TABLE 68.1B Staging and Management of Accidental Hypothermia | |||
![]() |
TABLE 68.2 Causes of Hypothermia | |
![]() |
Clinical Syndromes
Cardiovascular
A sympathetic response increases myocardial oxygen consumption and causes tachycardia and peripheral vasoconstriction—that is, diminished pulses, pallor, acrocyanosis, and cold extremities—in patients with mild hypothermia. Blood pressure and heart rate are initially increased, followed by bradycardia, which further deteriorates at 32°C, and consequently, cardiac output, myocardial contractility, and arterial pressure fall.
Electrocardiographic (ECG) findings include the Osborne J-wave after the QRS complex as hypothermia becomes more severe (Fig. 68.1). The Osborne J-wave is an important diagnostic feature, which can be observed in other pathologic conditions such as central nervous system lesions and sepsis, but it is frequently absent (3,13). Atrial and ventricular fibrillation are common, and electrical defibrillation during hypothermia is often ineffective. It is important to remember that the hypothermic myocardium is irritable, making placement of pulmonary artery or other central catheters dangerous.
Respiratory System
Respiratory rate falls. The patient becomes apneic or has an agonal respiratory pattern when the body temperature is less than 28°C.
Central Nervous System
The electroencephalogram (EEG) becomes flat at 19° to 29°C (14). Cerebrovascular autoregulation remains intact until the core temperature falls to below 25°C, but mentation starts to drop at 30°C. Dysarthria and hyperreflexia occur below 35°C, and hyporeflexia occurs below 32°C.

FIGURE 68.1 The electrocardiogram (ECG) shows atrial fibrillation with a very slow ventricular response, prominent J (Osborne) waves (late, terminal upright deflection of QRS complex; best seen in leads V3–V6), and nonspecific QRS widening. (Adapted from O’Keefe J, Hammill S, Freed M, et al. The Complete Guide to ECGs. 2nd ed. Royal Oak, MI: Physicians’ Press; 2002.)
Coagulation
Hypothermia produces coagulopathy via three major mechanisms (15,16). First, the enzymatic coagulation cascade is impaired; second, platelet dysfunction occurs; and third, plasma fibrinolytic activity is enhanced. Because coagulation tests, such as prothrombin time (PT) or partial thromboplastin time (PTT), are performed at 37°C in the laboratory, a major disparity between clinical coagulopathy and the reported values is frequently observed (17). A disseminated intravascular coagulation (DIC) type of syndrome is also reported (18). Clinically significant coagulopathies occur and are often associated with trauma (19,20).
Renal System
Exposure to cold induces a diuresis irrespective of the state of hydration. Centralization of the blood volume—due to the initial peripheral vasoconstriction—stimulates the diuresis. Hypothermia depresses renal blood flow by 50% at 27° to 30°C, and the renal cellular basal metabolic rate decreases (2). As a result, renal tubular cell reabsorptive function decreases and the kidney excretes a large amount of dilute urine. This is termed cold diuresis, resulting in a decreased blood volume and progressive hemoconcentration (21).
Glucose Metabolism
Blood glucose concentration commonly increases because pancreatic function, insulin activity, and/or response to insulin decrease, along with activated function of the autonomic nervous system in hypothermia. At the same time, hemoconcentration results in elevated serum glucose concentration.
Therapeutic Approach
While rewarming is the common goal in the clinical treatment of the hypothermic patient, it is both difficult and controversial to treat. At the same time as treatment is initiated, a search for and discovery of the mechanism of heat loss will play a key role in achieving a better outcome. Clinical management includes prehospital treatment as well as in-hospital critical care.
Prehospital Treatment
Hypothermia is often combined with mental and physical exhaustion. Even if a patient is found down, cold, stiff, and cyanotic, the patient is not necessarily dead and may make a recovery even when signs of life are initially absent. Thus, rescue efforts should not be given up while the patient is cold. Since ventricular fibrillation or asystole may be induced by any stimuli, such as tracheal intubation, comatose patients should be treated with extreme care.
The initial primary focus of prehospital treatment is to avoid further loss of heat. Removal of wet clothing and applying dry insulating covers such as blankets, pads, coats, and sleeping bags are effective in treating all the mechanisms of heat loss described above. During transportation, an aluminized space blanket may be used. To make the most of its effect, the patient should be carefully wrapped with additional blankets. The patient should be kept horizontal to minimize the circulatory and sympathetic change. Vigorous rubbing should be avoided because it induces vasodilation, which may be followed by hypotension or “rewarming shock.” Hot water bottles or hot packs may be used if available but should be used cautiously to avoid burn injury.
