Chapter Outline
ANESTHESIA GAS DELIVERY SYSTEM
OXYGEN SUPPLY TO THE INTERMEDIATE-PRESSURE SYSTEM
FLOW PATHWAYS FOR OXYGEN IN THE INTERMEDIATE-PRESSURE SYSTEM
Auxiliary Diameter Index Safety System Oxygen Connector
Oxygen Supply Failure Alarm System
Pneumatically Powered Anesthesia Ventilator
Pressure Sensor Shut-off (Fail-Safe) Valves
ANESTHESIA MACHINE GAS PIPING SYSTEM
OXYGEN RATIO MONITORING AND PROPORTIONING SYSTEMS
ANESTHESIA MACHINE LOW-PRESSURE SYSTEM CHECKOUTS
Testing for Leaks in the Anesthesia Machine and Breathing System
Narkomed Machines: No Outlet Check Valve
Datex-Ohmeda Machines with an Outlet Check Valve
Datex-Ohmeda Machines Without an Outlet Check Valve
ANESTHESIA MACHINE ELECTRICAL SYSTEMS
Anesthesia Gas Delivery System
The modern anesthesia gas delivery system is composed of the anesthesia machine, anesthesia vaporizer(s), ventilator, breathing circuit, and waste gas scavenging system. The basic arrangement of these elements is the same in all contemporary anesthesia gas delivery systems ( Fig. 2-1 ). The anesthesia machine receives gases under pressure from their sources of storage (see Chapter 1 ), creates a gas mixture of known composition and flow rate, and delivers it to a concentration-calibrated vaporizer, which adds a controlled concentration of potent, inhaled, volatile anesthetic agent. The resulting mixture of oxygen—with or without a second gas such as nitrous oxide, air, or helium (heliox)—is delivered to the machine’s common gas outlet (CGO). This gas mixture flows continuously from the CGO into the patient breathing circuit. The breathing circuit represents a microenvironment in which the patient’s lungs effect gas exchange, so that by controlling the fresh gas mixure’s composition, flow rate, and ventilatory parameters, the patient’s arterial partial pressures of oxygen, nitrous oxide, anesthetic agent, and carbon dioxide can be controlled. Fresh gas flows continuously into the breathing system, most commonly a circle breathing system. Gas must therefore be able to leave the circuit, otherwise the pressure would increase and possibly lead to barotrauma—unless a completely closed system is used. If ventilation is spontaneous or assisted, excess gas leaves the circuit via the adjustable pressure-limiting (APL) valve. If the lungs are mechanically ventilated, the ventilator bellows or piston acts as a counterlung, exchanging its volume with the patient’s lungs via the breathing circuit. In this case, excess gas exits the breathing circuit at end expiration via the ventilator pressure relief valve. The gas that exits the circuit is waste gas that enters the waste gas scavenging system to be discharged outside the facility. An understanding of the structure and function of the gas delivery system is essential to the safe practice of anesthesia.

Anesthesia Machine and Workstation
Gas delivery systems continue to evolve as advances in technology and safety are incorporated into current designs. The recent evolution can be traced through the voluntary consensus standards that have been developed with input from manufacturers, users, and other interested agencies. The current voluntary consensus standard that describes the features of a contemporary system is published by the American Society for Testing and Materials (ASTM). This document, standard F1850-00, published in March 2000 and reapproved in 2005, is entitled Standard Specification for Particular Requirements for Anesthesia Workstations and Their Components . It introduces the term “workstation” in distinction to “anesthesia (or gas) machine.” The anesthesia workstation is defined as a system for the administration of anesthesia to patients consistsing of the anesthesia gas supply device (i.e., the machine), ventilator, and monitoring and protection devices. This standard supersedes anesthesia machine standard F1161-88, published in 1989 by the ASTM. Standard F1161-88 had superseded the original anesthesia machine standard (Z79.8-1979) published in 1979 by the American National Standards Institute (ANSI). Like its predecessors, ASTM standard F1850-00 has been voluntarily adopted by anesthesia machine manufacturers. The standard is not mandated, but it is highly unlikely that a new manufacturer would build, or would a purchaser be likely to purchase, a workstation that did not comply with the current standards.
The evolution of the anesthesia workstation and advances in technology have led to many changes in design. Although basic operations remain the same, the components are more technologically advanced. For example, in many new models—such as the Dräger Apollo ( Fig. 2-2 ) and the GE Aisys ( Fig. 2-3 )—the familiar rotameter tubes are replaced by virtual flowmeters displayed on a computer screen. The gas flow-control needle valves may be replaced by electronically controlled gas-mixing devices. This chapter describes the basic components and functions of a traditional anesthesia machine (i.e., the gas delivery device described in standard F1850-00) to enable the reader to appreciate some of the changes that have been made in the most recent models.


