Sympathetic Neural Blockade of Upper and Lower Extremity
Harald Breivik
Michael J. Cousins
The effects of sympathetic nerves in maintaining normal constrictor tone in the blood vessels of the skin have been known since the classic work of Claude Bernard in 1852. The well–known observation of increased skin temperature of the foot after surgical lumbar sympathectomy was first made by Hunter and Royle in 1925 (1). Surgical sympathectomy has been performed at some clinics, as reported by De Bakey in 1950, to promote healing of ischemic cutaneous ulcers and to relieve pain in the foot at rest (rest pain) (2); however, in many vascular clinics today more emphasis is placed on vascular grafting and stenting procedures. These refined surgical techniques undoubtedly have greatly improved the prognosis of the patient with occlusive vascular disease. Despite this movement away from sympathetic ablation, there is considerable potential benefit from sympathetic neural blockade as an adjunct to vascular surgery or as primary treatment for patients with rest pain who are not fit for, or not amenable to, vascular reconstruction. The large series of 1,666 patients with neurolytic lumbar sympathetic blocks reported in 1970 by Reid and colleagues bears testimony to this, and it is surprising that many clinics have largely neglected this important option in the treatment of vascular disease (3).
Mandl first described the technique of lumbar sympathetic neural blockade in 1926, and his technique was clearly very similar to that for celiac plexus blockade described by Kappis in 1919 (4,5). A similar neglect of celiac plexus block for analgesia in upper abdominal cancer has been apparent (see Chapter 45). The classic “anterior” approach to stellate ganglion blockade was initiated by Leriche (6) in 1934, and it forms the basis of the technique described in this chapter (7).
Sympathetic blockade is produced together with motor and sensory blockade during regional block for operative surgery (e.g., spinal and epidural anesthesia, brachial plexus block). It has been asserted that “differential” sympathetic blockade without sensory and motor block can be produced using low concentrations of local anesthetic during epidural or spinal subarachnoid block. This selective vulnerability has been applied to clarify the mechanisms of individual patients’ chronic pain. We believe that selective sympathetic ganglion block provides more reliable information (see Chapter 38). In the management of acute pain, epidural block at the appropriate segmental level relieves labor pain and the pain of renal colic by blocking visceral (sympathetic afferent) nociceptive and sympathetic efferent nerve fibers (see later discussion and Fig. 39-1). However, the most specific technique for sympathetic blockade is selective blockade of the sympathetic ganglia.
Because the sympathetic ganglia are, except in the thoracic region, relatively well separated from somatic nerves, it is possible to achieve sympathetic blockade without loss of sensory or motor function. Selective sympathetic blockade offers the possibility of treating a variety of conditions in which reduced sympathetic activity might be beneficial. With careful technique, it is even possible to achieve permanent neurolytic blockade with essentially no loss of somatic sensory and motor function. Thus, sympathetic blockade, at the three major levels indicated in Figure 39-2, is potentially a rewarding series of techniques for the diagnosis and management of acute and chronic pain syndromes and other conditions (Table 39-1). Our clinical experience has been that, of all neural blockade techniques, lumbar sympathetic block for lower extremity rest pain due to vascular insufficiency and celiac plexus block for visceral pain in upper abdominal cancer offer the most benefit at the lowest risk.
Celiac plexus blockade, superior hypogastric plexus block, and ganglion impar block are described in Chapter 45, and cervical, thoracic, and lumbar sympathetic blocks are described in the present chapter, along with intravenous regional sympathetic (IVRS) block. Despite a duration of only up to 2 weeks, repeated IVRS efferent blocks are an attractive alternative to the higher-risk techniques of thoracic sympathetic block and thoracic surgical or thoracoscopic sympathectomy. However, the effects of IVRS blocks differ: Ganglion blocks will intercept efferent as well as afferent nerve fibres, although the latter are in the minority (8), IVRS blocks efferents only.
It may be possible to use either unilateral or bilateral lumbar sympathetic block for some kinds of pelvic pain (particularly urogenital). Doing so poses a much smaller risk than neurolytic subarachnoid block, with its possibility of loss of bladder function (see Chapter 42 and 50), and it is less invasive than subarachnoid opioid plus bupivacaine catheter infusion. To take full advantage of sympathetic neural blockade, it is essential to bear in mind the general features of the anatomy and physiology of the peripheral sympathetic nervous system (as described in the next section) and the regional anatomy, as described in connection with each of the techniques of sympathetic blockade. It is also important to use objective methods to evaluate the completeness of sympathetic blockade and its clinical effects.