If the hypothermic patient has no signs of life, CPR should be begun without delay (4). Warmed (42° to 46°C), humidified oxygen during bag/mask ventilation (22) and warmed intravenous fluids should be given if possible. Death should not be declared below 32°C. Defibrillation may be attempted at any temperature though the specific algorithm has not been established. If the ventricular arrhythmia is refractory, it may be reasonable to perform further defibrillation attempts according to the standard BLS algorithm concurrent with rewarming strategies (4).
In-hospital Treatment
Indicated monitoring includes an ECG, Doppler evaluation of pulses, and temperature. General laboratory studies include electrolytes, complete blood count, coagulation studies (PT and partial thromboplastin time), blood urea nitrogen, creatinine, amylase, calcium, magnesium, and glucose concentrations. Radiologic examinations are indicated. Patients with hypothermia are usually dehydrated, which should be corrected with IV fluids warmed to 43°C (22). The use of glucose-containing solutions should be used with caution as hypothermic patients are usually hyperglycemic due to hypoactivity of insulin.
The various options for therapeutic approaches can be considered, and rewarming should be performed along with other supportive therapy depending upon the severity of hypothermia. Techniques of rewarming include active and passive methods, and external and internal (core) methods (Table 68.3). In a patient with hypothermia and stable circulation, active external and minimally invasive rewarming is indicated, because of the increased risk of complications, including hemorrhage or thrombosis, as well as the absence of evidence that these methods improve the outcome (5). In patients with hypothermia and cardiac instability who do not have a response to medical management, ECMO or cardiopulmonary bypass (CPB) should be considered (23–32). When patients are in stage HT IV where signs of life and vital signs are absent, there is consensus that treatment with ECMO or CPB is safe and efficient (2–5,29,31,32). Although there are some reports on successful management with warm-water lavage of the thoracic cavity (32–36), this technique is indicated for patients in stage HT IV when ECMO or CPB is not available (5,32,36).
Bronchopneumonia secondary to aspiration is a common complication. Oral intake of warm or hot drink should be avoided because obtunded, hypothermic patients may have suppressed airway protective mechanisms, including cough or gag reflexes. Prophylactic tracheal intubation may be considered if suppression of these reflexes is present or patients are in arrest as recommended in the standard ACLS guidelines (4). When the trachea is intubated, warmed (42° to 46°C), humidified oxygen should be administered (22).
Previous guidelines suggest withholding IV drugs during CPR if the patient’s core body temperature is <30°C (86°F) because it has been conventionally indicated that the hypothermic heart may be unresponsive to cardiovascular drugs, pacemaker stimulation, and defibrillation. However, it may be reasonable to consider administration of a vasopressor during cardiac arrest according to the standard ACLS algorithm concurrent with aggressive active core rewarming strategies though the recommendation for administration or withholding of medications is not clear yet (4).
HYPERTHERMIA
There are many conditions that elevate the body temperature. Table 68.4 outlines the major causes, which may be classified as hyperthermia or fever. In this section, we discuss environmental hyperthermia as well as MH and the neuroleptic malignant syndrome (NMS).
Environmental Hyperthermia—Heat Stroke
Heat stroke is a medical emergency, characterized by a high body temperature, altered mental status, and hot dry flushed skin (37). It may lead to multisystem organ dysfunction with hemorrhage and necrosis in the lungs, heart, liver, kidneys, brain, and intestines (38). Heat stroke is thought to be relatively uncommon in temperate climates. However, extreme heat events are expected to become more common in such areas as the climate continues to change. In addition, urban areas are more affected because of the “urban heat island effect” (39). The documented body temperature with this disorder is 41.1°C or more. There has been no obvious decrease in mortality in the last 50 years, which is variably quoted as ranging between 10% and 50% (38).
TABLE 68.3 Rewarming Methods |
![]() |
TABLE 68.4 Hyperthermia and Fever |
![]() |
There are several heat-related illnesses, which may take the form of heat syncope, heat cramps, heat exhaustion, and heat stroke. Heat stroke may be further classified as exertional and nonexertional (classic heatstroke). Table 68.5 outlines the syndromes.
- Heat syncope: Heat syncope is fainting due to peripheral vasodilation secondary to high ambient temperature.
- Heat cramp: Heat cramp refers to muscular cramping occurring during exercise in heat, which is related to electrolyte deficiency; it is usually benign.
