Chapter 4 The anaesthetic workstation
The anaesthetic workstation (Fig. 4.1) has evolved over the years from the simple inhaler for the admixture of volatile anaesthetics, through anaesthetic machines with calibrated delivery of inspired agents to the current modern workstation complete with integral ventilator, breathing system and patient and machine monitoring. Automated record keeping systems allow integration of the machine into institutional information networks and, more recently, online access to expert systems for decision support (see Chapter 22, Information Technology and the Anaesthetic Workstation). Such systems remain rare and in spite of the diminishing costs and expanding capabilities of electronic-microprocessor-based control systems, simple pneumatic anaesthetic machines with needle-valve flowmeters and conventional vaporizers still dominate in UK hospitals. There has been no ‘revolution’ in anaesthetic machines as yet.
Products offered to a market place by successful companies reflect a balance of technology, economics and what the end user is or will be willing to engage with. In this respect the particular machines described in the latter section of this chapter when viewed as a whole, demonstrate how anaesthetists have only gradually come to be prepared to use ‘fly by wire’ technology: electronically controlled machines operated through a computer interface. Even within anaesthesia such technology is not new; along the way machines with iterations of such technology such as the Engstrom Elsa1 have perished in the market where the devices really were ‘before their time’.
When the anaesthetic machine appears a physically, if not intellectually, impenetrable box with opaque control systems operated through a simple video screen, it is tempting to think that the study of joints in metal tubing, pressure regulators, mechanical hypoxia prevention systems and flow valves is somewhat anachronistic. It may be, but caution is advised: the user manual for a state of the art workstation such as the Dräger Zeus runs to 346 pages (it is difficult to envisage more than a singular instruction sheet for the original Boyle’s trolley) and reflects the complexity of the processes underlying these devices. The logic and control of such systems is an elaboration of what has gone before and an understanding of the processes is necessary if we are to be able to cope with the machines and to demand better performance or deal with fault conditions. Additionally it seems we are occasionally required to relearn lessons of the past.2
Throughout the changes above, developments have been driven by the need for safety addressed by the inclusion of fail-safe systems, and by ‘the designing out’ of the possibilities of dangerous user errors. This aspect of the workstation may be regarded as having developed through successive generations of improvements and modifications having been incorporated, such that the ‘anaesthetic machine’ component of a modern workstation is still usually recognizable as a descendant of the first-generation Boyle’s trolley. Over time, technological safety features have become enshrined in national and international standards which are largely adhered to. As such, an analysis of critical incidents may help to inform a logical approach to the understanding of the safety features and design of modern machines, and for this reason such a section is included in this chapter.
One notable change over the last few years has been the narrowing gap between anaesthesia ventilators and intensive care ventilators. Software-driven, electronically controlled devices are seen that, if not identical in technology to the ITU types, are at least capable of emulating most ventilatory modes if desired. Simple purely mechanical ventilators are seen now only in devices purpose built for the developing countries (see Chapter 27). The reader is referred to Chapters 9 and 10 for more detailed information on ventilators.
Functions of the modern workstation
• Safe provision, selection and delivery of anaesthetic gasses and vapours together with an appropriate built-in breathing system, usually a circle system
• Provision of back-up supplies of gasses in the event of failure of the primary sources
• Respiratory support in the form of sophisticated automatic ventilators capable of managing the full range of patient needs
• Monitoring of machine function and settings
• Monitoring of patient physiological variables
• Automated archived record keeping (or at least the facility to output monitored parameters to other systems) and ideally on-line access to information and administration networks
• The integration of the display and auditory signalling of monitored modalities
• Provision of appropriate connection to an anaesthetic gas scavenging system
• Provision of medical vacuum via a suction regulator
• Provision of supplemental oxygen using an auxiliary O2 flowmeter (for reduced risk of inadvertent anaesthesia)
• Work surface and storage facility for ‘everyday’ items
• Provision of mains electricity sockets for low-consumption items used in association with delivery or monitoring of anaesthesia, e.g. syringe pumps. (international standards preclude the powering of one medical device from another. For the purpose of this standard, the workstation is seen as a resuscitation trolley.)
