Superficial incisional SSI
Infection occurs within 30 days after the operation
•and infection involves only skin or subcutaneous tissue of the incision
•and at least one of the following:
–Purulent drainage, with or without laboratory confirmation, from the superficial incision
–Organisms isolated from an aseptically obtained culture of fluid or tissue from the superficial incision
–At least one of the following signs or symptoms of infection: pain or tenderness, localized swelling, redness, or heat and superficial incision is deliberately opened by surgeon, unless incision is culture-negative
–Diagnosis of superficial incisional SSI by the surgeon or attending physician
Deep incisional SSI
Infection occurs within 30 days after the operation if no implant is left in place or within 1 year if implant is in place and the infection appears to be related to the operation
•and infection involves deep soft tissues (e.g., fascial and muscle layers) of the incision
•and at least one of the following:
–Purulent drainage from the deep incision but not from the organ/space component of the surgical site
–A deep incision spontaneously dehisces or is deliberately opened by a surgeon when the patient has at least one of the following signs or symptoms: fever (>38°C), localized pain, or tenderness, unless site is culture-negative
–An abscess or other evidence of infection involving the deep incision is found on direct examination, during reoperation, or by histopathologic or radiologic examination
–Diagnosis of a deep incisional SSI by a surgeon or attending physician
Organ/space SSI
Infection occurs within 30 days after the operation if no implant is left in place or within 1 year if implant is in place and the infection appears to be related to the operation
•and infection involves any part of the anatomy (e.g., organs or spaces), other than the incision, which was opened or manipulated during an operation
•and at least one of the following:
–Purulent drainage from a drain that is placed through a stab wound into the organ/space.
–Organisms isolated from an aseptically obtained culture of fluid or tissue in the organ/space.
–An abscess or other evidence of infection involving the organ/space that is found on direct examination, during reoperation, or by histopathologic or radiologic examination.
–Diagnosis of an organ/space SSI by a surgeon or attending physician.
Every surgical incision, no matter the wound class, has bacteria that are present and can be cultured at the conclusion of the procedure. Inoculation of the surgical site occurs inevitably during surgery, either inward from the skin or outward from the tissues being operated on (e.g., surgery upon a hollow viscus). However, the presence of bacteria does not predict infection by itself, and other clinical parameters amplify microbial effects. The pathophysiology of SSIs is related to several factors, including the following: the inoculum, the presence of adjuvants (e.g., suture material, prosthetic devices, devitalized tissue, blood), bacterial virulence factors, the microenvironment of the surgical site (e.g., oxygenation, perfusion), antibiotic prophylaxis, and host factors.
The Inoculum
Surgical site infections are nearly always the consequence of contamination that occurs at the time of the operation, and infection is most closely linked to the number of bacteria that contaminate the surgical site. Classic studies have demonstrated that greater numbers of bacteria, the greater the likelihood of infection; the “magic number” of 105 organisms/g of tissue has been the commonly recognized threshold [7]. However, this threshold is not valid across all types of microorganisms or different strata of host responses and can be reduced dramatically by the presence of adjuvants, and therefore is not practical for routine monitoring or everyday decision making.
Contamination of the surgical site with bacteria occurs from multiple sources, including the patient’s skin, the organ or tissue being operated upon, airborne organisms, and even the surgical team. Wounds are classified by the amount of contamination of the incision into clean, clean-contaminated, contaminated, and dirty (Altemeier classification). Clean surgical procedures involve the skin and subcutaneous and musculoskeletal soft tissues (e.g., breast, hernia, orthopedic joint replacement procedures), and have the lowest incidence of SSIs. Clean-contaminated procedures involve the controlled opening of a hollow viscus, including the respiratory, genitourinary, or gastrointestinal tract. In contaminated procedures, bacteria are introduced into a normally sterile tissue or body cavity, but are not present for a long enough time to establish an infection (e.g., penetrating abdominal trauma, unintentional enterotomy, open extremity fractures). Dirty procedures are performed to control an established infection (e.g., colon resection for complicated diverticulitis), and have the highest rate of SSI. Contaminated and dirty procedures carry an independent risk of SSI.
