SAH may be further defined as subarachnoid blood on computed tomography (CT) scan, blood or xanthochromic cerebrospinal fluid (CSF), or red blood cells in the final tube of CSF sample and positive angiography findings (Perry et al., 2013). Prehospital care is critical and includes basic care for airway, breathing, and circulation (ABCs) and triage to deliver the patient to the nearest medical center with neurological/neurosurgical services including CT scan, angiography, neurosurgical consultation, and comprehensive care from emergency department (ED) through rehabilitation. Large, tertiary medical centers are often regional treatment centers and have the specialized interprofessional health care teams to accept aSAH patients as the need arises (Zebian, Kulkami, & Kazzi, 2013).
RISK FACTORS
The incidence of aSAH increases with age, and females are affected more than males particularly in the 45- to 55-year age group when the risk is nearly doubled (Ganti et al., 2013). Studies have demonstrated a regional variance with aSAH, with an increased incidence among the Japanese and Finnish compared to more southern regions closer to the equator. The incidence is doubled in African Americans compared to Caucasians. In addition to age and ethnic background, risk factors for aSAH include smoking, cocaine abuse, excessive alcohol consumption, and hypertension (Bautista, 2012). Other risk factors for aSAH include family history, genetics, or congenital conditions. Marfan syndrome, Ehlers-Danlos disease, neurofibromatosis type I, and autosomal dominant polycystic kidney disease are among the collagen vascular diseases that may predispose a person to aneurysm formation (Patel & Samuels, 2012).
Although the population is aging, the elderly are in better health and have longer life expectancies, thus the number of elderly patients with aSAH is increasing. A recent review found that the annual incidence of aSAH in people older than 70 years of age has exceeded a rate of 25 per 100,000 people. Despite the age and regional variations, aSAH is a neurosurgical emergency that carries high morbidity and mortality. Research indicates a mortality rate of approximately 50% within a month of the event, with the remaining 50% suffering from disabling functional and cognitive deficits (Washington et al., 2011).
Older age (>60 years), cigarette smoking, female gender, posterior circulation location, atherosclerosis, hypertension, and larger IA size (>5 mm) are contributing factors for an IA to rupture. A family history of IA or SAH, previous history of SAH, or the presence of autosomal dominant polycystic kidney disease increases the risk of IA rupture (Washington et al., 2011). However, studies in animal models and preclinical subjects have demonstrated that inflammation and the complement system activation may have a role in IA formation and rupture (Abate & Citerio, 2014).
PATHOPHYSIOLOGY
IAs are generally found at vessel bifurcations within the circle of Willis. The most common locations are at the bifurcation of the basilar artery, at the junction of the ipsilateral posterior inferior cerebellar artery (PICA) and vertebral artery (VA), or on the anterior communicating artery. The hemodynamic changes caused by increased blood pressure and other risk factors promote changes in the vessels and contribute to the formation and rupture of an IA (Penn, Komotar, & Connolly, 2011). In addition, endothelial dysfunction is thought to be the result of hemodynamic stress that leads to the inflammatory response in the arterial walls. This endothelial dysfunction ultimately results in vascular remodeling and cell death. Macrophages and apoptosis of smooth muscle cells in conjunction with further degradation of the extracellular matrix contribute to the progressive weakening of the arterial wall (Chalouhi, Hoh, & Hasan, 2013). Heavy alcohol use with its effects on blood pressure and hemodynamic factors is considered an independent risk factor for aSAH. Cocaine and similar drug abuse increases the risk of aSAH due to the strong vasoconstrictor effects and the resulting extreme hypertension (Diringer, 2009) (Fig. 8-1).
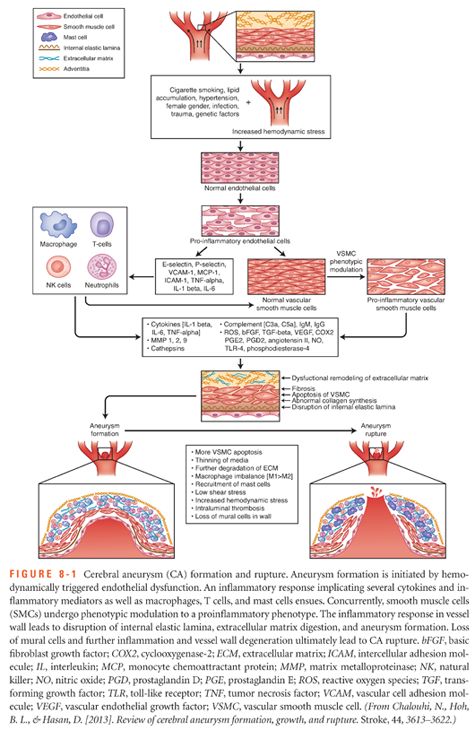
GRADING SCALES
A number of grading scales are used in practice to standardize the clinical classification of patients with SAH and to monitor progression and change in condition. These scales are based on the initial clinical neurological examination and the appearance of blood on the initial head CT. These scales include the Glasgow Coma Scale, the Hunt and Hess grading scale, the WFNS scale, the Fisher grading scale, and the modified Fisher grading scale. The Glasgow Coma Scale (GCS), introduced in 1974, was designed as a reliable and objective scale of neurological function in three subscales of level of consciousness, eye opening, and motor function (Teasdale & Jennett, 1974; Teasdale, Murray, Parker, & Jennett, 1979) (Table 8-1). The patient is assessed against the criteria of each scale, and the resulting points are added together to give a patient score between 3 and 15. In interpreting the score, the higher score correlates to a better patient’s condition. The GCS is used for initial and ongoing assessment of a patient with possible or confirmed cerebral trauma to determine neurological deficits and any changes in neurological condition over time.
