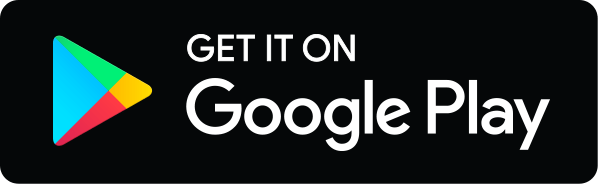