- Heat exhaustion: This is often referred to as heat prostration. Heat exhaustion occurs when the individual becomes dehydrated and weak. The patient collapses from dehydration, salt depletion, and hypovolemia. Anorexia, nausea, and vomiting frequently occur. Excessive sweating leads to a loss of water and/or electrolytes. There are two mechanisms for this disorder: salt-depletion and water-depletion heat exhaustion. Salt-depletion heat exhaustion usually occurs when an unacclimatized person exercises and replaces only water. Water-depletion heat exhaustion is usually observed in an acclimatized person who has inadequate water intake during exposure to extreme heat. Serum sodium concentration may be normal or mildly elevated. The core temperature may or may not be raised (usually mild to moderate, <38°C) and tissue damage does not occur.
- Heat stroke: Heat stroke occurs when the core body temperature rises against a failing thermoregulatory system (37). The core temperature most often quoted is a rectal temperature exceeding 40.6°C (40). Heat stroke may be divided into exertional and nonexertional (classic) heat stroke (40). Exertional heat stroke occurs in previously healthy young people exercising in hot and humid climates without being acclimatized. Nonexertional heat stroke occurs during extreme heat waves, the elderly being particularly vulnerable (Table 68.6).
TABLE 68.5 Heat Syndromes | ||
![]() |
TABLE 68.6 Exertional and Nonexertional Heat Stroke Syndromes | ||
![]() |
Temperature Regulation
Normal heat production is primarily due to metabolic activity in the liver and skeletal muscle, with the liver generating most body heat at rest and muscle being the major source with exercise or shivering. Skeletal muscle heat production ranges from 65 to 85 kcal/hr at basal level, but it may increase up to 900 kcal/hr (41). Heat elimination occurs by four major mechanisms as we have discussed in hypothermia. Convection and radiation are normally the most important mechanisms for heat elimination. Evaporation becomes a major mechanism for heat dissipation with incremental skeletal muscle metabolic activity. If the ambient temperature exceeds body temperature, heat loss may depend only on evaporation. However, sweating produces only 400 to 650 kcal/hr of heat dissipation. Therefore, blood flow regulation to skin and sweat gland activity are critical in maintaining thermal balance. There is a distinction made between exertional and nonexertional heat stroke at this point; failure of thermoregulation (lack of sweating) may be more important in nonexertional (classic) heat stroke and less so in exertional heat stroke (42).
The process of thermoregulation consists of three parts: (i) afferent thermal sensing, (ii) hypothalamic processing, and (iii) efferent responses through the sympathetic system. Heat stimuli are carried by C fibers from the skin to the spinal cord. Central temperature sensors in the abdominal and thoracic viscera, spinal cord, and brain may play a significant role in preventing hyperthermia, although peripheral sensors in the skin seem to be most important. The hypothalamus integrates all afferent temperature input to alter body temperature by regulating vasomotor tone to the skin and inducing sweat formation. Neural output to the cerebral cortex is also important in modifying behavior to compensate for changes in temperature. The efferent hypothalamic response to heat consists of cutaneous vasodilation, sweat formation, and inhibition of muscle tone. Vasomotor tonic changes result in cutaneous dilation and shunting of blood away from the liver and splanchnic circulation, facilitating heat transfer from the core to skin. Sweat formation is under cholinergic sympathetic control. Removing clothes, limiting physical activity, and moving to a cooler place are important behaviors.
Acclimatization is a physiologic process whereby an individual adapts to work in a hot environment (41). Acclimatization to sustained increases in body temperature is slow and requires 1 to 2 weeks for peak effect. Sweat volume increases from 1.5 L/hr up to 4 L/hr. The sweating threshold decreases over an extended period of time. Sweat sodium concentration decreases from 30 to 60 mEq/L to about 5 mEq/L. Plasma antidiuretic hormone, growth hormone, and aldosterone levels increase. Cardiovascular mechanisms include a 10% to 25% increase in plasma volume and an increased stroke volume and cardiac output with a slowing of heart rate.
Clinical Syndrome
Heat stroke is mostly defined as a core temperature above 40.6°C, but neurologic impairment may occur at lower temperatures in some cases; indeed, neurologic dysfunction is a cardinal feature of heat stroke (43). Neurologic manifestations include slurred speech, delirium, stupor, lethargy, coma, and seizures (44). Seizures occur more commonly at temperatures above 41°C. Ataxia, dysmetria, and dysarthria may also be observed.
The cardiovascular system is commonly compromised in the presence of heat stroke. Tachydysrhythmia and hypotension frequently occur (44). Hypotension may result from translocation of blood from the central circulation to the periphery to dissipate heat, or the increased production of nitric oxide may result in vasodilation (43,45). A study of Doppler and echocardiographic findings in patients with classic heat stroke and heat exhaustion reported a circulation that was hyperdynamic, with tachycardia, resulting in high cardiac output (40). It also reported that hypovolemia was more pronounced in heat stroke patients with signs of peripheral vasoconstriction. Heat exhaustion patients were more likely to demonstrate peripheral vasodilation.