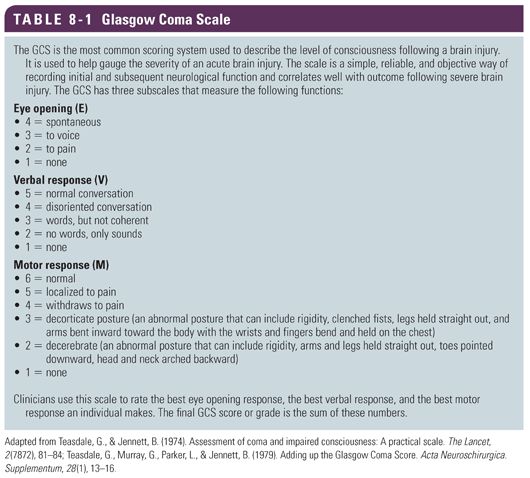
The World Federation of Neurosurgical Societies (WFNS) developed the WFNS grading scale, which describes the clinical presentation of SAH patients. The scale combines consciousness and motor deficit in its scoring system. The WFNS grading system uses a combination of consciousness and motor deficits from the GCS and presence of focal neurological deficits to grade the severity of SAH (Teasdale et al., 1988) (Table 8-2). The scale is useful for predicting recovery and response to treatment. For example, elderly patients who have a WFNS score of I to III have the best clinical recovery and seemed to benefit from aggressive treatment when compared to patients younger than age 70 years with a WFNS score of IV or V (Schöller et al., 2013).
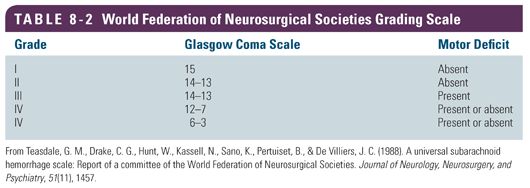
The Hunt and Hess grading scale, introduced in 1968, helps to classify the severity of an SAH based on the patient’s clinical condition. It is used as a predictor of a patient’s prognosis/outcome; higher score (grade) correlates to a lower survival rate (Hunt & Hess, 1968) (Table 8-3).
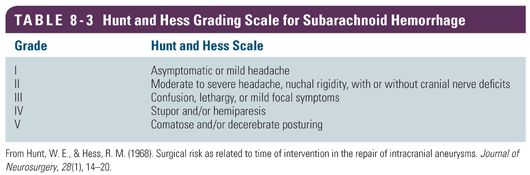
SIGNS AND SYMPTOMS
aSAH usually occurs without warning as a sudden cataclysmic event that changes the lives of the patient and the family. This hemorrhagic stroke is a neurosurgical emergency and often described as devastating, catastrophic, and often fatal. Clinical signs and symptoms may range from a slight headache and nuchal rigidity to coma and death. Patients presenting with IA rupture often complain of the “worst headache of my life” or a thunderclap headache and may have associated nausea and vomiting (often projectile), photophobia, and nuchal rigidity. Depending on the size of the rupture, neurological deficits may be present and range in severity from a focal deficit to coma. The presence of an unruptured IA or increased intracranial pressure (ICP) postrupture may cause cranial nerve palsies from compression on the third and/or sixth cranial nerve (CN III, CN VI). Some patients experience seizures with IA rupture (Diringer, 2009).
aSAH may be classified using scales including the GCS, the WFNS scale, and Hunt and Hess grading scale, which reflect the clinical condition of the patient. The Hunt and Hess grading scale and the WFNS scale are predictors of outcomes, with the higher the score, the poorer the prognosis (Green, Burns, & DeFusco, 2013). The Hunt and Hess grading scale is often shown in conjunction with the WFNS scale because they correlate SAH grades with the GCS. Table 8-4 provides a comparison of these scales with patient survival (Rosen & Macdonald, 2005). However, the Fisher grading scale is a numerical scale from 0 to 4 that provides insight to the amount of subarachnoid blood on CT scan (Table 8-5). These grading scales demonstrate a direct link to the severity of the hemorrhage, the clinical grade of the IA, and incidence of vasospasm after the SAH. In comparison, the modified Fisher grading scale provides more specific descriptions of the amount and location of blood seen on CT scan. Table 8-6 shows a comparison of the Fisher and modified Fisher grading scales (Claassen et al., 2001). The percentages associated with each grade on the modified scale are slightly higher for symptomatic vasospasm.