Operative procedures that enter a normally colonized area of the body allow contamination of the surgical site with organisms that are specific to the area’s colonizing flora. Procedures involving only the skin and subcutaneous tissues result in lower inocula because the mechanical preparation of the surgical site should minimize the number of bacteria present. However, colonic bacterial density may exceed 1010 organisms/g of feces. Bacterial contamination occurs of the surgical site even with meticulous preparation and surgical technique. Hence, colon operations have clearly higher rates of SSI than inguinal hernia procedures. A classic technique to decrease SSIs following high-risk procedures is to leave the incision open at the original procedure and then allow the wound to heal by secondary intention or attempt delayed primary closure in a few days (usually on or after the fourth day). Small randomized trials have demonstrated that delayed primary closure decreases the SSI rate, but this tactic should only be employed in dirty or infected cases as it increases the time to wound closure and, if the wound cannot be closed, may subject the patient to delayed wound healing and prolonged postoperative care [8–10].
Bacteria and Virulence Factors
The microbiology of SSI depends on the nature of the operation. Most SSI are caused by gram-positive cocci that are commensal skin flora [11], including Staphylococcus aureus, coagulase-negative staphylococci (usually S. epidermidis), and Enterococcus spp.; antibiotic prophylaxis for most cases should emphasize coverage of these organisms. The likelihood of gram-negative bacilli causing SSI is increased after head and neck surgery (if pharyngo-esophageal structures are entered), thoracic surgery (if tracheobronchial or esophageal structures are entered), gastrointestinal surgery, or infra-inguinal surgery of any type. Colorectal surgery and surgery of the female genital tract are at risk for SSI caused by obligate anaerobic bacteria (e.g., Bacteroides fragilis), as well as some gram-positive colonists (e.g., Enterococcus spp., Streptococcus spp.). The biliary tract harbors Escherichia coli, Klebsiella spp., and enterococci. Any of these potential pathogens may contaminate the surgical site, and infection occurs commonly with microbes that are most likely to contaminate a given procedure.
Patients who are chronic carriers of S. aureus are at increased risk of SSI [12], and surgeons who harbor S. aureus have increased rates of SSI among their patients. The staphylococcal carrier state may afflict as many as 30% of normal individuals [13], but nearly all carriers are colonized with relatively sensitive strains. The methicillin-resistant carrier state has also been described but remains unusual (<5% of the US population) [13]. Topical 2% mupirocin ointment applied to the nares of patients who are chronic carriers of S. aureus may reduce the increased incidence of SSI that is characteristic of chronic staphylococcal carriage, and may be useful in patients with previous methicillin-resistant Staphylococcus aureus (MRSA) infection [14], but is ineffective when applied to the general population. In a recent randomized trial of cardiac surgery patients, decontamination of the nasopharynx and oropharynx with 0.12% chlorhexidine gluconate reduced significantly the proportion of patients with S. aureus nasal colonization (p < 0.001) [15]. Topical chlorhexidine reduced the overall postoperative infection rate by 6.4% (95% confidence interval [CI] 1.1–11.7%, p < 0.01), lower respiratory tract infections by 6.5% (95% CI 2.3–10.7%, p < 0.01), and the risk of deep incisional SSI by 3.2% (95% CI 0.9–5.5%, p < 0.01). The benefit of pharyngeal decontamination has not been replicated in abdominal surgery.
Virulence factors vary among bacterial species, such that organisms with more potent virulence characteristics may require smaller inocula to cause infection. Virulence factors include structural components of the bacterial cell, secreted products of bacteria, and resistance of bacteria to antibiotics that shield the pathogen from prevention and treatment. The prototypical virulence factor of gram-negative bacteria is lipopolysaccharide (LPS), or endotoxin. Endotoxin is located in the outer membrane of gram-negative bacteria, and contains a potent Lipid A moiety that stimulates many components of the innate and adaptive host responses [16]. It is toxic to host tissues and generally enhances virulence by activating several mediator cascades. The Enterobacteriacae are the most common endotoxin-associated bacteria.