In the United States, the two largest manufacturers of gas delivery systems—machines, ventilators, vaporizers, and scavenging systems—are Draeger Medical Inc. (Telford, PA) and GE Healthcare (Waukesha, WI). This chapter reviews the features of a basic anesthesia delivery system, making reference to Dräger and GE (Datex-Ohmeda brand) products when appropriate. The flow of compressed gases from the point of entry into the machine, through the various components, and to the exit at the CGO is described. The function of each component is discussed so that the effects of failure of that component, as well as the rationale for the various machine checkout procedures, can be appreciated. This approach provides a framework from which to diagnose problems that arise with the machine. Of note, the individual machine or workstation manufacturer’s operator and service manuals represent the most comprehensive reference for any specific model of machine, and the reader is strongly encouraged to review the relevant manuals. The manufacturers also produce excellent educational materials, and a number of simulations are also available on the Internet.
Basic Anesthesia Machine
Although older anesthesia machines were completely pneumatic and required only a supply of gas under pressure, contemporary machines are electronic and pneumatic and therefore must be connected to an electrical outlet for normal, uninterrupted operation. When the main ON/OFF switch is turned on, both the pneumatic and the electronic functions are enabled.
In the OFF position, most of the electronic functions of the workstation are disabled, with the exception of the battery charger that charges the backup battery and the convenience electrical outlets on the back of the workstation, which are used to supply power to additional monitors (e.g., bispectral index) or a heated, pressurized desflurane vaporizer. The pneumatic functions maintained are the oxygen supply to the oxygen flush system and, in most machines, the auxiliary oxygen flowmeter.
When the ON/OFF switch is turned to ON, the workstation electronics go through a powering-up protocol that may include an automated checkout procedure that lasts several minutes. In case of an emergency, the automated checkout can be bypassed, but a record of this is maintained in the workstation’s computer. In the ON position, the pneumatic functions permit delivery of an anesthetic gas mixture from the flowmeters and the concentration-calibrated vaporizer.
Pneumatic Systems
The gas flow arrangements of a basic two-gas anesthesia machine are shown in Figure 2-4 . The machine receives each of the two basic gases, oxygen (O 2 ) and nitrous oxide (N 2 O), from two supply sources: a tank or cylinder source and a pipeline source. The storage and supply of these gases to the operating room (OR) is described in Chapter 1 .

The basic functions of any anesthesia machine are to receive compressed gases from their supplies and to create a gas mixture of known composition and flow rate at the CGO. The relation between pressure and flow is stated in Ohm’s law:

Controlling the flow of gases from high-pressure sources through the machine to exit the CGO at pressures approximating atmospheric pressure requires changes in pressure and/or resistance. Modern anesthesia machines also incorporate certain safety features designed to prevent the delivery of a hypoxic mixture to the patient circuit. These features include the oxygen supply pressure failure alarm, pressure sensor shut-off (“fail-safe”) system, and gas flow proportioning systems.
The anesthesia machine gas pathways have been conveniently divided by some authors into three systems :
- 1.
A high-pressure system that includes parts upstream of the first-stage regulator, where oxygen pressures are between 45 and 2200 psig
- 2.
An intermediate pressure system that includes parts between the pipeline gas inlet/downstream outlet of the first-stage pressure regulator and the gas flow control valves, where oxygen pressures are between 55 and 16 psig
- 3.
A low-pressure system that includes all parts downstream of the gas flow control valves, where pressures are normally slightly greater than atmospheric pressure
Other authors consider the high-pressure system to be simply all parts upstream of the gas flow control valves and the low-pressure system to be all parts downstream of the gas flow control valves. Indeed, this is in agreement with the system descriptions in the U.S. Food and Drug Administration 1993 preuse checkout recommendations. Either way, the most important definition is that of the low-pressure system, the one to which most preuse checkouts refer.
Oxygen Supply Sources: Pipelines and Cylinders
Pipeline oxygen is supplied to the wall outlets in the OR at a pressure of 50 to 55 psig. Gauge pressure is pressure above ambient atmospheric pressure. Ambient atmospheric pressure at sea level is usually considered to be equivalent to 760 mm Hg, or 14.7 psia. The wall outlet connectors are gas specific, but only within each manufacturer’s connector system. Thus an Ohmeda oxygen hose connector will not fit into an Ohmeda nitrous oxide wall outlet, but neither will it fit into an oxygen wall outlet in a Chemtron system. Thus the medical gas wall outlets and connectors are not interchangeable among the various gases or with the vacuum ( Fig. 2-5 ). A color-coded hose conducts the pipeline oxygen from the wall outlet to the anesthesia machine’s oxygen inlet. At the machine end of the hose, the connectors are gas specific by a national standard ( Fig. 2-6 ) known as the diameter index safety system (DISS) that ensures that the correct gas enters the correct part of the anesthesia machine. To standardize connections, many institutions are replacing the manufacturer’s gas-specific wall outlets with DISS connectors ( Fig. 2-7 ).



The machine’s oxygen pipeline inlet incorporates a check valve that prevents leakage of oxygen from the machine if the pipeline is not connected and oxygen tanks are in use ( Fig. 2-8 ). Failure of this valve would cause oxygen to leak from the machine. Upstream of the pipeline inlet in the machine is a pressure gauge that displays the pipeline gas supply pressure (see Fig. 2-4 ).

Tank oxygen is supplied to the machine from the back-up E-cylinders attached via the oxygen hanger yokes ( Fig. 2-9 ). The medical gas pin index safety system ensures that only an oxygen tank fits correctly into an oxygen hanger yoke (see Chapter 1 ). Although manipulation is possible, a medical gas cylinder should never be forced to fit into a hanger yoke.

The pressure in a full oxygen tank is normally between 1900 and 2200 psig. Oxygen enters the hanger yoke at this pressure and then passes through a strainer nipple ( Fig. 2-10 ) designed to prevent dirt or other particles from entering the machine. The oxygen then flows past a hanger yoke (“floating”) check valve to enter the anesthesia machine at high pressure.

Several considerations apply before a tank is hung in a yoke. First, the plastic wrapper that surrounds the tank valve must be removed. Then the valve is opened slowly, or “cracked,” to allow gas to exit the tank and blow out any particles of dirt that may be lodged in the outlet. The tank is then hung in the yoke. The gas outlet hole is aligned with the strainer nipple, and the two yoke pins are aligned with the corresponding holes in the tank. The tank should never be turned through 180 degrees and then hung in the yoke because a tightened T-handle screw might damage the tank valve-stem pressure relief mechanism (see Chapter 1 ). Although changing the oxygen tank on an anesthesia machine may seem straightforward, one study showed that a significant number of senior residents in a simulator could not perform this task satisfactorily, possibly because it is generally performed by technical staff. Checking to see that a backup tank contains sufficient oxygen is an important part of the preuse checkout and also ensures that a tank wrench is available for opening and closing the tank valve.
Oxygen is also available in freestanding E-size tanks pressurized to 3000 psig (1000 L gaseous oxygen) that incorporate a regulator that delivers oxygen at 50 psig to a DISS connector. Thus, if the pipeline fails, the machine’s oxygen hose could be connected to this tank outlet if the wall end of the hose has a DISS fitting ( Figs. 2-11 and 2-12 ).