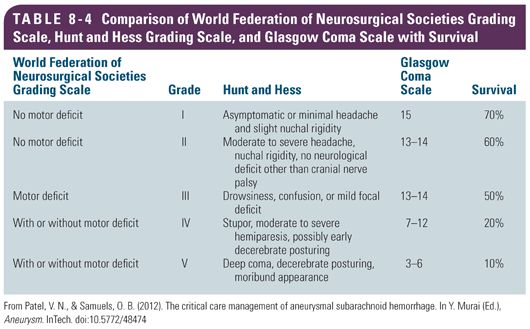
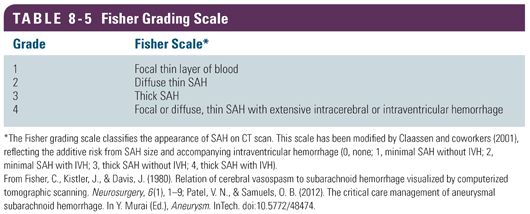
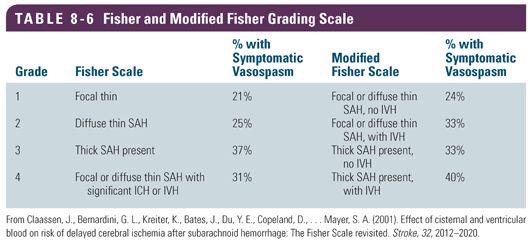
Clinical Pearl: All patients having signs and symptoms of stroke should be transported to the nearest primary stroke center or hospital with a similar designation for the available acute therapeutic interventions (American Heart Association/American Stroke Association). Patients with SAH may require transport to the nearest comprehensive stroke center to receive specialized care from vascular neurologists and neurosurgical and neurocritical care teams.
DIAGNOSTICS
Some patients may have a “warning” or sentinel headache that occurs hours to weeks before the SAH. Accurate diagnosis and early identification of a sentinel headache decreases morbidity and mortality related to SAH (Zebian et al., 2013). Approximately 2% of all ED admissions are for complaint of headache. Making the diagnosis of SAH in a patient with headache and a new neurological deficit is a straightforward process, but the diagnostic algorithm for headache alone without a neurological deficit becomes more challenging because the provider must decide which alert, neurologically intact patient needs further investigation. Half of all SAH patients present in this manner. Basic CT scan (without contrast) of the brain is performed, followed by a lumbar puncture (LP) if the CT scan is negative and SAH is suspected. The side effect of an LP may result in a headache that is worse than the original headache (Perry et al., 2013). Digital subtraction angiography (DSA) has been considered the preferred method for diagnosing intracranial IAs for many years. Improvements in CT technology, that is, computed tomography angiography (CTA), have been integrated into the diagnosis and treatment algorithms for patients with SAH in many neurological centers in Europe and the United States as the CT technology has improved dramatically (Abate & Citerio, 2014). Magnetic resonance imaging (MRI) may be used for diagnosis but is not considered a first-line option because the length of time to complete the examination is much longer that CT or CTA. Patient limitations such as cardiac pacemakers or other metallic-based implants may preclude the use of MRI (Patel & Samuels, 2012).
When a patient is being considered for either an endovascular or surgical approach for the treatment of an IA, a number of diagnostics are ordered to assist with identification of treatment options and decision making about the best approach for the particular needs of the patient. An angiogram is performed prior to surgical clipping to determine the location and size of the IA. Additional imaging studies may be conducted to more fully appreciate the characteristics of the IA. CT scan (with and without contrast), CTA, and DSA may be ordered preoperatively (Fig. 8-2). MRI is usually not one of the first-line examinations due to availability, cost, and the inability to distinguish hemorrhage. MRI may be useful for patients who are several days post-bleed or to identify small infarcts (American Association of Neuroscience Nurses [AANN], 2009). However, one small study compared susceptibility-weighted imaging (SWI) with standard CT techniques and fluid attenuated inversion recovery (FLAIR) to indicate SAH. The researchers found that by using a combination of SWI and FLAIR, MRI was able to detect SAH at higher rates than with CT alone (Verma et al., 2013). The neurosurgeon determines the surgical approach based on the location of the IA, with the main treatment options including clipping of an aneurysm or endovascular obliteration of the aneurysm. Clipping an IA is a major neurosurgical procedure that requires a craniotomy and generally lasts several hours. Research has shown that when a patient is anesthetized for long periods of time, there is an increased risk of complications due to physiological compromise and suppression of the immune system (Fox & Choi, 2009).