![]() Figure 39-1. Peripheral sympathetic nervous system. Cell bodies are located in the intermediolateral cell column of T1–L2 spinal segments. Efferent fibers (cholinergic) pass by way of the ventral root to a white ramus communicans and then to the paravertebral sympathetic ganglia or to more remotely located ganglia, such as the celiac ganglion. From each ganglion, they give rise to adrenergic fibers to supply viscera (e.g., from the celiac ganglion) or to join somatic nerves (from lumbar sympathetic ganglion) to supply efferent fibers to the limbs (sudomotor and vasomotor effects). In the case of lumbar sympathetic ganglia, the adrenergic fibers swing backward by a gray ramus communicans to join the somatic nerve. Afferent fibers travel by way of ganglia, such as the celiac and lumbar sympathetic, without synapsing, and reach somatic nerves and then their cell bodies in the dorsal root ganglia. They then pass to the dorsal root and synapse with interneurons in the intermediolateral area of the spinal cord. These afferent fibers convey pain impulses from the viscera and are similar to nociceptive afferents, except that they pass without synapsing through sympathetic ganglia. Part of the parasympathetic nervous system is indicated by the vagus nerve to and from the gut. These vagal fibers pass through the celiac plexus but do not synapse in the celiac ganglion. Their synapse is at gut wall level (see Fig. 39-5). The submucosal and the myenteric part of the enteric nervous system are indicated in the cross-section of the gut wall. |
Sympathetic Nervous System
Anatomy and Physiology
The peripheral sympathetic nervous system begins as efferent preganglionic fibers from neurons in the intermediolateral column of the spinal cord, passing in the ventral roots from T1 to L2 out of the spinal canal to run separately as white rami communicantes to ganglia in the sympathetic chain, alongside the vertebral bodies (Fig. 39-1). In the lower cervical region, the chain lies at the anterolateral aspect of the vertebral body; in the thorax it is adjacent to the neck of the ribs, still relatively close to somatic roots (Fig. 39-3). In the lumbar region, however, the chain angles forward to lie anterolateral to the vertebral bodies and is separated from somatic roots by psoas muscle and psoas fascia (Fig. 39-4). The preganglionic fibers pass a variable distance in the sympathetic chain to reach neurons in ganglia in the chain, or they may extend to peripherally located ganglia (i.e., in the gut; Fig. 39-1). The inconstant level of relay in the chain itself may be responsible for a number of disappointing results from an apparently technically successful block. Sympathetic ganglia are segmentally located in the chest (T1–T12). There are also three cervical ganglia, four to five lumbar ganglia, four sacral ganglia, and one unpaired coccygeal ganglion (the “ganglion impar”). The postganglionic fibers are widely distributed, partly to join peripheral nerves (via the gray rami communicantes) and partly to join vessels in different organs. The sympathetic chain not only receives efferent preganglionic but also afferent visceral fibers, which conduct pain from head, neck, and upper extremity (cervicothoracic ganglia); abdominal viscera (celiac plexus, hypogastric plexus) (see Chapter 45); and urogenital system and lower extremity (lumbar ganglia; Fig. 39-2).
The sympathetic nervous system is one part of the autonomic nervous system. Most viscera also receive efferent and transmit afferent impulses via the parasympathetic nervous system (Fig. 39-5). The enteric nervous system (the submucosal and myenteric parts) of the gastrointestinal tract interacts with the sympathetic and the parasympathetic systems (Fig. 39-1 and 39-5).
Sympathetic Efferents to Blood Vessels
The great vessels (carotids, aorta, vena cava) receive direct postganglionic filaments from adjacent sympathetic ganglia (Fig. 39-6) and from plexuses (Fig. 39-2). The main outflow of preganglionic fibers through the ventral roots follows four alternative courses: (a) synapse with postganglionic neuron in ganglia at the same level or adjacent ganglia and travel in somatic nerves to the vessels; (b) synapse in ganglia and pass directly to vessels via filaments; (c) pass directly through sympathetic ganglia and synapse in prevertebral plexuses, then reach vessels (Fig. 39-6); or (d) pass through sympathetic ganglia and ascend or descend to synapse in ganglia above (e.g., cervical) or below (e.g., lower lumbar) (Fig. 39-7).