Other bacterial species elaborate specific virulence features. The peptidoglycans of gram-positive bacterial cell walls activate host immunity in a manner analogous to LPS. Gram-positive cocci also elaborate exotoxins and numerous proteases that destroy tissue and facilitate invasion. Streptococcus pyogenes has a cell surface M-protein capsule that resists phagocytosis by neutrophils [17]. A number of different M-proteins exert differential effects upon virulence; strains lacking the M-protein component lose the potential to cause infection [18]. Bacteroides fragilis has a polysaccharide capsule that retards phagocytosis [19]. This capsular material appears to still incite abscess formation even when the bacterium itself has been heat-killed. Because Bacteroides spp. are obligate anerobes, they are usually associated in a synergistic relationship to cause infection with aerobic pathogens (well documented, in the case of enterococci), which consume oxygen from the microenvironment and thereby promote strict anaerobiosis.
Other virulence factors are exotoxins, products secreted by gram-positive bacteria. For example, S. aureus elaborates the exotoxin coagulase [20]. Coagulase activates the coagulation cascade and creates a fibrin-rich local environment in soft tissue, which protects the organism from phagocytosis by host neutrophils. Another much-discussed exotoxin is the Panton–Valentine leukocidin (PVL), which is associated with community-associated methicillin-resistant S. aureus (CA-MRSA) [21]. The PVL exotoxin is a potent leukocidin. Interplay among the bacteria, the exotoxin, and the liquefactive necrosis of neutrophils results most commonly in a dramatic local soft tissue infection with a black central eschar (Fig. 11.1). Infection with this organism will likely be observed with SSIs in addition to community-acquired soft tissue infections [22]. Other examples of exotoxins include streptococcal superantigens associated with necrotizing soft tissue infections and toxic shock-like syndrome [23]. Toxic shock toxins produced by some strains of S. aureus may cause a fulminant shock syndrome associated with surgical packing in open wounds [24].
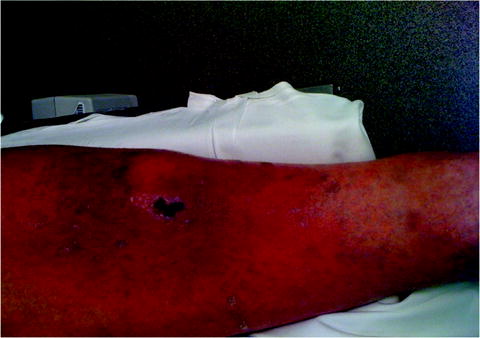
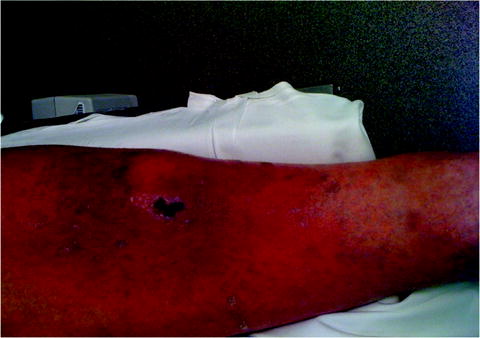
Fig. 11.1
A local soft tissue infection with a black central eschar
Antibiotic resistance should also be considered a virulence factor, as the increasing prevalence of resistant organisms in both the community and the hospital become a larger problem in SSI prevention and management. Beta-lactamase production has reduced dramatically the susceptibilities of vast numbers of bacteria. Mutations change the bacterial cellular phenotype with changes in porin proteins [25] and other structural changes of the cell that mediate resistance. Carbapenemase activity has been detected in a variety of organisms, including E. coli, Acinetobacter baumannii, K. pneumoniae, and other Enterobacteriaceae. Of note, MRSA, although usually not resistant per se to vancomycin, may be associated with treatment failures due to inability to reach high-enough concentrations when the minimum inhibitory concentration (MIC) exceeds 1 mcg/mL. Isolates of MRSA with vancomycin MIC 1 mcg/mL are at high risk for treatment failure, and MRSA with MIC ≥ 2 mcg/mL should be treated routinely with an alternative agent to vancomycin because bactericidal drug concentrations cannot be achieved with nontoxic doses [26, 27].
Microenvironment of the Surgical Site
The foundation of any strategy to decrease the risk of SSI is proper patient preparation and sound surgical technique [28]. Disruption of skin integrity provides a portal of entry for pathogenic organisms and represents the most important etiologic factor for most skin and soft tissue infections [29]. Local factors predisposing to infection within the surgical incision also affect the ability of bacteria to overcome host defenses. Local factors predisposing to infection include soft tissue trauma (including thermal injury from exuberant use of electrocautery), animal or human bites, burns, wound contamination, diminished perfusion (e.g., peripheral vascular disease), obesity, poor hygiene, presence of a foreign body, or venous insufficiency and stasis [30].