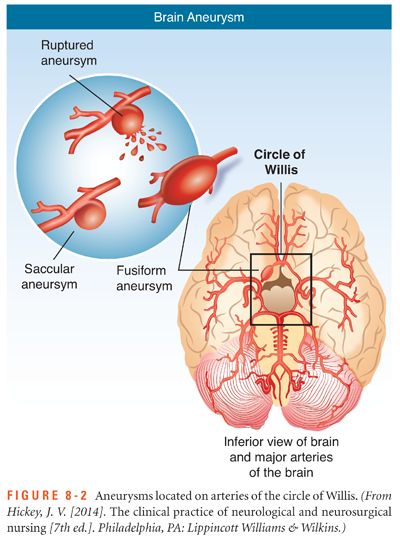
Positioning the patient for the procedure requires meticulous attention to detail by the surgical staff. Neurosurgical procedures can last several hours, and improper positioning or inadequate padding of bony prominences can result in significant patient injury and complications. For example, peripheral nerve injury such as brachial plexus or ulnar nerve compression can result in permanent damage that causes numbness, tingling, or intractable pain (Gerken, 2013). Insufficient or improperly placed padding may result in a hospital-acquired pressure ulcer (HAPU). HAPUs are considered one of the Centers for Medicare and Medicaid Services (CMS) “never events,” and the organization will not be reimbursed for any care or treatments to heal a HAPU (McKeon & Cardell, 2011). More importantly, the patient would be subjected to additional pain, the possibility of additional surgical procedures to debride or graft a severe HAPU, and a possible increased length of stay. See Chapter 5 on diagnostics.
Clinical Pearl: When educating the patient, family, or significant other, use the term stroke in addition to the term subarachoid hemorrhage to help them understand that aSAH is a type of stroke.
TREATMENT OVERVIEW
SAH patient care is often organized according to the severity of disease as measured on the aforementioned scales. Treatment includes both medical and interventional options of endovascular coiling or direct surgical clipping of the IA. Patients are generally treated with both medical and interventional options. More conservative or less invasive interventions may be sufficient for patients presenting with a grade I or II SAH. The key goals for all SAH patients are prevention of rehemorrhage (rebleeding), supportive care, pain management, and accurate diagnosis and treatment.
Although the immediate care is aimed at preventing rehemorrhage and securing the aneurysm, other common problems include hydrocephalus and vasospasm. Hydrocephalus is present in up to 30% of patients with intraventricular hemorrhage. In patients with a significant SAH and a higher (more severe) clinical grade, the incidence of rebleeding is higher and may be evident upon admission to the ED or intensive care unit (ICU). Hydrocephalus often occurs within the first 3 days post hemorrhage. Placement of an intraventricular external drainage device is required with the majority of patients showing signs of clinical improvement (Diringer, 2009). Approximately 60% of patients with aSAH have radiographic vasospasm defined as vasoconstriction and narrowing of the cerebral arteries evident with cerebral angiography with or without clinical signs or symptoms. About 39% of patients will have clinical manifestations of vasospasm.
Osmotic or loop diuretics and antihypertensive medications for blood pressure control may be sufficient to stabilize the patient until surgical or endovascular interventions can be initiated to secure the IA (Becske et al., 2013). Grades III, IV, or V require more aggressive and extensive care that may include intubation and mechanical ventilation to protect the airway and support respiratory effort in patients with decreased level of consciousness. Rapid-sequence intubation (RSI) is recommended for patients with SAH, including sedation and short-acting neuromuscular blockade and other agents that lessen the possibility of an increase in ICP. Intravenous access is necessary, and arterial blood pressure monitoring is preferred; central line access should be accomplished as soon as safely possible. Cardiac monitoring, pulse oximetry, and end-tidal carbon dioxide measurements provide additional data to the health care team to direct care. Other interventions may include an indwelling urinary catheter, seizure prophylaxis, and calcium channel blockers (Becske et al., 2013).
Medical Management
The major concerns after an aSAH are rebleeding, hydrocephalus, and vasospasm. The most immediate concern is rebleeding. The greatest risk of rebleeding and mortality is within the first 8 hours of the initial hemorrhage. About 9% to 17% of patients are at risk of rebleeding in the first 72 hours. Rehemorrhage carries a mortality rate up to 50% (Patel & Samuels, 2012). Nearly 15% of patients rebleed in the first few hours after the initial hemorrhage; however, the risk of rebleeding once the IA is obliterated is about 1% (Washington et al., 2011). Blood pressure control is a major component of prevention of rebleeding and is one of the major categories of drug therapy for management of aSAH. The following section discussed drug therapy used for aSAH, including blood pressure control, vasospasm, and anticoagulants.
Drug Therapy
Historically, large hospitals and academic medical centers have had specialized neuroscience units and staff. In recent years, smaller community hospitals who previously cared for neuroscience patients in general medical or surgical ICUs are progressively becoming more specialized and the number of neuroscience/neurological ICUs and specialty medical-surgical units are increasing. The role for neuroscience-specialized pharmacists is growing, providing an additional level of expertise to understand the effects of neurological illness or injury, drug interactions, and the effects on the patient (Rhoney, 2010). The pharmacist is vital in managing dosing of drugs such as antiepileptics, anticoagulants, antibiotics, pain control, and medications that require pharmacy-dosing services. Patients in the ICU often have multiple continuous intravenous infusions for blood pressure control and sedation and pain medication in addition to scheduled medications for the prevention of seizures, treatment of hyponatremia, or other complications of aSAH. Neuroscience ICUs have evidence-based, drug-specific protocols to reduce medication errors and decrease the variability between providers. Optimizing drug protocols has the potential to increase patient safety and improve outcomes, and collaboration with the pharmacy team is essential (Rhoney, 2010).