Vascular nerves and filaments from these diverse sources are united around individual vessels in extensive “perivascular adventitial plexuses,” which in turn ramify into plexuses between adventitia and media and between media and intima. Plexuses are augmented by branches from nearby cranial or spinal nerves. Thus, there is great overlap of innervation originating from several spinal cord segments. Arteries and arterioles are more richly supplied than veins and venules. Although nerve fibers accompany venules, it is not known whether they serve a vasoconstrictor role.
The complex influences of remote and local factors on vascular tone are illustrated in Figures 39-8,39-9,39-10.
Sympathetic fibers are generally found in a deep fascial plane and are less accessible than segmental nerves. Interruption of sympathetic nerve fibers by neural blockade can be achieved in the following ways: at the sympathetic chain by anatomically specific sympathetic blockade, at peripheral nerves by a mixed nerve block, at a vessel by perivascular infiltration, or to some extent by intradural or extradural injection of local anesthetic. Sympathetic efferent activity can also be blocked pharmacologically by an α-receptor blocker (phentolamine, Dibenzyline); by a “depleter” of norepinephrine activity in sympathetic nerve ending (guanethidine or reserpine); or by a β-receptor blocker, propranolol (β1 and β2) or metoprolol,
atenolol, esmolol (mainly β1-receptor). Norepinephrine released from sympathetic nerve endings acts on both presynaptic and postsynaptic receptors (Fig. 39-11). Phenoxybenzamine and phentolamine are nonspecific α-blockers, acting on both pre- and postsynaptic receptors. Blockade of presynaptic receptors interrupts the negative feedback that modifies norepinephrine release. More selective postsynaptic blockers (e.g., prazosin) have a much more sustained degree of α-blockade (Fig. 39-11).
atenolol, esmolol (mainly β1-receptor). Norepinephrine released from sympathetic nerve endings acts on both presynaptic and postsynaptic receptors (Fig. 39-11). Phenoxybenzamine and phentolamine are nonspecific α-blockers, acting on both pre- and postsynaptic receptors. Blockade of presynaptic receptors interrupts the negative feedback that modifies norepinephrine release. More selective postsynaptic blockers (e.g., prazosin) have a much more sustained degree of α-blockade (Fig. 39-11).
Table 39-1 Clinically painful conditions that sympathetic blockade may benefit | |
---|---|
|
Function of α- and β-Blockers
Vasoconstriction by α-Receptors
Arterioles (in the skin and in the splanchnic area), smaller arteries, and, in particular, peripheral veins, are normally under moderate vasoconstrictor influence. Pain, anxiety, and blood loss can provoke a very marked increase in arteriolar vasoconstrictor tone, mediated by the sympathetic nervous system. This increase in resistance, particularly in skin vessels, influences the distribution of blood flow. In addition, the associated increase in venous vascular tone decreases the compliance of the venous system, reducing its blood content and increasing venous pressure. Co-release of neuropeptide-Y from some sympathetic nerve endings increases and prolongs the vasoconstrictive effect of norepinephrine (8).
Heart Muscle Activity (Chronotropic and Inotropic Effect)
β1-Stimulation causes increased heart rate and increased cardiac contractility; thus, high (T1–T4) thoracic sympathetic blockade may cause a marked reduction in cardiac output.
Bronchial Tone
β2-Stimulation causes bronchodilation, so that it is theoretically possible for thoracic sympathetic blockade to cause bronchoconstriction, although this does not appear to be the case (see Chapter 11).
Vasodilatation
β2-Stimulation causes vasodilatation in some vascular beds (e.g., muscle); however, this is a minor effect and is overridden by local metabolic effects (see later in this chapter).
Smooth Muscle Tone
In the gut and bladder, β2-stimulation causes smooth muscle relaxation and sphincter contraction. Thus, sympathetic blockade results in smooth muscle contraction (a small, contracted gut) and sphincter relaxation. These effects provide excellent surgical access during procedures such as abdominoperineal resection. Sudomotor structures (sweat glands) and hair follicles have the same postganglionic efferents as do blood vessels; however, the neurotransmitter in sweat glands is acetylcholine.