Development of the anaesthetic workstation
Integrated and modular designs
• a circle breathing system and usually a ‘bag in bottle’ arrangement to separate respiratory gasses from the usually pneumatically driven ventilator (see Dräger Zeus for an alternative configuration)
• a choice of ventilator, often a single design but software driven and available in a variety of software configurations
• usually one of a choice of back bar vaporizer attachment systems, i.e. Selectatec or Dräger, allowing vaporizers to be exchanged for a choice of volatile agent; however, some newer electronically controlled machines utilize their own proprietary systems for volatile agent dosing (see later)
• a patient monitor of choice, itself often of a modular design where individual parameters can be ‘hot swapped’, i.e. exchanged whilst actually in use
• an archived/networked or stand alone anaesthesia record system if so desired
Electronics: monitoring or control?
The flow of gasses through the workstation has largely remained under pneumatic and direct mechanical control. There are currently only very few machines offering electronic servo control of gas flow and/or vapour concentrations. Although needle valves (see below) remain ubiquitous for control of gas flows, many machines do use electronic monitoring (and display) of the flow rates instead of rotameters (see Chapter 2).
The anaesthetic delivery system
A typical machine consists of:
• a rigid metal framework on wheels; attached to this is a source of compressed gas consisting of a pipeline system and/or metal cylinders containing the relevant gasses
• pressure regulators for reducing the high pressures in the attached cylinders to machine working pressures of approximately 420 kPa (60 psi) or 310 kPa (45 psi) in some countries. (The British Standard stipulates 420 kPa which is 61.3 psi. For convenience this has been rounded off to 60 psi)
• secondary regulators for damping surges in machine working pressure (see below)
• pressure gauges to show pipeline and cylinder pressures
• a method of metering (e.g. flowmeters), using adjustable valves for proportioning and mixing the various gasses
• a system for attaching vaporizers to the anaesthetic machine for the addition of volatile anaesthetic agents to the gas mixture
• a safety mechanism to warn of the failure of the oxygen supply and to prevent hypoxic mixtures of gas/vapour reaching the patient (oxygen failure warning device)
• a safety mechanism for releasing high-pressure build-up of gasses (back bar pressure relief valve) should a fault occur in the machine
• a system that bypasses the flowmeter for the administration of a high flow of pure oxygen in an emergency
• in-built connection to a circle breathing system with the facility to switch to a single outlet for delivering the gasses and vapours to any other breathing system (the common gas outlet).
The compressed gas attachments
Cylinders
The cylinders are clamped onto the machine by a yoke arrangement and secured tightly using a wing-nut (Fig. 4.2). To prevent installation of the wrong gas cylinder to a yoke, the cylinder heads are coded with appropriately positioned holes that match pins on the machine yoke. This is called a pin index system, for which there is an internationally agreed standard (ISO 407:2004) (see Fig. 1.5). A thin neoprene and aluminium washer (Bodok seal) is interposed between the cylinder head and yoke to provide a gas-tight seal when the two are clamped together.
A leak of not more than 15 ml min–1 through an open yoke is acceptable in new machines. However, in older machines the non-return valve may not be as efficient owing either to the design (the valve not being spring loaded) or to wear and tear, and could result in greater than acceptable backpressure leaks. These leaks, when occurring unexpectedly, have been shown to alter the composition of the gas leaving the flowmeter block and have resulted in the delivery of a hypoxic gas mixture to an attached breathing system (see section on Flowmeters). Blanking plugs (dummy cylinder heads) are available and should be inserted into all empty yokes to overcome this problem (Fig. 4.3).