Blood Pressure Control
Blood pressure control is critical to reduce the risk of rebleeding until the IA is secured and during the first 24 to 48 hours postoperative period (Becske et al., 2013). The sudden surge in central sympathetic stimulation associated with aSAH results in hypertension, and the generally accepted goal is to maintain the systolic blood pressure less than 160 mm Hg. The risk of rebleeding is highest in the first 24 hours of the initial hemorrhage and carries a mortality rate up to 78%. Opioid pain medications may help to decrease the blood pressure and promote patient comfort; however, administering rapid-acting intravenous (IV) antihypertensive medications given as needed or via continuous infusion work to maintain the blood pressure within ordered parameters (Becske et al., 2013). Nitrates and nitroprusside have fallen out of favor as first-line agents for blood pressure control due to the potential for increased ICP and toxic side effects, particularly with prolonged nitroprusside infusions (Abate & Citerio, 2014).
Labetalol (Normodyne) and hydralazine (Apresoline) are often the favored intermittent dosing medications, whereas nicardipine (Cardene) and clevidipine (Cleviprex) are continuous infusions used for blood pressure control (Diringer, 2009). Labetalol is a beta-blocker that may be administered over 2 minutes in doses ranging from 5 to 20 mg IV every 15 minutes (Labetalol, 2014). Nursing considerations for this medication include a maximum dose of 300 mg in 24 hours and a contraindication for patients with bradycardia. Beta-blocker antihypertensives should be held for heart rate of less than 60 beats per minute. Labetalol is contraindicated in patients with a history of asthma, obstructive airway disease, or cardiac conditions that are associated with bradycardia or heart block (Physician’s Desk Reference [PDR], 2014). Hydralazine may be given in incremental IV doses of 20 to 40 mg every 30 to 60 minutes. The mechanism of action of hydralazine is also vasodilation, but with a direct effect on arterioles to decrease systemic resistance and reduce blood pressure. The duration of action is longer than labetalol and lasts 1 to 4 hours with a half-life of 2 to 8 hours. Contraindications include patients with coronary artery disease (CAD); it should be used with caution in patients with severe kidney impairment, hypovolemia, or stroke. The precaution for stroke patients is to avoid a sudden drop in blood pressure related to antihypertensive medications, particularly in ischemic stroke that may result in a hypoperfusion injury to the brain (PDR, 2014).
Nicardipine (Cardene) is given via continuous IV infusion in titrated doses from 5 to 15 mg/hr to maintain systolic blood pressure of maximum 150 to 160 mm Hg to prevent rebleeding. Some clinicians prefer to maintain the blood pressure less than 140 mm Hg to prevent rehemorrhage (Green et al., 2013). Nicardipine is a calcium channel blocker that inhibits transmembrane influx of extracellular calcium ions across vascular smooth muscle and cardiac cells without effect on serum calcium levels. Side effects may include symptomatic hypotension or tachycardia and should be used with caution in patients with congestive heart failure (CHF) or left ventricular dysfunction. Nicardipine has a rapid-acting onset of action and has been shown to be effective to reduce blood pressure in a controlled manner. The half-life of this medication is about 2 to 4 hours. It is recommended to change the peripheral infusing sites every 12 hours (PDR, 2014).
Clevidipine (Cleviprex) is also a calcium channel blocker with a similar mechanism of action as nicardipine. The onset of action is about 2 to 4 minutes, with a half-life of about 15 minutes. Dosing and titration begin at 1 to 2 mg/hr and may be doubled every 90 seconds. When the blood pressure is near the targeted goal, dosing is less than doubled and titration times are increased from the rapid titration interval to every 5 to 10 minutes. Clevidipine is in a lipid base without preservatives. Patients should be screened for allergy or hypersensitivity to soy or egg products prior to administration. This medication is contraindicated in patients with CHF (PDR, 2014).
Vasospasm Prevention and Treatment
The drug categories associated with the prevention and treatment of vasospasm include calcium channel blockers, magnesium, endothelin antagonists, and statins. These categories are reviewed in the following sections to describe their current use, if any, in vasospasm management.
Calcium Channel Blockers
Calcium channel blockers (CCBs) have been used for their effect to reduce contraction of cardiac and smooth muscle without an effect on skeletal muscle. The effectiveness of this classification of drugs has been a source of many studies over the years, but it is theorized that the abnormal vasoconstriction of cerebral vascular smooth muscle is mitigated by CCBs (Greenburg, 2010). Nimodipine (Nimotop) is a cost-effective agent as well as the drug of choice for use in patients with aSAH. A side effect of nimodipine is hypotension, especially if the patient is not well hydrated. Titration of vasoactive infusions may be necessary to augment blood pressure post dosing. Patients may be sensitive to blood pressure fluctuations. As a result, focal deficits and a decrease in blood pressure can occur after CCBs are administered to patients with vasospasm. The neuroscience nurse must be aware of this side effect and closely monitor the patient’s vital signs and neurological assessment when this medication is given. Nimodipine is generally administered orally (PO) and recently became available in IV form. However, oral administration is generally preferred. Nimodipine is given in doses of 60 mg every 4 hours or 30 mg every 2 hours for 21 days. If hypotension is a recurring problem after dose is given, the recommendation is to administer smaller, more frequent dosing (Diringer, 2009).