![]() Figure 39-5. The sympathetic and parasympathetic parts of the autonomic nervous system. Continuous lines are preganglionic axons; dotted lines are postganglionic axons. The sympathetic outflow to skin and deep somatic structures of the extremities and trunk are shown in Figure 39-7. III, oculomotor nerve; VII, facial nerve; IX, glossopharyngeal nerve. From Jänig W. The integrative action of the autonomic nervous system. In: Neurobiology of Homeostasis. Cambridge: Cambridge University Press, 2006, with permission. |
Metabolic
The sympathetic nervous system has a metabolic effect that is said to explain the relaxation of smooth muscle in vessels (β2) and gut, and its effect on the heart muscle. This metabolic effect is widespread and also affects carbohydrate and lipid distribution and utilization (9).
Nociception is mediated in various ways by the sympathetic nervous system. Uterine nociceptive input, as in labor pain, is transmitted by afferent fibers that traverse the lower thoracic sympathetic ganglia, whereas pain from upper abdominal viscera and the gut, as far distal as the descending colon, can be relieved adequately by celiac ganglion block. Nociceptive input from some pelvic viscera may be transmitted via lumbar sympathetic ganglia (Fig. 39-2). Sympathetic efferents may influence limb pains (10,11,12). After nerve or tissue trauma, release of neurotransmitters such as norepinephrine increases discharge from peripheral primary afferent nociceptors. Denervation hypersensitivity from augmented adrenoceptor activity may increase discharge from primary afferent nociceptors in the absence of increased sympathetic efferent nerve activity. (see Chapters 31 and 32). Recently, Jørum and colleagues (13) documented direct stimulation of nociceptive fibers by sympathetic efferent discharge in a patient with sympathetically maintained pain (13). Also, microcirculatory changes caused by intense sympathetic activity may alter the local biochemical environment so as to enhance nociceptor activity (Fig. 39-12). Sympathetic blockade may reverse such effects, provided it is done before abnormal nociceptive activity is permanently established rostral to the spinal cord. Visceral afferent
inputs can give rise to sensations of pain and discomfort, shape emotions, and produce reflex effects on autonomic target organs.
inputs can give rise to sensations of pain and discomfort, shape emotions, and produce reflex effects on autonomic target organs.
Physiologic Effects on Peripheral Blood Flow
A regional sympathetic block has its primary and most obvious effect on vasomotor activity. In a normal subject, this leads to dilatation of veins, promoting an accumulation of blood in the veins, and dilatation of the arterial vessels, leading to a fall in the peripheral resistance and, if the perfusion pressure has not been altered, to an increased capillary blood flow.
In a normal subject, complete sympathetic block will be followed by visibly dilated veins or by increased blood flow, seen clinically in reduced capillary refill time or as measured by plethysmography (Figs. 39-13 and 39-14), laser Doppler flowmetry, (14,15) or 133Xe clearance, all accurate means to measure capillary blood flow. Peripheral pulse waves will be enlarged on oscillometric recordings. Vasoconstrictor responses, as to the “ice response,” are abolished (Figs. 39-13 and 39-14). The blood flow increase will, to a large extent, be restricted to the skin, followed by an increase in skin temperature and a marked feeling of warmth in the extremity (Fig. 39-15) (2). Skin capillary oxygen tension and venous oxygen tension and saturation are also increased. A widespread block will cause a peripheral pooling of blood, diminishing the venous return and lowering cardiac output and blood pressure.
Application to Improve Blood Flow in Peripheral Vascular Disease
Muscle blood flow, being automatically regulated according to muscle metabolism, should not be affected by sympathetic blockade at rest, during work, or after ischemia (1). Thus, reduced muscle flow (claudication) may not be helped by sympathetic block (Fig. 35-15). Therefore, it seems logical to use sympathetic blockade to improve the blood flow in a patient with insufficient peripheral superficial blood flow due to vasospasm or arterial disease (i.e., rest pain) (Fig. 39-15) (1,16). It is not possible, however, to predict the effect of sympathetic blockade in a patient with a diseased vascular system, as explained with the aid of the following illustrations. Figure 39-16 shows an artery that divides into two smaller branches. The total flow (QA) in the artery is proportional to the perfusion pressure (P) and inversely proportional to the peripheral resistance (PR). In each branch (B and C) the flows (QB and QC) are affected by the perfusion pressures, almost the same as in the artery (A) and inversely related to the regional resistance (RRB and RRC) in each branch. A blockade of the sympathetic fibers to branch B alone will have very little effect on the perfusion pressure (Fig. 39-17). As the regional resistance decreases in branch B, the flow through this vessel will increase. A unilateral sympathetic blockade (in humans) is generally followed by a slight increase in vasomotor tone in the contralateral side, thus increasing the regional resistance and reducing the blood flow through this part of the vascular system.
