- Cornelis Kruger
- David Sidebotham
Assessment of left ventricular (LV) function is an essential part—if not the primary focus—of every echocardiogram performed in cardiac surgical patients. A strong correlation between LV function and survival has been demonstrated in several clinical scenarios. Preoperative assessment of global systolic function helps to stratify perioperative risk and identify patients likely to have difficulty in separating from CPB. In patients with hemodynamic instability, assessment of LV function helps anesthesiologists and intensivists to titrate fluid and pharmacologic therapy and may indicate the need for mechanical cardiovascular support. Identification of new SWMAs after CPB is suggestive of myocardial ischemia and may indicate the need for surgical intervention. Throughout the perioperative period, transesophageal echocardiography (TEE) provides information on intravascular volume status that is superior to that provided by any other monitoring device. Hence, the ability to accurately assess global and regional LV function and filling is an essential skill for every echocardiographer.
Global left ventricular function
Factors affecting systolic function
Loading conditions and contractility
Contractility is the intrinsic contractile strength of the ventricles and can be defined as the amount of work the heart can perform under constant loading conditions (i.e., constant preload and afterload). Preload is myocardial fiber stretch immediately before the onset of contraction, which clinically equates to left ventricular end-diastolic volume (LVEDV). Afterload is ventricular wall stress during systole. Wall stress (σ) can be expressed using the Laplace equation of
σ ∝ Δ P r w ,
Stroke volume and ejection fraction are indices of systolic performance that can be measured with echocardiography. Surrogates of ejection fraction, such as fractional area change (FAC) and fractional shortening, can also be measured. Stroke volume depends on preload, afterload, and contractility. By the Frank-Starling mechanism ( Figure 7-1 ), over the physiologic range, increased preload proportionally increases stroke volume. A sudden increase in afterload immediately reduces stroke volume. Over the next few heartbeats, stroke volume gradually recovers due to increased venous return (i.e., increased LVEDV and the Frank-Starling mechanism). However, patients with impaired LV systolic function, who are operating at the peak of their ventricular function curve (see Figure 7-1 ), are unable to increase stroke volume in response to increased afterload. Such patients are said to have reduced preload reserve, and their stroke volume is exquisitely sensitive to changes in afterload.