Some anesthesia machines have two hanger yokes for oxygen. Once oxygen has passed through the check valve, the two hanger yokes are connected by high-pressure tubing, to which an oxygen pressure gauge is also connected (see Fig. 2-16 ). This gauge measures the pressure of the oxygen cylinder supply connected via hanger yokes. On many machines, both yokes may share one pressure gauge. In this case, to measure the tank pressure, the pipeline supply is first disconnected from the machine and both tanks are turned off. Next, the oxygen flush button is depressed to drain all oxygen from the machine, and the tank and pipeline gauge readings should both fall to zero. One tank is then turned on, and its pressure is noted on the gauge. The tank is then turned off, the oxygen flush button is again depressed, and the tank gauge pressure falls to zero. The second tank is then opened and its pressure noted. The second tank is then closed, and the oxygen flush button is depressed. The pipeline connector is then reattached to the wall oxygen outlet.

As previously noted, a check valve in each oxygen hanger yoke is designed to prevent oxygen from flowing out of the machine through the strainer nipple. This valve prevents loss of gas via the hanger yoke when no oxygen tank is hanging in one yoke, but an oxygen tank in the other yoke is being used to supply the machine (see Fig. 2-16 ). These valves also prevent transfilling of one oxygen tank to the other, if two tanks are hanging on the machine and both are on. In other words, without a check valve, oxygen would tend to flow from the full tank to the empty one if both were open. If the check valve were not present, the transfilling and sudden compression of oxygen into the empty cylinder could cause a rapid temperature rise in the pipes, gauge, and tank with an associated risk of fire. This is known as an adiabatic change, in which the state of a gas is altered without the gas being permitted to exchange heat energy with its surroundings.
If there are two hanger yokes for oxygen but only one tank is hanging, a yoke plug should be inserted and tightened in the empty yoke. Thus, if an oxygen check valve leaks, loss of oxygen from the empty hanger yoke is prevented by the yoke plug ( Fig. 2-13 ).

The pressure gauges used in the traditional machine to measure pipeline supply pressure or tank supply pressure ( Fig. 2-14 ) are of the Bourdon tube design. In principle, the Bourdon tube is a coiled metal tube sealed at its inner end and open to the gas pressure at its outer end (see Chapter 9 ). As gas pressure rises, the coiled tube tends to straighten. A pointer attached to the inner-sealed end thereby moves across a scale calibrated in units of pressure. If the Bourdon tube were to burst, the inside of the gauge could be exposed to high pressure. The gauge is therefore constructed with a special heavy glass window and a mechanism designed to act as a pressure fuse so that gas is released from the back of the casing if the pressure suddenly rises. The cylinder and pipeline pressure gauges for the gases supplied to the machine are generally situated in a panel on the front of the anesthesia machine (see Fig. 2-14 ). In some workstations, pressure is sensed by a pressure transducer and is displayed on a screen ( Fig. 2-15 ).


Cylinder Pressure Regulator
A pressure regulator is a device that converts a variable, high-input gas pressure to a constant, lower output pressure. As previously mentioned, tank oxygen enters the machine at pressures of up to 2200 psig depending on how full the tank is. These variable, high-input pressures are reduced to a constant, lower output pressure of 45 psig by the oxygen cylinder pressure regulator, sometimes termed the first-stage regulator (see Fig. 2-4 ). As noted in Figure 2-16 , the tank oxygen from both yokes flows to a common pathway leading to the inlet of the regulator. One regulator serves the two oxygen hanger yokes and is located under the machine’s work surface.
The principles of action of the regulator are shown in Figure 2-17 . This is described as a direct-acting regulator because the high-pressure gas tends to open the valve. In an indirect-acting regulator, the high-pressure gas tends to close the valve. In essence, the regulator works by balancing the force of a spring against the forces that result from gas pressures acting on a diaphragm. Oxygen at tank pressure enters the high-pressure inlet and is applied over a small area to the valve seat (see Fig. 2-17 ), and the valve opening is opposed by a return spring. The valve seat is connected by a thrust pin to a diaphragm in the low-pressure chamber of the regulator. Upward movement of the diaphragm is opposed by a spring that exerts a pressure of 45 psig on the diaphragm. The adjustment of this spring is such that oxygen may flow from the high-pressure inlet across the valve seat and into the low-pressure chamber. If pressure in the low-pressure chamber exceeds 45 psig, the diaphragm moves upward and closes the valve opening, halting the flow of oxygen from high- to low-pressure chambers, until the gas pressure exerted on the diaphragm falls below 45 psig. The pressure in the low-pressure chamber and the low-pressure piping of the machine when supplied by the tanks is thereby kept at a constant 45 psig. A cessation of flow from the low-pressure chamber, such as would occur if the oxygen flow control valve meter were closed, causes pressure to build up here, closing the regulator valve and halting the flow of gas from the cylinder into the regulator.