In Figure 39-18, an arterial obstruction in branch B is shown. Such an obstruction will in itself diminish blood flow by mechanically increasing the regional resistance; however, decreased blood flow across the obstruction might at least be compensated by an increase in the collateral blood flow. A widespread sympathetic blockade, as illustrated in Figure 39-19, will diminish the regional resistance, mainly in branch C with its undamaged vessels. The blood flow will be diverted into this part of the vascular tree, and thus blood will be shunted from the diseased part (branch B). Such “stealing” of blood is known to occur in patients with advanced arterial disease. In theory, stealing can occur at three different levels: a generalized vasodilatation in the body will steal blood from, for instance, one extremity; increased blood flow around the hip and in the pelvis will diminish distal blood flow to the peripheral part of the lower extremity; or increased skin blood flow might steal blood from the muscles (Fig. 39-15) (1,17,18).
In contrast, a localized vasodilatation that affects the collaterals to branch B and vessels beyond the arterial obstruction should increase blood flow to this region (Fig. 39-20).
If a decrease in vasomotor tone in the collaterals cannot be achieved, the maintenance of a good perfusion pressure is essential, as stressed by Lassen and Larsen (19,20).
From this discussion, it is obvious that the clinical effect of a sympathetic block cannot easily be predicted. Only physiologic studies in patients and clinical experience will identify when a sympathetic block is indicated.
In patients with occlusive vascular disease, the prime indication for surgical or chemical sympathectomy is rest pain in a
limb not amenable to direct arterial reconstruction (2). Because of associated coronary artery disease, pulmonary disease, and other physical conditions, many patients in this situation are poor candidates for surgery and are thus better candidates for neurolytic sympathectomy (16). However, the clinical results of both surgical and neurolytic sympathectomy, as reported in the literature, are contradictory. This might be explained by a poor selection of patients or by a lack of use of independent assessment of successful sympathetic ablation and its separate effects on blood flow and pain. This task may be simplified if one of the methods of evaluation in each category depicted in Table 39-2.
limb not amenable to direct arterial reconstruction (2). Because of associated coronary artery disease, pulmonary disease, and other physical conditions, many patients in this situation are poor candidates for surgery and are thus better candidates for neurolytic sympathectomy (16). However, the clinical results of both surgical and neurolytic sympathectomy, as reported in the literature, are contradictory. This might be explained by a poor selection of patients or by a lack of use of independent assessment of successful sympathetic ablation and its separate effects on blood flow and pain. This task may be simplified if one of the methods of evaluation in each category depicted in Table 39-2.
The detrimental effects of generalized systemic vasodilatation in patients with arteriosclerotic vascular disease that causes rest pain or intermittent claudication have been well described and are generally accepted today. The most important of these effects is the maldistribution of blood flow away from vital organs, such as the myocardium and central nervous system (CNS) (18).
It has been suggested that an increased skin blood flow should always be present in the presence of regional vasodilatation. This finding has also been reported in patients with obliterative arterial disease (21,22,23).
Although skin blood flow is markedly under the control of sympathetic activity, a decreased blood flow has been demonstrated in patients with severe arteriosclerosis and, in particular, in patients with lower limb vascular disease who have a low ankle blood pressure (less than 60/20 mm Hg) before lumbar sympathetic blockade (23,24,25,26,27,28). In these patients, the vascular lesions in the periphery are so extreme that, hypothetically, a “proximal stealing” always occurs, and thus a fall in the perfusion pressure peripherally is followed by a vasodilatation in vessels located proximally. Although rare, there are reports of worsening of pain and gangrene (29). Remarkably enough, an increase in skin temperature is not always related to an increase in blood flow through the skin and may merely reflect venous pooling and local inflammatory changes (28). During spinal anesthesia, changes in skin temperature are not closely correlated to changes in skin blood flow, as evaluated by laser Doppler flowmetry (15,30).