Pipelines
Each pipeline source is attached to the machine via a gas-specific connection. In the UK this is called a NIST (non-interchangeable screw-threaded) connection and is discussed in greater detail in Chapter 1 (see Fig. 1.29). In the USA, a similar system is employed called DISS (Diameter Indexed Safety System). However, the diameters of the nipples and bodies for the various connections are smaller and not compatible with the NIST system.
Pressure (contents) gauges
The pressure in cylinders and pipelines is measured by Bourdon-type gauges (see Fig. 2.6). The gas entry to the pressure gauge has a constriction so as to smooth out surges in pressure that could damage the gauge, as well as to prevent total and rapid loss of gas should a gauge rupture. The gauges are labelled and colour coded for each gas, according to the standards for each country. They are also calibrated for each gas used on the machine. The scale on the gauge extends to a pressure at least 33% greater than either the filling pressure of the cylinder or pipeline pressure (at a temperature of 20°C). Each cylinder yoke may be fitted with a gauge or, alternatively, a single-cast brass block may be used to house the NIST/DISS pipeline connection, cylinder yoke, pressure regulator and housings for the pressure gauges (Fig 4.4). This minimizes the number of connections and potential leaks, but is a somewhat dated arrangement now.
Single-block manifold
The single-cast brass block has given way in recent years to the single-block gas manifold (Fig. 4.5), which is drilled to accommodate as many as possible of the gas pipeline and pneumatic valve interconnections. The small, compact manifold (Fig. 4.5A), for reasons of design economy, does not carry the cylinder yokes and is hence typically downstream of the primary pressure regulator which is invariably part of the cylinder yoke assembly (Fig. 4.5B).
Pressure regulators (reducing valves)
Pressure regulators are used on anaesthetic machines for three main reasons:
1. The pressure delivered from a cylinder is far too high to be used with safety in apparatus where a sudden surge of pressure might accidentally be delivered to the patient.
2. If the pressure were not reduced, flow-control (fine-adjustment) valves, tubing and various other parts of the apparatus would have to be much more robust, and a fine and accurate control of gas flow would be difficult to achieve. There would also be a danger of pressure building up and damaging other components of the apparatus.
3. As the contents of a cylinder are exhausted, the pressure within the cylinder falls. If there were no regulating mechanism to maintain a constant reduced pressure, continual adjustment would have to be made of the flow-control valve in order to maintain a given flow rate.
Working principles
In Fig. 4.6, the chamber C is enclosed on one side by the diaphragm D. As gas enters the chamber through the valve V, the pressure in the chamber is increased and the diaphragm is distended against its own elastic recoil and the force from the spring S. Eventually the pressure rises high enough to move the diaphragm far enough to close valve V. The pressure at which this occurs may be varied by adjusting the screw X so as to alter the force exerted by the spring S. If gas is allowed to escape from the outlet of the chamber, the pressure falls and valve V reopens. When the regulator is in use, a steady pressure is maintained in the chamber by the partial opening of valve V.
In another form of regulator (Adams valve), the push-rod is replaced by a ‘lazy tongs’ toggle arrangement (Fig. 4.7), which reverses the direction of the thrust transmitted from the diaphragm.
The accuracy of regulators
Let us consider that the push-rod is pushed downward by two forces: the compression in the spring and the elastic recoil of the diaphragm (Fig. 4.8). Let these be added together and represented by S. The force that opposes S consists of two parts: the high pressure (P) of the gas pushing on the valve V over an area of a; and the low pressure (p) acting on the diaphragm over an area A, so:
In the Adams valve (Fig. 4.9), it can be seen that the pressure P exerted by the high-pressure gas to open valve V is assisted by the spring and the recoil of the diaphragm S. These forces jointly oppose the force exerted by the low-pressure gas on the diaphragm, so:
Now as P falls, so does p; therefore the regulated pressure falls slightly as the cylinder pressure drops. At the same time the valve V opens slightly and this, by allowing the spring to expand, reduces S, which slightly accentuates the fall in p. The fall of p can be minimized by making S great compared with Pa.