Failure of the pressure reduction function of a regulator can transmit excessively high pressure (up to 2200 psig) to the machine’s low-pressure system (see Fig. 2-17 ). To protect against such occurrences, the regulator incorporates a pressure relief valve in the low-pressure chamber in which excess pressures are vented to the atmosphere. If the diaphragm were to rupture or develop a hole, the regulator would fail and gas would escape around the adjustment screw and spring. The high flow of escaping oxygen makes a loud sound, alerting the anesthesiologist to the possibility of a regulator failure. Such a hole represents a significant leak in the high-pressure system or intermediate-pressure system through which oxygen would be lost. Figure 2-4 shows that even if the tanks were turned off and the pipeline supply were in use, a ruptured diaphragm in the regulator would cause loss of oxygen from the machine’s high-pressure system or intermediate-pressure system and a possible failure of oxygen supply to the flowmeters. Such a machine should be withdrawn from service until the problem has been corrected by an authorized service technician. Meanwhile, oxygen may be supplied to the patient by a self-inflating (Ambu) bag connected to a portable supply of oxygen, such as a transport oxygen cylinder with its own pressure-reducing valve and flowmeter.
Oxygen Supply to the Intermediate-Pressure System
Oxygen is typically supplied to the machine’s pipeline connector inlet at pressures of 50 to 55 psig, whereas the tank oxygen supply is regulated to enter at 40 to 45 psig. This difference in supply pressures is deliberate; if the pipeline is connected and the oxygen tanks are open, oxygen is preferentially drawn from the pipeline supply. This is because the higher pressure (50 to 55 psig) from the pipeline supply closes the valve in the first-stage oxygen regulator, thereby preventing the flow of oxygen from the tank. However, at times, such as during heavy oxygen use, the pipeline pressure may fall below 45 psig. In this case, oxygen would be drawn from the tanks if they were open. Thus, once the tank supply has been checked, it should be turned off to prevent loss of the backup oxygen supply. Also, if the tank is left open, oxygen might leak around the plastic washer between the tank and the yoke.
An awareness of the differential in supply pressures of oxygen to the machine is essential. If a pipeline crossover is suspected, such as when a hypoxic gas is flowing through the oxygen pipeline to the piping in the machine, the machine must be disconnected from the pipeline supply if the backup oxygen supply is to be used. For example, if a hypoxic gas (e.g., nitrous oxide) is accidentally used to supply the oxygen pipeline at a supply pressure of greater than 45 psig, the anesthesiologist cannot deliver the true oxygen from the backup tanks because the pressure from the wall supply is greater than that from the first-stage oxygen regulator.
Flow Pathways For Oxygen in the Intermediate-Pressure System
Having entered the machine intermediate-pressure system at 50 to 55 psig (pipeline) or 40 to 45 psig (tank first-stage regulator), oxygen can flow or pressurize in several directions.
Oxygen Flush
As soon as any oxygen supply is connected to the machine, pressing the oxygen flush button results in a flow of oxygen to the machine CGO at 35 to 75 L/min. Figure 2-18 shows that this pathway bypasses the main pneumatic and electronic ON/OFF switches and that the pressure at the CGO could increase the supply pressure to the machine unless some pressure relief mechanism is present. To avoid barotrauma in a patient, extreme caution is therefore necessary when oxygen flush is used. Contemporary machines incorporate a pressure-limiting device to prevent such potentially harmful pressures, particularly if the flush is activated during the inspiratory phase of positive-pressure ventilation (see Chapter 6 ).