Surgeons have attempted to decrease the inoculum size at the site of surgery by hair removal and antiseptic showering. The routine removal of hair has been questioned as a potential cause of SSIs by causing unnecessary breaks in the skin (by shaving in particular). Several randomized controlled trials (RCTs) have examined hair removal with clipping, shaving, or depilatory agents, and no hair removal. In a meta-analysis, shaving with razors increased SSIs over clipping (relative risk [RR] 2.02, 95% CI 1.21–3.36) or depilatories (RR 1.54, 95% CI 1.05–2.24) [31]. By contrast, neither dispensing with hair removal altogether nor hair removal immediately prior to surgery had an effect on SSIs. Antiseptic showering has been studied in two prospective, randomized, controlled trials [32, 33]. Although bacterial counts were reduced, there was no lowering of the rate of SSI in those who showered with the antiseptic. Showering might be useful in certain high-risk patients or where the institutional SSI rate is high; if done they should be repeated, as cumulative benefit may accrue.
Surgeon hand antisepsis has been studied as a potential way to decrease SSIs, whether by alcohol hand-rub or traditional surgical scrub techniques with soap and water. Most studies have examined bacterial load (colony-forming units, CFUs) as the endpoint rather than the clinical endpoint of SSI [34]. One equivalence study, which examined SSI rates as the primary endpoint after aqueous alcohol hand rub versus traditional hand scrub in 4,387 patients [35], found no difference in SSI rates between groups (hand-rubbing 2.44% versus hand-scrubbing 2.48%, for a difference of 0.04%, 95% CI −0.88% to +0.96%). Compliance is better and cost is lower for hand-rubbing solutions [35, 36], which is now the primary method of hand antisepsis for many centers. Chlorhexidine–alcohol preparations for hand scrubbing are superior to povidone–iodine for reducing CFUs recovered from skin.
Chlorhexidine–alcohol also appears to be superior to iodine-based preparations for patient skin preparation. A RCT of preoperative skin preparation with chlorhexidine–alcohol scrub versus povidone–iodine in clean-contaminated surgery found that the rates of superficial and deep incisional SSIs were significantly lower in the chlorhexidine–alcohol group (4.2% versus 8.6% for superficial SSIs, p = 0.008; 1% versus 3% for deep incisional SSIs, p = 0.05) [37]. There was no difference in organ-space SSIs. A meta-analysis, which included the aforementioned study in addition to eight other randomized controlled trials comparing povidone–iodine and chlorhexidine (n = 3,614 patients), concurred with these observations [38]. A cost–benefit model in the same study estimated a net cost savings of $16–26 per case [38].
Incisional hematoma is another important local risk factor for SSIs. In addition to being impenetrable to neutrophils, hemoglobin is a rich source of ferric iron, making the hematoma a fertile environment for bacterial growth [39]. Foreign bodies provide a nonbiological surface for adherence of microbes, and pose a functional challenge to phagocytosis. In addition, foreign bodies such as mesh, drains, and other implants are potential nidi for infection and formation of difficult-to-treat biofilms. The classic studies of Elek and Conen [40] have demonstrated the dramatic reduction in inoculum size that is required to cause soft tissue infection when a mere silk suture is present. Tissue ischemia or damage from electrocautery becomes a haven for contaminants from the surgical procedure. Dead space within the incision accumulates serum and provides a locale where microbes are inaccessible by neutrophils. These adjuvant effects enhance microbial proliferation, reduce the inoculum necessary, and inhibit host defenses, which in turn result in clinical infection.