Clinical Pearl: Sudden and severe decreases in blood pressure with associated focal neurological deficits have been reported anecdotally by neuroscience nurses when nimodipine has been given via a duodenal feeding tube. Start nimodipine at the lowest ordered dose to assess the patient’s response to the medication.
Magnesium
Magnesium has been studied and found to have mixed results on neuroprotective and vasodilatation properties. Vasodilation results from an inhibition of calcium channel–mediated smooth muscle contractions. Various spasmogenic agents such as endothelin-1, norepinephrine, angiotensin II, and serotonin are counteracted by magnesium. Magnesium is thought to have some degree of neuroprotective effects because hypomagnesemia is associated with a worse outcome (PDR, 2014). Reportedly, more than one third of patients admitted with aSAH have hypomagnesemia that is related to the size of the hemorrhage. Studies with magnesium administration protocols in SAH have not determined dosing or optimal magnesium levels (Patel & Samuels, 2012). One consideration when infusing magnesium is the effect on the heart and arrhythmias, specifically the risk for torsades de pointes. Protocols for magnesium infusions for SAH generally include cardiac considerations and parameters for QT and QTc. The QT interval is the time between the start of the Q wave and the end of the T wave in the cardiac electrical cycle. This measurement provides information on how quickly the ventricles are repolarized and ready for a new cardiac cycle. The QTc is the measurement of time between the onset of ventricular depolarization and the completion of ventricular repolarization. Magnesium infusion should be limited in patients with a QTc less than 440 or 445 to avoid prolongation of the QT interval and the potential for lethal dysrhythmia (Frangiskakis et al., 2009).
The Magnesium for Aneurysmal Subarachnoid Hemorrhage (MASH-2) trial was a phase 3, randomized, placebo-controlled trial conducted in eight centers in Europe and South America. A total of 1,204 patients were enrolled. An updated Cochrane meta-analysis of seven prior randomized trials from MASH-2 and those randomized trials that were eligible for inclusion since the MASH-2 trial involved 2,047 patients and demonstrated that magnesium was not superior to a placebo in reducing poor outcomes after aSAH. Therefore, magnesium could not be recommended for routine administration in aSAH (Dorhout et al., 2012). Another prospective, randomized, placebo-controlled study found that the incidence of delayed ischemic neurological deficit was significantly lower in patients treated with magnesium infusion. Transcranial Doppler (TCD) and angiographically identified vasospasm was significantly reduced in the group receiving magnesium. However, it is of note that the outcome scores were measured by Glasgow Outcome Scale (GOS), and patients considered a “good outcome” was scored as a 4 or 5 on a scale of 1 to 5 after 6 months (Westermaier et al., 2010).
Endothelin Antagonists
Endothelin antagonists (ET-A) are another potential treatment under investigation. ET-A receptors mediate vasoconstriction in arterial smooth muscle. Clazosentan is a selective ET-A receptor antagonist that demonstrated a decrease and a reversal in vasospasm after SAH. One study, CONSCIOUS-1 (Clazosentan to Overcome Neurological Ischemia and Infarct Occurring after Subarachnoid Hemorrhage), found a significant dose-dependent effect on vasospasm when evaluated with angiography. The 413 patients in this study were randomized and given placebo or clazosentan within 56 hours and continued on their assigned protocol for up to 14 days (Rhoney, McAllen, & Liu-DeRyke, 2010). Subsequent studies with clazosentan have reported no benefit with this drug.
Statins
Research with statin drugs have also demonstrated a lack of efficacy and have shown no benefit in the treatment of vasospasm; however, studies are ongoing. To date, the recommendations have been to continue the statin medication if the patient had been taking it prior to the onset of the SAH (Abate & Citerio, 2014).
Anticoagulants
Patients presenting with SAH and who were on anticoagulant therapy for cardiovascular conditions have a worse prognosis and outcomes. Anticoagulants tend to increase the amount of bleeding at the time of rupture, thereby increasing the overall amount of blood in the subarachnoid space, basal cisterns, and the parenchyma (Rinkel, Prins, & Algra, 1997). On the other hand, thrombotic complications related to venous thromboembolism (VTE) may be equally as lethal in patients with stroke. Most of the literature regarding stroke and VTE does not differentiate between hemorrhagic and ischemic strokes. Members of the interprofessional team are charged with implementing preventive strategies upon admission that include mechanical (i.e., sequential compression devices) and pharmacological interventions (Field & Hill, 2011).
aSAH induces a prothrombotic state, thereby increasing the risk of VTE, a medical condition that includes deep vein thrombosis (DVT) and pulmonary embolism (PE). Over the past 10 years, the CMS in collaboration with The Joint Commission have focused on hospital-acquired conditions (HACs). HACs have since been tied to hospital reimbursements whereby the hospital is responsible for the costs associated with the care and treatment of the patient who develops one or more of the nine designated HACs. HACs may have additional implications for health care organizations by way of legal action, that is, malpractice lawsuits, and results that are publicly reported on websites such as Medicare Hospital Compare Quality of Care (http://www.hospitalcompare.hhs.gov) (Hight, 2010). Prevention of VTE is one of several quality measures set forth by government agencies to improve care and prevent HAC.