Metabolic acidosis associated with hyperlactatemia may occur. Since patients in heat stroke are in shock, the mechanism by which lactate is cleared by the liver and converted to glucose is less effective, and restoration of the circulating volume may lead to worsening metabolic acidosis as skeletal muscle is reperfused and the elevated lactate cleared. Patients typically hyperventilate to compensate for the acute acidosis with an acute respiratory alkalosis. This may lead to heat-induced tetany. After several hours, a mixed acid–base disorder may occur because of sustained tissue damage.
Significant dehydration is noted in most patients with exertional heat stroke and may be reflected as elevated blood urea nitrogen and creatinine levels or hemoconcentration. Sodium, potassium, phosphate, calcium, and magnesium serum concentrations are frequently low in the early period (41,46–50). Sodium, potassium, and magnesium are lost through increased sweating. Hypokalemia may be as a result of catecholamine release or may occur secondary to hyperventilation. Hypokalemia decreases sweat secretion and skeletal muscle blood flow, which may impair heat dissipation. Cellular death begins to occur throughout the body at temperatures above 42°C. Hyperkalemia may occur if significant skeletal muscle damage or cellular lysis develops. If significant rhabdomyolysis develops, injured cells release phosphate, which reacts with serum calcium and may lead to hypocalcemia.
Renal dysfunction is well documented in exertional heat stroke, with the incidence of acute renal failure approximately 25% (51). The cause is usually multifactorial, including direct thermal injury, the prerenal insults of volume depletion, and renal hypotension, rhabdomyolysis, and DIC (39).
Liver damage is very frequently seen and is probably related to splanchnic redistribution (52,53). Elevated liver enzymes are common.
Hemorrhagic complications may be observed. These may be petechial hemorrhages and ecchymoses, which may represent direct thermal injury or may be related to the development of DIC. Damatte et al. (44) reported that 45% of patients had laboratory evidence of DIC. This consumption coagulopathy may be further compounded by hepatocellular damage.
Complications
Cardiac complications include myocardial pump failure, tachydysrhythmia, high cardiac output, and myocardial infarction. ECG abnormalities are also observed (54). Sinus tachycardia and QT prolongation are followed by nonspecific ST-T wave changes, suggesting cardiac ischemia.
Neurologic complications include seizures, cerebral edema, and localized brain hemorrhages. Irreversible brain damage occurs above 42°C. Cerebellar impairment may persist after recovery.
Pulmonary edema may be caused by a limited cardiac function or may develop secondary to the acute respiratory distress syndrome (ARDS) (55). Pulmonary aspiration may be observed in obtunded patients.
Acute renal failure may be caused by direct heat damage, renal hypoperfusion, or rhabdomyolysis. The incidence of renal failure is about 35% with exertional heat stroke and about 5% in classic nonexertional heat stroke, with which rhabdomyolysis is less likely to coexist.
Liver damage and dysfunction occur in most patients with heat stroke. Cholestasis and centrilobular necrosis elevate bilirubin and liver enzymes, which may not be apparent until 48 to 72 hours after injury (50).
Hematologic complications include hemolysis, thrombocytopenia, and DIC (55). DIC is triggered by diffuse endothelial and organ damage, has an onset delay of 2 to 3 days after the initiating event, and is associated with high mortality.
Therapeutic Approach
Heat stroke requires prompt and effective treatments. Oxygen therapy, rapid cooling, and cautious hydration should be immediately instituted to avoid complications and achieve recovery. Tracheal intubation should be considered if the patient is obtunded or in respiratory distress. Oxygen delivery is often less than normal, and pulmonary shunt fraction is increased (56).
Rapid cooling is accomplished by external techniques. These include immersion in ice water or application of cooling blankets above and below the patient (conductive cooling technique), and wetting the skin with water or alcohol, followed by the use of fans to facilitate evaporation and heat dissipation (evaporative-convective cooling technique) (57). Both techniques usually reduce core temperature below 40°C in 1 hour. A significant disadvantage of immersion is impairment of access to the patient and limited monitoring. Another disadvantage of immersion is that intense vasoconstriction can slow the rate of heat loss (58). Vasoconstriction may also have adverse cardiovascular effects in patients with limited cardiac function because it increases cardiac afterload. Vasoconstriction may be reduced by skin massage, which prevents dermal stasis of cooled blood. More aggressive cooling techniques include gastric lavage with iced saline, cold hemodialysis, and cardiopulmonary bypass. They are only rarely required, being used in cases of refractory temperature elevation or malignant hyperthermia, in which thermogenesis is ongoing. In addition, the efficacy of rapid infusion of large-volume ice-cold intravenous fluid (LVICF)—using either lactated Ringer solution or normal saline—has been implicated in clinical trials of induced hypothermia. Bernard et al. (59) showed that 30 mL/kg of LVICF (lactated Ringer solution at 4°C) over 30 minutes decreased core temperature by 1.7°C immediately after infusion, with improvements in acid–base and renal function.