There are several types of pressure regulator available, the choice being dependent on:
Interchangeability of regulators
Pressure regulators used to be labelled and coded for specific gasses. This is because a special alloy was required in the valve seating for some gasses (e.g. nitrous oxide) in order to prevent corrosion. However, modern regulators are designed to be compatible with all anaesthetic gasses. This is achieved by using materials such as PTFE coatings on the diaphragms together with nitrile (a hard synthetic rubber) valve seats and chrome-plated brass for the regulator body (Fig. 4.10). These too are described as ‘universal’ by their manufacturers.
Common faults in regulators
• Damage to the soft seating of valves may occur as a result of the presence of grit or dust, usually from a dirty cylinder. This may cause a steady build-up of pressure in the apparatus when the cylinder is left turned on but with no gas flowing.
• A hissing noise may indicate a leaking or burst diaphragm. The regulator will need replacing or repairing by the manufacturer or service engineer.
• Adams valves sometimes develop a fault that causes continual ‘jumping’ of the flowmeter bobbin, indicating an intermittent change of pressure and flow rate. This is usually due to the ‘lazy tongs’ sticking as a result of wear, but it may also be caused by small particles of grit or metal in the lazy tongs or the valve seating.
Relief valves on regulators
Safety blow-off valves are often fitted on the downstream side of regulators to allow the escape of gas if, by accident, the regulators fail and allow a high-output pressure. With a regulator designed to give a pressure of 420 kPa (60 psi), the relief valve may be set at 525 kPa (70 psi). These valves may be spring loaded (Fig. 4.10), in which case they close when the pressure falls again, or they may operate by rupture, in which case they remain open until repaired. As a further safety feature, a flow restrictor (usually in the form of a simple pin hole orifice) on the high pressure inlet side of the regulator, limits maximal flow from the cylinder to between 70 and 150 l min–1, ensuring that in the event of regulator failure the high-pressure relief valve can dump the maximal flow without further pressure rises.
Secondary pressure regulators
Several factors cause the machine working pressure (420 kPa in the UK) to fluctuate by up to 20%. For example, at times of peak demand in a hospital, pipeline pressures may well drop by this amount. Similarly, if an auxiliary outlet on the anaesthetic machine is used to drive a ventilator with a very high sudden and intermittent gas demand, a similar pressure drop will occur before the pipeline or cylinder is able to restore the supply. These pressure fluctuations produce parallel fluctuations in flowmeter performance. A second (secondary) regulator set below the anticipated pressure drop smoothes out the supply, minimizing these fluctuations. This is important in machines incorporating mechanically linked anti-hypoxia systems attached to the flowmeter bank (see below), as these systems assume that the oxygen supply pressure is constant in order to achieve an accurate flow of gas. A mechanically linked system would not be able to detect altered gas flow rates caused by changing pressures. Furthermore, secondary regulators also prolong the accurate supply of oxygen to the flowmeter if there is a gradual failure of the oxygen supply (i.e. cylinder emptying) prior to the oxygen failure warning device being activated.
Flow restrictors
It was an occasional practice to entirely omit regulators from the pipeline supply (420 kPa (60 psi)) in older anaesthetic machines. Sudden pressure surges at the patient end of the anaesthetic machine were prevented by flow restrictors. These consisted of constrictions in the regulated pressure pipework upstream of the flowmeters. The disadvantage of using flow restrictors without regulators was that changes in pipeline pressure were reflected in changes of flow rate, which made readjustment of the flow control valves necessary. Also, there was a danger that if there was an obstruction at the outlet from the anaesthetic machine, pressure could build up in the vaporizers and cause damage. This was normally prevented by the inclusion of a ‘blow-off’ safety valve (see section on ‘the back bar’ below). Flow restrictors do not normally require any maintenance.