The blood flow through muscle is automatically regulated according to local metabolic needs. Thus, in intermittent claudication, muscle tissue hypoxia and accumulation of metabolites during exercise should be followed by maximal dilatation of the muscle blood vessels, so that sympathetic blockade should be of no value or may even worsen the symptoms because of “stealing” blood flow to the skin (1,31,32). However, because pain is a primary factor in provoking increased sympathetic activity, its presence could result in vasoconstriction in the collateral vessels that supply the affected muscle (Fig. 39-15). Sympathetic blockade under these circumstances may decrease pain and thereby improve blood flow to muscle, thus explaining why sympathectomy benefits some patients with intermittent claudication (3,21,33). This assumption and the clinical experience of several investigators are supported by a metabolic study in which deep venous blood was drawn before and at the maximum of a one-leg bicycle ergometer test in patients with intermittent claudication (34). In patients with fairly high and segmentally located arterial lesions given sympathetic block (Group I), the rise in femoral venoarterial lactate difference during exercise was not as marked as before
their sympathetic block, indicating an improvement in nutrient blood flow. By contrast, in a group of patients with multiple vascular lesions (Group II), no improvement in nutrient blood flow was achieved following sympathetic blockade. In Group I, eight of 10 also experienced less ischemic pain on exercise during the sympathetic blockade. No patients in Group II experienced such pain relief. The most important effect of sympathetic blockade in Group I seemed to be pain relief during exercise, at least partly because of improved blood flow and also enhancement of the development of collateral vessels, which is known to occur over time, providing opportunities for revascularization (Table 39-3). It should be acknowledged that, with respect to pain evaluation, a placebo effect may occur, as has been reported with lumbar sympathetic blockade (35).
their sympathetic block, indicating an improvement in nutrient blood flow. By contrast, in a group of patients with multiple vascular lesions (Group II), no improvement in nutrient blood flow was achieved following sympathetic blockade. In Group I, eight of 10 also experienced less ischemic pain on exercise during the sympathetic blockade. No patients in Group II experienced such pain relief. The most important effect of sympathetic blockade in Group I seemed to be pain relief during exercise, at least partly because of improved blood flow and also enhancement of the development of collateral vessels, which is known to occur over time, providing opportunities for revascularization (Table 39-3). It should be acknowledged that, with respect to pain evaluation, a placebo effect may occur, as has been reported with lumbar sympathetic blockade (35).
![]() Figure 39-12. Peripheral mechanisms of pain, sympathetic activity, and microcirculatory changes. Physical stimuli (e.g., trauma), the chemical environment (e.g., H+ changes), algesic substance (e.g., serotonin [5HT] and bradykinin [BK]), and microcirculatory changes (e.g., edema) may all modify peripheral nociceptor sensitivity. Increased nociceptor activity increases afferent fiber activity, with resultant increases in efferent sympathetic vasoconstriction, and also norepinephrine (noradrenaline [NA]) release with further increases in nociceptor sensitivity. Thus, a repetitive cycle is set up. Substance P (SP) is probably the peripheral pain transmitter. Prostaglandins (PGE) also increase nociceptor sensitivity. Points of blockade of pain (shaded and marked “blocker”) are as follows: 1. Afferent fibers (local anesthetics); 2. efferent sympathetic fibers (local anesthetics); and 3. prostaglandin synthesis (e.g., nonsteroidal anti-inflammatory drugs given orally). All three may relieve pain and produce an associated improvement in microcirculation by breaking the repetitive cycle. New approaches to pain relief include substance P depletion (e.g., by capsaicin). Additional points of blockade to produce vasodilation include the following: 1. Norepinephrine depletion at sympathetic nerve endings; 2. norepinephrine receptor blockade (see Fig. 39-33); 3. selective increase in levels of the prostaglandins PGE and PGE1 (by intravascular infusion); and 4. calcium channel blockade (see Fig. 39-33). |