Antibiotic Prophylaxis
Antimicrobial prophylaxis is a key step in the prevention of SSI, but not a panacea. Prophylaxis was shown by Polk and Lopez-Mayor in their seminal 1969 RCT to reduce the SSI rate following colectomy from more than 30% to 5% [41]. However, prophylaxis only protects the incision itself, and only during the period of time when it is open. If not administered properly, antibiotic prophylaxis is ineffective and puts the patient at risk for other complications, such as drug reactions or superinfection such as Clostridium difficile infection (CDI). Four principles guide the administration of the appropriate antimicrobial agent for prophylaxis: (1) safety, (2) an appropriate narrow spectrum that covers relevant pathogens, (3) little or no reliance upon the agent for therapy of infection so that resistance is not promoted, and (4) administration within 1 h before surgery and for a defined, brief period of time thereafter (ideally a single dose, but no more than 24 h) [42]. According to these principles, fluoroquinolones or carbapenems should not be used for surgical prophylaxis. However, in prophylaxis for colon surgery, fluoroquinolones have been endorsed by the Surgical Care Improvement Project (SCIP) for penicillin-allergic patients, and ertapenem has also been recommended [43].
Antibiotic prophylaxis is indicated for most clean-contaminated and contaminated (or potentially contaminated) operations. An example of a clean-contaminated operation where antibiotic prophylaxis is usually not indicated is elective laparoscopic cholecystectomy [44–46]. Three separate meta-analyses have been performed examining antibiotic prophylaxis for elective laparoscopic cholecystectomy [44–46]. The most recent of these, by the Cochrane collaboration, included 11 randomized clinical trials (1,664 patients) at low anesthesia risk, with few comorbidities and low risk of infection. There was no difference between antibiotic prophylaxis and no prophylaxis in the proportion of SSIs (odds ratio [OR] 0.87, 95% CI 0.49–1.54), or extra-abdominal infections (OR 0.77, 95% CI 0.41–1.46) [46]. However, antibiotic prophylaxis is indicated for high-risk biliary surgery; high risk is conferred by age >70 years, diabetes mellitus, or a recently instrumented biliary tract (e.g., bile duct stent).
Elective colon surgery is a clean-contaminated procedure where preparatory practices are in evolution [47, 48]. The use of antibiotic prophylaxis in colorectal surgery has been shown consistently to be beneficial in RCTs when the antibiotic is administered prior to the incision and the regimen demonstrates appropriate activity against colonic flora [41, 49–52]. A Cochrane meta-analysis of prophylaxis of colorectal surgery reported results of 182 trials, including 11 RCTs comparing any regimen to placebo, clearly showing a decrease in risk of SSI with any appropriate prophylaxis regimen (RR 0.30, 95% CI 0.22–0.41) [53]. However, there is no consensus as to which regimen is best. Oral antibiotic regimens, standardized in the 1970s by the administration of nonabsorbable neomycin and erythromycin base in addition to mechanical cleansing, reduced the risk of incisional SSI further to its present rate of approximately 4–8% [48]. Currently, oral antibiotic prophylaxis is often omitted, due to the belief that no additive benefit exists beyond parenteral antibiotic prophylaxis. Current SCIP guidelines for antibiotic prophylaxis of elective colon surgery recommend regimens including oral prophylaxis alone, parenteral prophylaxis alone, or the combination [47]. However, oral prophylaxis alone likely is not as effective as parenteral prophylaxis. Song and Glenny [54] examined oral antibiotics alone compared with oral/systemic antibiotic prophylaxis (five trials), and found a higher SSI rate with oral prophylaxis alone (OR 3.34, 95% CI 1.66–6.72), although the CI was wide. However, there may be benefit to the combined use of oral and parenteral prophylaxis; Lewis et al. performed a meta-analysis of 13 RCTs of systemic versus combined oral and systemic prophylaxis, and showed significant benefit for the combined approach (RR 0.51, 95% CI 0.24–0.78) [48]. Mechanical bowel preparation in colon surgery is also currently controversial. A 2009 Cochrane meta-analysis of 13 RCTs compared bowel preparation to no bowel preparation for 4,777 participants undergoing elective colorectal surgery. Patients received no benefit from mechanical bowel preparation, as the rates of anastomotic leak and SSI were similar in both groups [55].
Antibiotic prophylaxis of clean surgery has been also been controversial. Where bone is incised (e.g., craniotomy, sternotomy) or a prosthesis is inserted, antibiotic prophylaxis is generally indicated. Some controversy persists with clean surgery of soft tissues such as breast or hernia. Meta-analysis of RCTs shows some benefit of antibiotic prophylaxis of breast cancer surgery without immediate reconstruction [56, 57]. The use of antibiotic prophylaxis for elective open groin hernia surgery with or without mesh has also been controversial. A variety of small studies have been performed which showed no benefit of antibiotics [58–60]. However, a 2007 meta-analysis suggested that there may be a benefit to antibiotic prophylaxis in elective groin hernia repairs if mesh is used, with infection rates of 1.4% and 2.9% in the prophylaxis and control groups, respectively (OR 0.48, 95% CI 0.27–0.85). If mesh was not used, the difference was less pronounced (3.5% versus 4.9%, OR 0.71, 95% CI 0.51–1.00) [61].