The workstation standard requires that the oxygen flush valve be self-closing and designed to minimize unintended operation by equipment or personnel. A modern design for an oxygen flush button is shown in Figure 2-19 ; note that the button is recessed in a housing to prevent accidental depression and that the valve is self-closing.


Auxiliary Oxygen Flowmeter
Most contemporary machines incorporate an auxiliary oxygen flowmeter that delivers oxygen, usually via a pressure-reducing regulator, to an accessible nipple at flows typically up to 10 L/min ( Fig. 2-20 ). This is the source used to connect devices that deliver supplemental oxygen to a nasal cannula, face mask, or self-inflating reservoir bag, such as an Ambu bag. Similar to the oxygen flush, this flowmeter is active when the machine’s main ON/OFF switch is off.

Auxiliary Diameter Index Safety System Oxygen Source
Many workstations provide an auxiliary source of oxygen at pipeline pressure (50 to 55 psig) via a DISS connector while the machine is connected to a pipeline supply. An available DISS connector can be beneficial if the machine’s oxygen hose and the wall oxygen outlets have quick-connect fittings ( Figs. 2-20 and 2-21 ). The outlet can be used to drive a Sanders-type jet ventilator, a Venturi-based suctioning device, or other device that requires pipeline oxygen pressure.

Unless otherwise stated, oxygen flows to, or pressurizes, the components that follow it only when the main ON/OFF switch is in the ON position (see Fig. 2-4 ).
Oxygen Supply Failure Alarm System
Oxygen pressurizes an oxygen supply failure alarm system such that if the supply pressure falls, usually below 30 psig, an alarm is triggered (see Figs. 2-4 and 2-18 ). Some older machine models use a canister pressurized with oxygen that emits an audible alarm for at least 7 seconds when the pressure falls below the threshold. Contemporary machines use a pressure-operated electrical switch that ensures a continuous audible alarm when the oxygen supply pressure falls below the threshold setting.
The workstation standard requires that whenever oxygen supply pressure falls below the manufacturer-specified threshold, a medium-priority alarm is activated within 5 seconds. After the alarm has been activated, it may be released by the user for a period of up to 120 seconds but is automatically reset after restoration of oxygen supply pressure to a level above the alarm threshold.
Pneumatically Powered Anesthesia Ventilator
Oxygen at a pressure of 50 to 55 psig (pipeline) or 45 psig (tanks) is used as the power source for pneumatically driven anesthesia ventilators, such as the GE Datex-Ohmeda 7000, 7800, and 7900 series and the Dräger AV-E. In Datex-Ohmeda machines, when the ventilator connection is made, the valve opens to permit compressed oxygen to flow to the ventilator ( Fig. 2-22 ). In Dräger Narkomed 2, 3, and 4 machines, the oxygen takeoff to drive the ventilator is downstream of the machine main ON/OFF switch, so the ventilator cannot be operated if the machine is turned off (see Fig. 2-4 ). In older model Ohmeda machines, the oxygen takeoff to the ventilator circuit is upstream of the main ON/OFF switch.

During operation, pneumatically powered ventilators consume large quantities of oxygen. If the machine is being supplied by a backup E-cylinder, that cylinder’s contents are rapidly exhausted because it is also supplying oxygen to the patient circuit. In ventilators that use 100% oxygen as the driving gas—such as the Datex-Ohmeda 7000, 7800, and 7900 series—the ventilator oxygen consumption is in excess of the minute ventilation set on the ventilator (i.e., Rate × Bellows tidal volume). The Dräger AV-E is more economical in terms of oxygen because it uses oxygen to drive a Venturi that entrains air. The air-oxygen mixture is then used as the final driving gas mixture that enters the ventilator bellows housing (see Chapter 6 ).
In some workstations, if the pressurized supply of oxygen is lost but air is not, the ventilator may switch to being powered by compressed air ( Fig. 2-23 ). Of course, this is not an issue in workstations that use a piston ventilator powered by an electric motor.