Interprofessional team members must understand the risks of prescribing anticoagulants for patients with SAH whether the IA is secured or unsecured. Accepted clinical practice is to initiate pharmacological prophylaxis therapy 24 to 48 hours after surgery with unfractionated or a low-molecular-weight heparin (Bautista, 2012). However, nonpharmacological interventions are recommended and should be implemented upon admission. VTE prevention measures are often supported by nurse-driven protocols to apply intermittent pneumatic compression (IPC) devices upon admission to the nursing unit. VTE prophylaxis may also include graded elastic compression stockings known in the United States as “TED stockings” and antiplatelet therapy. Additional measures may include early mobilization and prevention of dehydration. Stroke units that promote early mobilization have demonstrated lower rates of DVT. Patients with stroke are at high risk for dehydration due to dysphagia and inadequate oral intake or decreased level of consciousness (Field & Hill, 2011).
Pain Control
Pain management is an important aspect of patient care. Given the “worst headache of my life” complaint, the interprofessional health care team needs to provide interventions to make the patient as comfortable as possible. Nonopioid medications such as acetaminophen (650 mg PO or 1,000 mg IV) can be administered every 4 to 6 hours; however, consideration must be given to contraindications, that is, liver disease. Accurate monitoring by the pharmacist and nurse to ensure that the patient does not receive more than the maximum allowable dose of 3 g in a 24-hour time period is essential. Opioid medications such as fentanyl (12.5 to 25 mg IV every hour as needed), Dilaudid (0.2 to 4 mg IV every 3 to 4 hours as needed), and morphine sulfate (2 to 4 mg IV every 1 to 2 hours as needed) may provide pain relief as well as a degree of sedation and serve to decrease anxiety. Patient effect must be monitored closely to avoid oversedation and an inability to accurately assess the patients’ neurological status. Pain management is essential but with caution so that worsening in neurological status is not masked (Green et al., 2013).
HYDROCEPHALUS
Hydrocephalus is a concern with low-grade aSAH and may impact the ability to effectively control blood pressure. Hydrocephalus is the result of the influx of blood into the ventricles and ventricular system in the brain that disrupts the absorption of CSF by the arachnoid villi. Clipping or coiling the IA does not influence development of hydrocephalus. Approximately 30% of patients with SAH have impaired reabsorption of CSF that requires urgent placement of an external ventricular drain (EVD). An EVD provides a means to manage hydrocephalus and monitor ICP. The intraventricular catheter attached to the external drainage system is placed by a physician or, in some institutions, a specially trained advanced practice nurse. The majority of patients demonstrate clinical improvement after EVD (Diringer, 2009). Hydrocephalus may persist past the acute phase and require placement of a permanent shunt. Interventions with fibrinolytics such as intraventricular recombinant tissue plasminogen activator (rtPA) have been used to facilitate faster clearance of bloody CSF (Diringer, 2009).
The interprofessional team, and especially nursing, must keep in mind multiple considerations for patients with EVDs, including accurate monitoring of ICP and cerebral perfusion pressure (CPP), assessment of the ICP waveform, and correlating the neurological examination findings with ICP changes or changes in level of consciousness when the level of the EVD is raised or the drainage system is closed in the presence of hydrocephalus. Prompt notification to the physician by the nurse is critical to prevent further deterioration. The nurse may anticipate orders for a CT scan to assess ventricle size and the reopening or lowering of the level of the drainage system to assist in draining the CSF. Protocols for EVD site care vary widely across the United States; variations include sterile, occlusive dressings to an open-to-air insertion site, with site care at specified intervals. The nurse should follow the institutional protocols for monitoring parameters and care of the drainage system.