Arterial reconstruction with prosthetic graft is an example of clean surgery where the antibiotic prophylaxis is warranted because the susceptibility to infection is high. These cases are high-risk due to the presence of ischemic tissue and the infra-inguinal location of many incisions. One recent meta-analysis [62] identified 35 RCTs for prevention of infection after peripheral arterial reconstruction, with 23 specifically examining prophylactic systemic antibiotics. Prophylaxis reduced the risk of SSI by approximately 75%, and early graft infection by about 69%. There was no benefit to prophylaxis for more than 24 h, of antibiotic bonding to the graft material itself, or preoperative bathing with an antiseptic agent compared with un-medicated bathing. Placement of closed suction drains also does not decrease the rate of SSIs [63].
Given that most SSIs are caused by gram-positive cocci, the antibiotic chosen should be directed primarily against staphylococci for clean cases and high-risk clean-contaminated biliary and gastric surgery. A first-generation cephalosporin is preferred almost always; clindamycin may be used in cases of penicillin allergy [42]. If gram-negative or anaerobic coverage is required, a second-generation cephalosporin or a combination of a first-generation agent plus metronidazole is the regimen of first choice. Single agent prophylaxis is often preferred in institutions due to ease of administration. Vancomycin prophylaxis is generally appropriate only in institutions where the incidence of MRSA infection is high (>20% of all SSIs caused by MRSA) or if a patient is a known carrier of MRSA.
Parenteral antibiotic prophylaxis should be given within 30–60 min prior to incision [64]. Antibiotics given sooner are ineffective, as are agents that are given after the incision is closed. However, compliance with this guideline in the USA has been poor. A 2001 nationwide audit of prescribing practices in the USA indicated that only 56% of patients who received prophylactic antibiotics did so within 1 h prior to the skin incision [42]; timeliness was documented in only 76% of cases in a 2005 audit in US Department of Veterans Affairs (VA) hospitals [43]. Most inappropriately timed first doses of prophylactic antibiotic occur too early [42, 43]; changing institutional processes to administer the drug in the operating room can improve compliance with best practices [43]. Even though SCIP specifies a 24-h limit for prophylaxis, preoperative single-dose prophylaxis (with intraoperative re-dosing, if indicated) is equivalent to multiple doses for the prevention of SSI [65]. Antibiotics with short half-lives (e.g., cefazolin or cefoxitin) should be re-dosed every 3 (for cefoxitin) to 4 h (for cefazolin) during surgery if the operation is prolonged or bloody [66]. As SCIP reporting becomes linked to hospital payments, compliance with these guidelines has improved, but there is no definitive evidence that SSI rates have declined as a result.
Prolonged antibiotic prophylaxis is both pervasive and potentially harmful. Antibiotics should not be given to “cover” indwelling drains or catheters, in irrigation fluid, or as a substitute for poor surgical technique. As a result of ischemia caused by surgical hemostasis, antibiotic penetration into the incision immediately after surgery is questionable until neo-vascularization occurs (24–48 h). However, recent US data show that only 40% of patients who receive antibiotic prophylaxis do so for less than 24 h [42]. Clostridium difficile infection follows disruption of the normal balance of gut flora, resulting in overgrowth of the enterotoxin-producing C. difficile [67]. Although virtually any antibiotic may cause CDI (even a single dose), prolonged antibiotic prophylaxis increases the risk. Prolonged prophylaxis also increases the risk of nosocomial infections unrelated to the surgical site, and encourages the emergence of multi-drug-resistant (MDR) pathogens. Both pneumonia and vascular catheter-related infections have been associated with prolonged prophylaxis [68, 69], as has the emergence of SSI caused by MRSA [70].