Pressure Sensor Shut-off (Fail-Safe) Valves
When the main ON/OFF switch is in the ON position, oxygen pressurizes and holds open a pressure-sensor shut-off valve. These valves reduce or interrupt the supply of nitrous oxide and other hypoxic gases (e.g., carbon dioxide and helium), but not air, to their flowmeters if the oxygen supply pressure falls below the threshold setting. This valve, in relation to control of the nitrous oxide supply, is the fail-safe system designed to prevent the unintentional delivery of a hypoxic mixture from the flowmeters.
The action of turning the machine’s main ON/OFF switch to the OFF position allows the oxygen pressure in parts of the machine downstream of the switch (normally 45 to 55 psig) to be vented to the atmosphere. The resulting decrease in oxygen pressure causes the fail-safe valves to interrupt the supply of all other gases, usually with the exception of air, to their flow-control valves. The design of the fail-safe system differs between the Dräger and Datex-Ohmeda machines.
In older model Datex-Ohmeda machines, when the oxygen supply pressure in the intermediate-pressure system falls below 20 to 25 psig, the flow of nitrous oxide to its flowmeters is completely interrupted. The pressure sensor shut-off valve used by the older model Datex-Ohmeda machines is an all-or-nothing threshold arrangement—open at oxygen pressures greater than 20 to 25 psig and closed at pressures below 20 psig ( Fig. 2-24 ).


In the Datex-Ohmeda Aestiva/5 workstation, a more recent model, the fail-safe valve is not an all-or-nothing design; it is a variable valve in a balance regulator, in which the secondary regulator for oxygen reduces the pressure to approximately 30 psig in the intermediate-pressure system (see Fig. 2-4 ). The oxygen pressure is then piloted to the balance regulator, where it is applied to the oxygen side of the regulated diaphragm. If the pressure of oxygen is sufficient, the diaphragm pushes against a mechanism that opens the flow pathway for nitrous oxide. If the oxygen piloting pressure decreases, the mechanism begins to close off the pathway for nitrous oxide in proportion to the decrease in piloted oxygen pressure. The balance regulator for nitrous oxide completely closes when the pressure of oxygen falls to 0.5 psig. Balance regulators for heliox and carbon dioxide interrupt the flow of these gases when the piloted oxygen pressure falls below 10 psig.
The fail-safe valve in Narkomed 2, 3, and 4 machines is called an oxygen failure protection device (OFPD) and, as in the Datex-Ohmeda systems, there is one for each of the gases supplied to the machine ( Fig. 2-25 ). As the oxygen supply pressure falls and the flow of oxygen from the machine’s flowmeter decreases, the OFPDs proportionately reduce the supply pressure of other gases to their flowmeters. The supply of nitrous oxide and other gases is thereby completely interrupted when the oxygen supply pressure falls to below 12 ± 4 psig. Therefore, the OFPD functions similarly to the balance regulator in the Datex-Ohmeda Aestiva/5.

Both fail-safe valve designs ensure that at low- or zero-oxygen supply pressures, only oxygen may be delivered to the machine’s CGO. However, as long as the oxygen supply pressure is adequate , other gases may flow to their flowmeters. The fail-safe system does not ensure oxygen flow at its flowmeter, only a supply pressure to the oxygen flowmeter. Thus a normally functioning fail-safe system would permit flow of 100% nitrous oxide, provided the machine has an adequate oxygen supply pressure. The term “fail-safe” therefore represents something of a misnomer because it does not ensure oxygen flow ( Fig. 2-26 ).