Core body temperature should be monitored closely at the rectum, bladder, or tympanic membrane. Vital signs, neurologic functions, urine output, and laboratory measurements should also be monitored closely. Laboratory measurements include arterial blood gas and serum electrolyte concentrations, especially potassium, which may increase significantly and result in life-threatening hyperkalemia. Glucose–insulin therapy should be instituted emergently in patients with ECG changes.
Intravenous volume repletion should be individualized. Volume deficit is not a prominent feature in classic nonexertional heat stroke. Central venous catheter and pulmonary artery catheter placement may be invaluable to assess volume depletion, peripheral vascular vasodilation, or primary myocardial dysfunction, especially in patients with limited cardiac reserve. Hypotension usually responds to intravenous fluids, but if an inotropic drug is needed, dobutamine is the drug of choice for heat stroke.
Seizures occur commonly in heat stroke patients and should be treated with intravenous diazepam or other benzodiazepines. The efficacy or clinical rationale for the administration of dehydrating drugs is uncertain, but these drugs may be potentially beneficial for some patients at risk of acute renal failure secondary to rhabdomyolysis, as acute renal failure can be a major cause of patient morbidity. This may be prevented by prompt repletion of intravascular volume and restabilizing adequate renal perfusion pressure. Hemodialysis may be required if hyperkalemia or other metabolic disturbances exist.
DIC may be treated with continuous infusion heparin therapy. Although this therapy brings some benefit, its utility seems uncertain (46,48,50).
Malignant Hyperthermia and the Neuroleptic Malignant Syndrome
MH and the NMS are disorders of rising body temperature related to an imbalance between heat production and heat dissipation. MH was not clearly described as a syndrome until 1960 (60,61). NMS was first described by Delay et al. (62) after the introduction of neuroleptics in 1960. These disorders are uncommon but life-threatening complications related to the administration of anesthetic or neuroleptic drugs. Their main features include hyperthermia, muscle rigidity, metabolic acidosis, and autonomic disturbances. Endogenous heat production resulting from impaired physiologic heat-dissipating mechanisms and hypothalamic temperature regulation is responsible for elevation of core body temperature in NMS. On the other hand, it usually appears intact in MH (63). Both of them are uniquely characterized by their association with various drugs, although they are distinctive from each other; associated drugs are listed in Table 68.7 (63–72). An additive in commercial succinylcholine, chlorocresol, has been reported as an additional trigger in MH (73). The in vitro halothane–caffeine contracture test on skeletal muscle helps to identify susceptible individuals and to establish with certainty the genetic nature of the disorder in most individuals (74). Similar to NMS, use of serotonergic drugs including selective serotonin reuptake inhibitors (SSRIs) is known to cause the serotonin syndrome (SS) (75). Serotonin, a neurotransmitter in the central nervous system, is involved in neuronal circuits that control sleep–wakefulness cycles, mood, and thermoregulation. SS, which presents a combination of mental status changes, autonomic hyperactivity, and neuromuscular abnormalities, is caused by overstimulation of serotonin receptors. The differential diagnosis of the symptoms may be confusing in severe SS. Serotonergic drugs that may produce SS is listed in Table 68.8 (76).
TABLE 68.7 Drugs Associated with Malignant Hyperthermia and the Neuroleptic Malignant Syndrome | |
![]() |

Full access? Get Clinical Tree
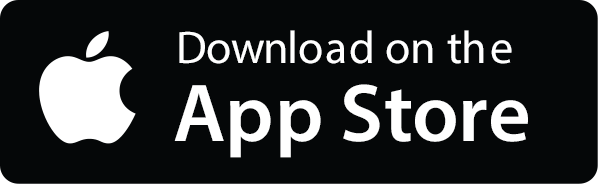
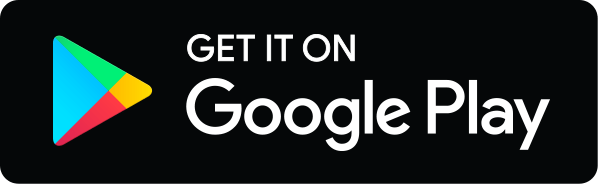