Host Factors
In the majority of procedures, the host manages effectively the inoculum of bacteria and all of the other parameters that favor infection. It is almost certain that genetic variability among patients leads to increased susceptibility to infection: an intrinsic difference in the capacity to generate an inflammatory response, differences in phagocytic efficiency, or differences in specific immune response. Whereas the exact mechanisms of host defense impairment are not defined, it is clear that each host variable is impactful in some way on the probability of infection. However, quantifying the risk of individual patients and the risk associated with each variable has remained elusive.
The relationships among the surgical site, microbes, and host defenses describe the overall risk of SSI. Acquired problems of host defense have been associated by statistical inference in subpopulations of patients that appear to have higher rates of SSIs than others. These variables include age, obesity, corticosteroids, systemic chronic illness, or immunocompromised states, malnutrition, low serum albumin concentration, tobacco smoking, uncontrolled diabetes mellitus, and ischemia secondary to vascular disease or irradiation [71–79]. Scott et al. showed that age and albumin concentration were most predictive of SSI in a cohort of more than 9,000 patients from a single community hospital [80]. A low serum albumin concentration is a surrogate marker for a wide range of comorbid conditions that render the patient immunocompromised or impaired nutritionally. Patients who are malnourished should be considered for preoperative enteral feeding or, if necessary, total parenteral nutrition (TPN) if extensive surgery is planned. The Veterans Affairs Cooperative study on preoperative nutritional support demonstrated fewer complications other than catheter-related infections in those patients who were malnourished and given an average of 9 days of preoperative TPN [81]. More recently, supplemental enteral nutrition reduced the risk of SSI in malnourished patients [82, 83]. Impaired lymph flow may also be a potential risk factor for SSI, with axillary or inguinal lymphadenectomy. These are now used less commonly for the regional treatment of cancer, having been supplanted by sentinel node biopsy. Transitory physiological states may also increase the risk of SSI; examples include severe injury, shock, blood transfusion, hypothermia, hypoxia, and hyperglycemia.
The length of the preoperative hospital stay was considered historically a risk factor for SSI, but has become less so as outpatient surgery is performed increasingly. Bacterial colonization with often-MDR nosocomial organisms occurs routinely within 72 h of hospitalization. Such patients should have their microbiologic history reviewed prior to an operation so that a rational decision can be made about the choice of agent for prophylaxis. In patients who have had infections with MDR microbes, antibiotics should be used that are potent against the bacteria demonstrated previously. Existing infection should be treated before elective surgery is undertaken, as the presence of a concomitant infection even at a remote site increases the risk of SSI. Colonization by MRSA often leads to infection by the same organism, especially in elderly patients, dialysis patients, and nursing home residents. These patients should be given an antibiotic active against MRSA for prophylaxis. Use of agents with treatment efficacy against MRSA may also be helpful in institutions with a high rate of MRSA SSIs.
Risk Stratification for Surgical Site Infections
It is important to consider these factors (bacteria, host, and surgical site factors) to stratify an individual’s risk for SSI. The CDC guidelines formulate the risk of SSI as the size of the bacterial inoculum (contamination) multiplied by bacterial virulence, the product being divided by host resistance; however, this is not of much use clinically [84]. The Study on Efficacy of Nosocomial Infection Control (SENIC) assessed the effectiveness of various measures in the years 1976–1988 [85]. Four parameters were independent markers of a higher risk of SSI: Abdominal surgery, surgery lasting longer than 2 h, a contaminated or dirty procedure, and more than three diagnoses at the time of discharge. This predictive method proved to be more accurate than the long-standing practice of surgeons of making risk predictions on the basis of their clinical experience. A similar attempt to identify factors predictive of SSI was the National Nosocomial Infection Surveillance (NNIS) study [86–88], begun in 1970 and continuing to the present time as the National Healthcare Safety Network (NHSN). This risk factor index score remains in wide usage. The three important factors identified were an American Society of Anesthesiologists (ASA) score of 3 or more on a 5-point scoring system, a contaminated or dirty procedure, and an operation lasting longer than the 75th percentile of the average duration for that procedure. The NNIS system may be used to formulate a risk categorization index ranging from 0 to 3, where 1 point each is assigned for an ASA score of 3 to 5 points, a contaminated or dirty incision, and prolonged operating time [87, 88]. The risks for SSI associated with different index scores are: 0 = 1.5%; 1 = 2.9%; 2 = 6.8%; and 3 = 13% [87]. A modification subtracts one point for laparoscopic procedures, so that the final index score ranges from −1 to 3 points [89].