Gas-tight connections within the machine
Whilst there is no standard for the design of gas piping within the machine, with the advent of nylon tubing, manufacturers tend to use pipes of differing diameters and/or sometimes colour for the different gasses to reduce the risk of accidental misconnection during servicing and assembly (see Fig 4.5A where the white tubing is for oxygen and the blue and black, respectively, for nitrous oxide and air).
Joints in metal tubing
1. One pipe may have a slightly larger diameter than the other so that they overlap (Fig. 4.11A).
2. Where the diameters are similar, both ends are inserted into a sleeve of metal (Fig. 4.11B).
The adjacent surfaces are then bonded together by brazing (applying a molten filler alloy whose melting temperature is above 430°C) or hard soldering (a similar principle using an alloy with a lower melting point). After making such a joint it is important that all traces of flux are removed. Flux is a material applied to the surfaces to be bonded, allowing the molten filler to spread more evenly. More recently, a system of brazing copper pipes and brass fittings without flux has been evolved. This is used particularly for medical gas pipeline installations.
Where provision has to be made for disconnection and reconnection of a joint, a union is used. This consists of two parts held together in a gas-tight manner, usually by a nut or cap, which screws onto a parallel male thread. Figure 4.11C shows a ball and cone (or cone seated) union, in which the seating is by direct metal-to-metal contact. A flange (or flat seated) union (Fig. 4.11D) requires a washer to complete the seal. With pipes carrying oxygen, this washer should be of non-flammable material.
For some other purposes tapered threads (Fig. 4.11E) may be used and the seal made either by screwing them down extremely tightly or by interposing a sealing compound such as PTFE (polytetrafluoroethylene/Teflon) in the form of a tape. The joint between the valve block and the body of a medical gas cylinder, for example, is sealed by a metal foil between two tapered threads and is designed to melt at high temperatures, allowing gradual release of cylinder contents.
Other detachable joints
High-density nylon tubing, connected by metal junctions, is now ubiquitous in anaesthetic machines (Fig. 4.12). It is cheaper, less prone to rupture and simpler to install. Joints are made gas-tight by an ‘O’-ring fitted between the components. This consists of a simple ring made to a fine tolerance out of a material such as neoprene. It is housed in a recess in the larger component to stop it becoming dislodged.
Valve glands
Where a valve spindle passes from an area of high pressure to one of low pressure, provision must be made to prevent the leak of gasses along the line of the spindle. This is achieved by means of a gland. In Figure 4.13, the nut ‘N’ must be screwed down sufficiently tightly to ensure that the packing is applied so closely to the spindle that no gas can escape by this route. There is provision for the nut to be tightened down further to prevent leaks as the packing wears.
The principle can be used for a high-pressure gland, such as that of an oxygen cylinder (see Chapter 1), or in a low-pressure gland such as that in a flowmeter (Fig. 4.14). In the case of high-pressure valves, a special type of leather or long-fibre asbestos was at one time used for the packing, but modern glands are filled with specially shaped nylon. Those in low-pressure flow control valves may be filled with rubber, nylon, neoprene or cotton.

Full access? Get Clinical Tree
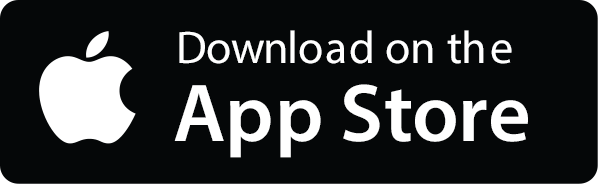
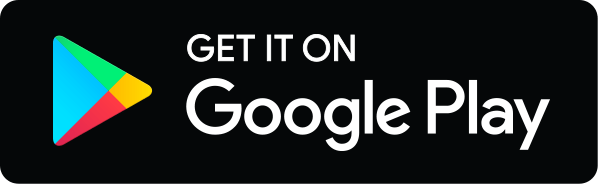