Flowmeters
Oxygen flows to the oxygen flow control valve and flowmeter(s), traditionally rotameters. Supply pressure to the oxygen flowmeters differs in Datex-Ohmeda and Dräger machines. In contemporary Datex-Ohmeda machines, the oxygen supply pressure to the flowmeters is regulated to a constant, lower pressure—14 to 30 psig, depending upon the model—by a second-stage regulator. This regulator (see Figs. 2-4 and 2-18 ) ensures a constant supply pressure to the Datex-Ohmeda oxygen flowmeter. Thus, even if the oxygen supply pressure to the machine decreases below 45 to 50 psig, as long as it exceeds the set second-stage regulated downstream pressure, the flow setting on the oxygen flowmeter is maintained. Without this second-stage regulator, if the oxygen supply pressure were to fall, the oxygen flow at the flowmeter would decrease. If another gas (e.g., nitrous oxide) was also being used, a hypoxic gas mixture could result at the flowmeter manifold.
The second-stage oxygen regulator used in Datex-Ohmeda machines is similar in terms of principle of operation to that of the first-stage regulator (see Fig. 2-17 ). However, because it normally handles lower pressures than the first-stage regulator, it neither requires nor incorporates a pressure relief valve.
Narkomed anesthesia machines do not use a second-stage oxygen pressure-regulator valve (see Fig. 2-4 ). These machines have OFPDs that interface the supply pressure of oxygen with that of nitrous oxide and the other gases supplied to the machine. The OFPD consists of a seat-nozzle assembly connected to a spring-loaded piston (see Fig. 2-25 ). When deactivated, the spring is expanded, forcing the nozzle against the seat so that no gas can flow through the device to the flowmeter. As oxygen pressure increases, it is applied to the piston, which in turn forces the nozzle away from its seat so that gas can flow through the OFPD. The OFPD responds to oxygen pressure changes such that as pressure falls, the other gas flows will fall in proportion. When the oxygen supply pressure is less than 12 ± 4 psig, the OFPD is completely closed. A decrease in oxygen supply pressure causes a proportionate decrease in the supply pressures of each of the other gases to their flowmeters. As the oxygen supply pressure and flow decrease, all other gas flows are decreased in proportion to prevent the creation of a hypoxic gas mixture at the flowmeter level (see the preceding section). The operation of the OFPD can be demonstrated. With the Narkomed machine supplied from pipeline oxygen (55 psig), set 6 L/min flows of both nitrous oxide and oxygen; if the pipeline oxygen is disconnected and the tank oxygen supply is opened (45 psig), flow of both oxygen and nitrous oxide will be observed to have decreased at the rotameters.
The use (GE Datex-Ohmeda) or nonuse (Dräger) of a second-stage oxygen regulator affects the total gas flow emerging from the CGO of the machine if the oxygen supply pressure falls. In an older model Datex-Ohmeda machine, as long as the oxygen supply pressure exceeds the set threshold of the second-stage regulator, all gas flows are maintained at the original flowmeter settings. In a Narkomed machine, if the oxygen supply pressure falls from normal (45 to 55 psig), all gas flows decrease in proportion via the OFPDs. A decrease in total gas flow from the machine’s CGO might result in rebreathing, depending on the breathing circuit in use.

Full access? Get Clinical Tree
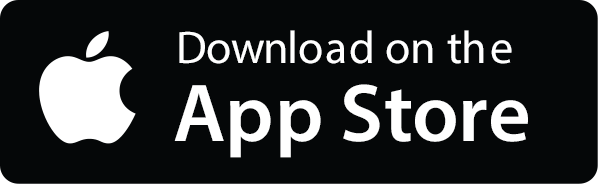
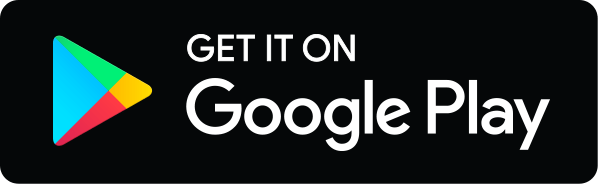