Diagnosis
Despite their often nonspecific appearance and paucity of symptoms, SSIs remain a clinical diagnosis based on history and physical examination. Infection is the result of microbial proliferation in tissue, which in turn activates the inflammatory cascade. This local inflammatory response produces the classic clinical findings of rubor (erythema), dolor (pain), calor (heat), and tumor (swelling). These manifestations of inflammation provide the physical evidence for the diagnosis of infection, and are often accompanied by increasing wound pain, lack or stagnation of clinical recovery, or malaise. Surgical control of the infectious source remains the crucial diagnostic and therapeutic maneuver. Often times, this can be achieved by simply opening and draining the infected incision in superficial incisional SSIs. However, deep incisional SSIs may require thorough surgical debridement and open wound care to resolve the infectious process, whereas organ/space SSIs usually require percutaneous drainage of formal intracavitary reoperation. In select cases, a small intracavitary abscess (<5 cm diameter) may respond to antibiotic therapy alone, but the clinical response must be adjudged with diligence.
The presenting complaints depend on the depth of infection. Typical complaints include localized pain that worsens upon physical activity and contact to the affected area. Instead of steady clinical improvement postoperatively, patients with a developing SSI often begin to worsen clinically on postoperative day 4 or 5. Rare early SSIs include infections caused by beta-hemolytic streptococci or Clostridium spp., and may manifest as early as postoperative day 1 or 2. Streptococcal infections cause pain and local wound erythema, whereas clostridial infections drain a grey-colored thin fluid and lack the characteristic inflammatory skin changes.
The incision itself is usually the source of increasing pain and erythema prior to the discharge of pus. The presenting symptoms may appear out of proportion to the clinical findings, especially in deep or necrotizing infections [90]. Probing the wound after partial removal of sutures is simple and allows diagnosis and treatment of a superficial incisional SSI. Alternatively, if the infection only involves the organ/space, symptoms specific to that body cavity will usually predominate, such as prolonged postoperative ileus, persistent respiratory distress, neurologic deficit, or altered mental status.
Culturing the wound by the swab method has been shown experimentally to be reliable. Recovery of 105 CFU of bacteria/g of tissue or mL of fluid has been the traditional indicator of local infection, whether of the lung, the urinary tract, or the surgical incision [91]. Intraoperative cultures of high-risk incisions may also be considered, and the results may be used later to guide treatment; these cultures must not be utilized as justification to prolong prophylaxis. In a study of 52 open fractures, patients with intraoperative quantitative bacterial counts >105 CFU/mL had an SSI rate of 50%, whereas patients with <105 CFU/mL had an SSI rate of 5% [91]. Among patients who underwent abdominal hysterectomy, recovery of >104 CFU/mL from pelvic fluid was associated with a subsequent SSI rate of 42%, whereas only 12% of patients had an SSI when <103 CFU/mL were recovered at operation [92]. In the case of deep incisional SSIs, tenderness may extend beyond the margin of erythema, or crepitus and cutaneous vesicles or bullae may manifest [93]. With ongoing infection, signs of systemic inflammation such as fever, tachycardia, hypotension, and altered mental status manifest the clinical picture of sepsis/severe sepsis (sepsis with organ dysfunction). These clinical findings usually portend a worse clinical prognosis and are often observed with more severe infections that will require surgical intervention in order to resolve.

Full access? Get Clinical Tree
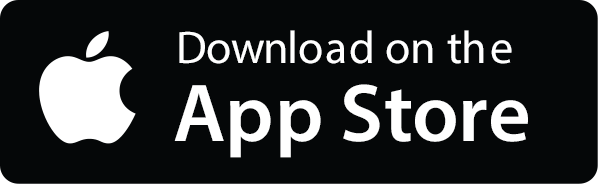
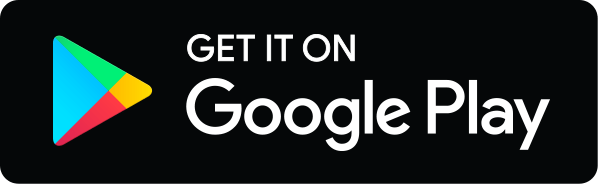