Loading conditions also influence ejection fraction (along with FAC and fractional shortening) but do so to a lesser extent than stroke volume. Over the physiologic range, ejection fraction is relatively independent of preload. This is illustrated in Figure 7-1 , where it can be seen that ejection fraction is the slope of the ventricular function curve (i.e., stroke volume/LVEDV), which is relatively linear over the physiologic range. However, ejection fraction is sensitive to changes in afterload, particularly when systolic function is impaired.
Ejection fraction and stroke volume do not always change in parallel. For instance, hypovolemia (decreased preload) reduces stroke volume but not necessarily ejection fraction. Acute LV failure reduces both stroke volume and ejection fraction, but chronic LV dysfunction (high LVEDV) may be associated with normal stroke volume despite low ejection fraction.
To evaluate contractility, loading conditions must be taken into account. This is difficult to do clinically but can be done experimentally by simultaneously recording LV pressure and LV volume (see Figure 7-1 ). From multiple pressure–volume loops, obtained under different loading conditions, the end-systolic pressure–volume relationship can be derived, which is a (relatively) load-independent index of contractility.
Cardiac disease
Acute systolic dysfunction may be due to myocardial ischemia, infarction, stunning, or hibernation. These conditions result in SWMAs, which, when extensive, lead to a global reduction in systolic function. A variety of conditions cause chronic LV systolic dysfunction, including coronary artery disease, valvular heart disease, hypertensive heart disease, intracardiac shunts, and the cardiomyopathies (dilated, hypertrophic, and restrictive).
There is significant overlap between systolic LV dysfunction and the clinical syndrome of congestive cardiac failure. However, not all congestive cardiac failure is due to systolic dysfunction. Other causes of congestive cardiac failure include diastolic dysfunction, constrictive pericarditis, and high-output cardiac failure. Furthermore, it is possible for a patient to have significant LV dysfunction yet be asymptomatic.
Left ventricular dimensions and shape
Chronic systolic dysfunction may be associated with spherical dilatation of the left ventricle. Causes include aortic or mitral regurgitation, myocardial infarction (“ischemic cardiomyopathy”), dilated cardiomyopathy, and some left-to-right shunts (e.g., VSDs). By contrast, with hypertensive heart disease, aortic stenosis, hypertrophic cardiomyopathy, and restrictive cardiomyopathy LV chamber dimensions are typically not increased and may even be reduced—at least, early in the disease process.
Abnormal wall thickness is common with LV systolic dysfunction. Chronic LV volume overload (e.g., aortic or mitral regurgitation) leads to eccentric hypertrophy (i.e., LV wall thickness is increased in proportion to increased chamber size). Chronic pressure overload (e.g., aortic stenosis or hypertensive heart disease) leads to concentric hypertrophy (i.e., LV wall thickness is increased in the presence of normal chamber size). Concentric hypertrophy is also seen with hypertrophic cardiomyopathy. With chronic myocardial infarction, LV wall thickness may be asymmetrical, with some myocardial segments demonstrating thinning due scarring and fibrosis.
With TEE, LV chamber dimensions (end-systolic and end-diastolic diameter and area, as described later) and wall thickness can be measured in the transgastric mid-short-axis view. Wall thickness should be measured at end diastole. To avoid the papillary muscles, and to use the same convention as for TTE, the thickness of the inferolateral (posterior) and anteroseptal walls are usually reported. With M-mode imaging in the transgastric mid-short-axis view, the ultrasound beam passes through the anterior and inferior walls; therefore, to measure the inferolateral and anteroseptal walls, 2-D imaging must be used. Normal LV end-diastolic wall thickness is 0.6 to 1.2 cm.
Relative wall thickness (RWT) can be calculated as
RWT = 2 PWT LVEDD ,
Semiquantitative assessment
In the operating room and ICU, LV systolic function must be assessed rapidly, and there may be insufficient time for quantitative assessment. In this circumstance, LV function can be graded semiquantitatively based on changes in LV radius in the transgastric mid-short-axis view as (1) normal (>30% change in radius), (2) moderately impaired (10% to 30% change in radius), and (3) severely impaired (<10% change in radius). A fourth grade, hyperdynamic, can be used to describe vigorous LV function. Serial recordings and side-by-side playback allow changes in LV function to be assessed over time.
Another semiquantitative approach is to visually estimate ejection fraction and report the result as lying within a range of values (e.g., 20% to 30%, 30% to 50%, or 50% to 70%). Semiquantitative estimates of LV systolic function show reasonable agreement to quantitative measurements.
Quantitative assessment: Motion-mode imaging
Fractional shortening
Fractional shortening (FS) is the change in LV short-axis diameter during systole, expressed as a percentage:
FS = LVEDD − LVESD LVEDD × 100 .
In the transgastric midpapillary short-axis view, the M-mode cursor is positioned through the left ventricle, transecting the inferior and anterior walls. LVEDD and LVESD are measured from the endocardial border ( Figure 7-2 ). End systole can be identified as the point at which the LV inferior wall is thickest, the point at which the cardiac dimension is smallest, or the frame immediately preceding MV opening. End diastole can be identified by the Q wave on the ECG or, preferably, as either the point at which the cardiac dimension is largest or the frame after MV closure.

Ejection fraction primarily depends on the fractional shortening of the square of the short (minor) axis of the left ventricle; the contribution of long (major) axis shortening to ejection fraction is only 3% to 7%. Thus, in the absence of SWMAs, ejection fraction and fractional shortening correlate closely. However, fractional shortening is a poor index of global LV systolic function in the presence of significant SWMAs. It is important to center the M-mode beam perpendicularly and centrally through the short axis of the left ventricle. If the M-mode beam is oblique, the LV diameter is overestimated; if the beam is not central, the LV diameter is underestimated.
Cubed method
The cubed method uses M-mode data to calculate LV volumes based on the assumption that the left ventricle has an ellipsoid shape with a 2:1 long-axis–short-axis ratio. These methods (from Quinones et al. and Teichholz ) are no longer recommended, as they are associated with inaccuracies due to geometric assumptions.
Fractional shortening at the midwall
With concentric LV hypertrophy, the subendocardium contributes more to systolic thickening than the endocardium or epicardium. Thus, values for fractional shortening are increased in the presence of LV hypertrophy when LVEDD and LVESD are measured from the endocardial border. Measurements made at the midwall provide a more useful measure of global systolic LV function in this circumstance.
Velocity of circumferential fiber shortening
The velocity of circumferential shortening ( V cf ) is a measure of the mean velocity of ventricular shortening in the LV short axis and is calculated as
V cf = FS ET ,
Mitral annular descent
During systole, the mitral annulus moves toward the relatively stationary apex, shortening the long axis of the left ventricle. The magnitude of systolic mitral annular descent provides a simple index of global LV function that is useful when endocardial definition is poor. Mitral annular decent can be measured using either 2-D or M-mode imaging, but measurements are more easily performed with M-mode. An M-mode cursor is placed through the lateral mitral annulus in the midesophageal four-chamber view, ensuring the longitudinal motion of the heart is parallel to the M-mode line ( Figure 7-3 ). A value above 10 mm is normal. A value below 8 mm correlates with an ejection fraction of less than 50%, with a sensitivity of 98% and a specificity of 82%. Mitral annular descent cannot be used to distinguish between moderate and severe LV dysfunction.