Lumbar sympathetic blockade in connection with vascular surgery improves the flow through reconstructed vessels and should be of value in the immediate postoperative period (21,36,37,38,39). Although less selective, this effect can also be achieved with epidural sympathetic block. Whether blockade of β-fibers (sympathetic preganglionic fiber) occurs during spinal and epidural blockade has been questioned (15,40,41,42,43,44). Efferent sympathetic change has been studied with the help of skin conductance response (SCR), in which different stimuli (e.g., electrical stimulus, short, deep breath, verbal stimulation) have been used. In spinal analgesia, the sympathetic blockade starts at a level much more caudal than that of analgesia, in contrast to what has historically been stated in the literature (45). Even with T1 blockade (which should block all preganglionic fibers), an SCR is often seen in the foot, indicating that β-fibers are not blocked as easily as previously thought. In most cases, when a T6 blockade has been achieved, a marked depression of the sympathetic responses is seen mainly in the foot (T12–L1). This partial sympathetic blockade, however, disappears much earlier than the analgesia, in most cases starting 20 to 40 minutes after the injection of the spinal anesthetic. Similar results have been seen when the skin blood flow has been studied with the help of laser Doppler flowmetry (14,15). Also with a high blockade (T4–T6), an increased blood flow was seen only in the foot and in the thigh, at segment levels far below that of analgesia. A very high spinal analgesia is thus needed to make sure that marked depression of sympathetic activity in the lower extremity is produced. Changes in SCR related to the level of epidural blockade are very similar to those seen in spinal analgesia (44).
The increase in limb blood flow resulting from epidural block has been clinically related to a lower incidence of postoperative deep venous thrombosis (see Chapter 11). This effect is most likely correlated with an increase in cardiac output, provoked by the local anesthetic itself (see Chapters 2, 4, and 11). The local anesthetics lidocaine and mepivacaine, when applied directly into the arterial vessels, produce a contraction, in contrast to what is seen during an IV infusion (46).
The limited blocking of sympathetic fibers produced by epidural blockade also explains the fact that a very high epidural blockade is required to ablate stress responses during and after lower abdominal surgery. In upper abdominal surgery, epidural blockade is less effective in blocking the stress
response. The latter can be rendered much more complete if a splanchnic blockade is added (see Chapter 6).
response. The latter can be rendered much more complete if a splanchnic blockade is added (see Chapter 6).
Proper selection of patients for sympathetic blockade is obviously of great importance. Because the number of patients with vascular disease is very large, complicated and time-consuming physiologic studies are not always feasible. The use of continuous catheter sympathetic blockade that lasts for 5 days is one method of obtaining a clinical evaluation of the effect of such blockade, which should at least identify patients whose symptoms are exacerbated by blockade (47). A selective alternative is IVRS block, which can eliminate technical failure and is a useful preliminary test for whether surgical sympathectomy or permanent blockade by chemical sympathectomy will be successful (48,49). The often prolonged duration (1–3 weeks) of response to IV sympathetic block can allow adequate time for clinical assessment. A third alternative is to perform
diagnostic block with long-acting local anesthetic mixed with contrast medium, and then check the adequacy of sympathetic chain coverage under an image intensifier; however, this has proved the least reliable of the three (16,39).
diagnostic block with long-acting local anesthetic mixed with contrast medium, and then check the adequacy of sympathetic chain coverage under an image intensifier; however, this has proved the least reliable of the three (16,39).
In a clinical series, Kövamees and Löfström found that the most beneficial effect of sympathetic blockade was relief of rest pain (19 of 23, or 83%), with a somewhat lesser effect of improved healing of ulcers (23 of 55, or 42%) (Table 39-4) (24). Increased walking tolerance (seven of 16, or 44%) was a significant benefit in a small group of patients with intermittent claudication. Sympathetic blockade was part of a general treatment program that included mobilization and wound treatment, and sometimes infusion of low-molecular-weight dextran. It was not possible to attribute improvement to the sympathetic blockade alone. However, Cousins and colleagues (16) reported that duration of relief of rest pain was similar to duration of lumbar sympathetic blockade, strengthening the link between pain relief and sympathetic denervation. In one study,
pain relief was accompanied by increased skin temperature and skin blood flow (venous occlusion plethysmography), whereas regional sweating and vasoconstrictor ice response were decreased (Fig. 39-14) (39). Another study indicated that nutritive blood flow was not increased, but pain was relieved in a high percentage of patients (50). Good results with relief of rest pain in the lower limbs have been reported by others (3,51,52). Very little data are available on upper limb vascular disease, although case reports indicate beneficial results with sympathetic block in vasospastic disorders such as those listed in Table 39-1.
pain relief was accompanied by increased skin temperature and skin blood flow (venous occlusion plethysmography), whereas regional sweating and vasoconstrictor ice response were decreased (Fig. 39-14) (39). Another study indicated that nutritive blood flow was not increased, but pain was relieved in a high percentage of patients (50). Good results with relief of rest pain in the lower limbs have been reported by others (3,51,52). Very little data are available on upper limb vascular disease, although case reports indicate beneficial results with sympathetic block in vasospastic disorders such as those listed in Table 39-1.
![]() Figure 39-15. Blood flow distribution after lumbar sympathetic blockade in arteriosclerotic patients at rest. Blood flow in the femoral artery (electromagnetic flow meter) is increased, as is skin blood flow (skin temperature); however, muscle blood flow (133Xe clearance) is reduced. Note that this is a widespread sympathetic block (both lower limbs), which may sometimes even reduce the skin blood flow through diseased vessels (see Fig. 39-19). From Cousins MJ, Wright CJ. Graft muscle skin blood flow after epidural block in vascular surgical procedures. Surg Gynecol Obstet 1971;133:59, with permission. |
It would appear that the primary benefits that result from sympathetic blockade are pain relief and improved healing of skin lesions; however, controlled studies of efficacy of the sympathetic block are required to achieve a better definition of its role in the treatment of vascular disease of the lower and the upper limbs.
Application for Pain Relief other than in Occlusive Vascular Disease
The pain-relieving effect of a sympathetic block in a patient with peripheral arterial disease is said to follow improved blood flow. Good pain relief often occurs, however, when no improvement in peripheral circulation can be noted (50); the possible mechanisms for this are discussed in the next sections (Fig. 39-12). The precise mechanism whereby the nervous system is involved in peripheral pain independent of effects upon flood flow have not yet been fully elucidated (11). It has been suggested that the cutaneous pain threshold, by means of a
negative feedback loop, is influenced by sympathetic efferents (Fig. 39-12) (53). Interruption of this feedback loop may explain the often remarkable pain relief seen after sympathetic blocks in patients with threatening gangrene, despite a lack of improvement in skin blood flow. It is possible that increased efferent sympathetic activity increases activity in nocireceptors by way of sympathetic fibers in proximity to sensory receptors. This is an explanation for pain relief that may follow stellate ganglion block in patients with arteriopathy (e.g., associated with scleroderma) in the upper limb, despite the lack of change in blood flow.
negative feedback loop, is influenced by sympathetic efferents (Fig. 39-12) (53). Interruption of this feedback loop may explain the often remarkable pain relief seen after sympathetic blocks in patients with threatening gangrene, despite a lack of improvement in skin blood flow. It is possible that increased efferent sympathetic activity increases activity in nocireceptors by way of sympathetic fibers in proximity to sensory receptors. This is an explanation for pain relief that may follow stellate ganglion block in patients with arteriopathy (e.g., associated with scleroderma) in the upper limb, despite the lack of change in blood flow.
![]() Figure 39-18. The blood flows through an artery (A) or its branches (B and C) are proportionate to the perfusion pressures and inversely proportionate to total and regional resistances, respectively. |
Table 39-2 Independent tests of sympathetic function, blood flow, and pain | |
---|---|
|

Full access? Get Clinical Tree
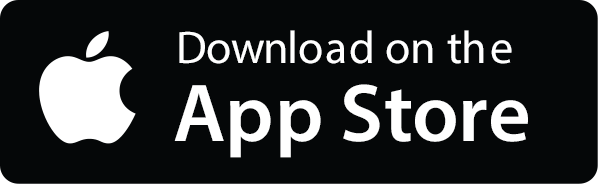
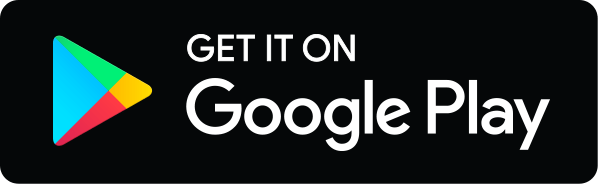