Quantitative assessment: Two-dimensional imaging
Fractional area change
FAC is widely used for quantifying global systolic function with TEE in the perioperative setting. FAC is the proportional change in LV area during systole, expressed as a percentage,
FAC = LVEDA − LVESA LVEDA × 100 ,
Typical normal values for LVEDA, LVESA, and FAC are 12 to 16 cm 2 , 3 to 6 cm 2 , and 36% to 64%, respectively, with the caveat that loading conditions and other factors, listed previously in the “Fractional Shortening” section, significantly influence LV areas. Good agreement has been demonstrated between TEE measurements of FAC and ejection fraction.
FAC is measured in the transgastric mid-short-axis view. Using the freeze and scroll functions, the largest (LVEDA) and the smallest (LVESA) areas are identified and the areas are traced ( Figure 7-4 ). The largest and smallest cavity sizes should be chosen irrespective of the ECG, because the time delay between the image frames and the phase of the ECG is not always the same. If the largest area is difficult to assess visually, then the Q wave of the ECG may be used to determine the LVEDA. The endocardial borders of the septum and the lateral walls are often poorly visualized, as they lie parallel to the echo beam and therefore provide weak specular reflection. In such instances, the trace should be extrapolated to form a smooth curve. To estimate the true location of the endocardial surface in the region of echo dropout, it is useful to scroll rapidly back and forth between end-diastolic and end-systolic frames, as the endocardial border is often more obvious in systole due to the enhanced reflectance from the more trabeculated surface. Extrapolating the endocardial surface assumes normal wall motion in the region of uncertainty and overestimates FAC if wall motion is abnormal.

The traced area can include or not include the papillary muscles. If the papillary muscles are not included in the traced area, the blood pool area is measured. Software that allows automatic border detection measures the blood pool area. Thus, excluding the papillary muscles from the traced area is also recommended when tracing borders manually (see Figure 7-4 ).
Automated border detection provides rapid, continuous evaluation of FAC. The blood tissue interface is recognized automatically by acoustic quantification of ultrasonic backscatter signals. FAC by automatic border detection using TEE demonstrates good agreement with ejection fraction calculated by radionuclide methods.
The main shortcoming of FAC is that it does not take account of basal and apical SWMAs, which is important given that the apex is commonly affected by wall motion abnormalities.
Ejection fraction
Ejection fraction (EF) is the proportional change in LV volume during systole, expressed as a percentage:
EF = SV LVEDV × 100
SV = LVEDV − LVESV ,
where LVESV is left ventricular end-systolic volume. Typical normal ranges for LVEDV, LVESV, and ejection fraction are 80 to 180 mL, 30 to 90 mL, and 55% to 75% respectively, with the caveat that volume measurements highly depend on loading conditions and are influenced by diastolic function, RV function, and pericardial and intrathoracic pressure. In addition, women typically have lower volume measurements than men. Most published figures provide volume measurements indexed to body surface area (with typical ranges for LVEDV and LVESV being 50 to 100 and 15 to 40 mL/m 2 , respectively); however, indexed values are cumbersome to interpret in the perioperative period.
Following myocardial infarction, LVESV and ejection fraction are highly predictive of mortality. In one study, an LVESV below 95 mL was associated with a 94% 5-year survival rate, whereas LVESVs of 95 to 130 mL and higher than 130 mL were associated with 5-year survival rates of 78% and 52%, respectively. Similarly, an ejection fraction greater than 50% was associated with a 94% 5-year survival rate, whereas an ejection fraction below 40% was associated with a 65% 5-year survival rate.
There are numerous formulae for calculating LV volume from 2-D images. The two methods recommended by the ASE are the biplane and the single-plane ellipsoid methods.
Biplane method (modified Simpson method, method of discs)
LV volume is calculated from a summation of the volumes of a stack of discs as
V = ( π 4 ) ∑ i = 1 20 a 1 b 1 × ( L 20 ) ,

Full access? Get Clinical Tree
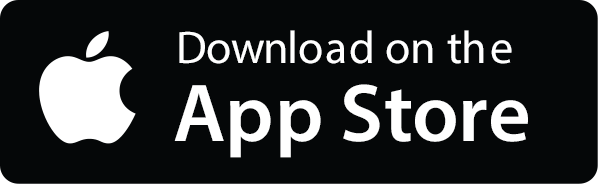
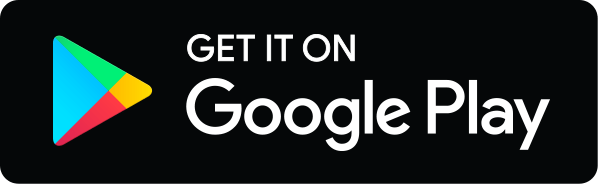