Case Study: Mr. W., a 68-year-old patient with a ruptured anterior communicating artery IA, is postbleed day 12 and postendovascular coiling of aneurysm day 11. He is able to follow simple commands with all extremities, but his legs are weaker than his arms. His EVD is open to drain at 10 cm H20 and draining approximately 10 to 15 mL/hr. The CSF color remains bloody but has progressed to a light pink color. The neurosurgeon ordered the EVD level to be raised to 15 cm H20 yesterday; today he orders the level increased to 20 cm H20. After about 6 hours, the nurse notes Mr. W. to be more lethargic, slower to follow commands, and requires frequent stimuli (verbal and tactile) during the assessment. His ICPs had increased from an average of 8 to 10 mm Hg to 15 to 18 mm Hg. The neurosurgeon was notified immediately of the decline in neurological status, and a CT scan was ordered and completed “STAT.” The CT scan demonstrated enlarged ventricles when compared to the last CT scan done 3 days prior. The surgeon ordered the level of the EVD to be decreased back to 10 cm H20. Within another 6 hours, Mr. W. was becoming more responsive and less lethargic, and the ICPs have returned to baseline of 8 to 10 mm Hg. Mr. W. required placement of a ventriculoperitoneal shunt prior to his transfer from the ICU for a permanent, internal means to control hydrocephalus (Fig. 8-3). ■
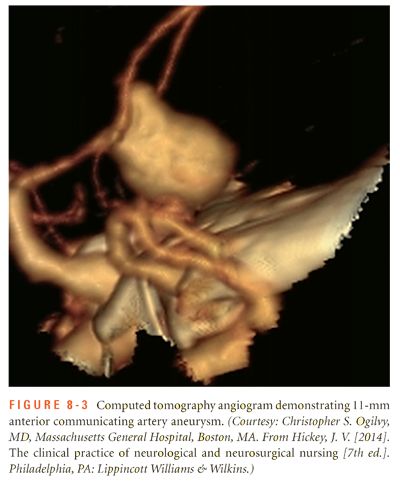
VASOSPASM
As previously mentioned, approximately 60% of patients with aSAH will develop radiographic vasospasm defined as vasoconstriction and narrowing of the cerebral arteries evident with cerebral angiography with or without clinical signs or symptoms. About 30% of aSAH patients will have clinical vasospasm, which is also referred to as delayed ischemic neurological deficit (DIND). DIND or delayed cerebral ischemia (DCI) is gaining favor to describe the effects of vasospasm, which is described as worsening or new focal neurological deficits or obvious sites of ischemic damage on CT scan (Abate & Citerio, 2014). These patients will be symptomatic, with changes that are characterized by a decreased level of consciousness or confusion or focal neurological deficits (Greenburg, 2010). Patients with vasospasm and DCI have longer lengths of stay in the ICU and may have new onset of neurological deficits and an overall poorer prognosis and recovery. Vasospasm is the result of cerebral arterial narrowing that is thought to be triggered by the breakdown of hemoglobin in the subarachnoid space, which creates an inflammatory response, impairs autoregulation, and results in ischemia and infarction (Abate & Citerio, 2014; Bautista, 2012). The CT scan provides a means to measure the size of the subarachnoid blood and visualize blood in the cisterns or the ventricles. The Fisher grading scale and modified Fisher grading scale are helpful in predicting the risk of vasospasm (Table 8-6).
Vasospasm begins at about postbleed day 3 and may last 21 days. The most severe vasospasm is seen between postbleed days 7 and 10. The neurological status of the patient may “wax and wane” throughout the period of vasospasm, testing the assessment skills of the neuroscience nurse and the expertise of the interdisciplinary team. Invasive monitoring devices to measure brain tissue oxygenation, cerebral microdialysis, thermal diffusion flow measurement, and near-infrared spectroscopy may be helpful in the management of patients with vasospasm but are not available in all neurological centers.
Noninvasive monitoring devices such as TCD measure blood flow velocities, thereby approximating the degree of narrowing in the anterior and posterior cerebral circulation. Determination of vasospasm via TCD relies on a ratio of flow velocities between the middle cerebral artery (MCA) and internal carotid artery (ICA). When the flow velocities are greater than 200 cm/sec and the MCA-to-ICA ratio is greater than 6, vasospasm is present. Vasospasm is a severe complication of aSAH that will result in death in about 25% of patients and leave another 50% with severe disabilities (Abate & Citerio, 2014).
In the past, the so-called triple H therapy was the mainstay of treatments for vasospasm. The use of triple H therapy employed a combination of hypertension, hypervolemia, and hemodilution aimed at “thinning” the blood (IV infusions of saline and/or albumin) with a goal of decreasing the hematocrit. The rationale for hemodilution is to decrease blood viscosity, thus increasing cerebral blood flow. However, multiple studies have demonstrated that hemodilution reduces the oxygen-carrying capacity of the red blood cells, resulting in a diminished cerebral oxygen delivery. Several adverse effects have been associated with hypervolemia; it has not shown efficacy over maintaining euvolemia (Abate & Citerio, 2014). As a result of the research, practitioners are changing the treatment to include hypertension but promote an overall state of euvolemia (Diringer, 2009).
As practitioners move to euvolemia, 24 to 48 hours after the aneurysm is secured, permissive hypertension is becoming part of the treatment; that is, the patient’s blood pressure is naturally allowed to trend upward. When signs and symptoms of vasospasm occur, an IV infusion with vasoactive drugs can be initiated to further increase the blood pressure and, consequently, cerebral blood flow and perfusion. Hypertension is accomplished with IV fluids and the addition of vasoactive infusions such as phenylephrine (Neo-Synephrine) or norepinephrine (Levophed) to maintain the systolic blood pressure (SBP) to a level dependent on the patient’s condition and provider practice preferences. Studies have shown that increasing the blood pressure to an individual goal or to a percent of the baseline blood pressure based on the presence or absence of the patient’s clinical symptoms is the most effective means to manage the patient (Diringer, 2009). Additional vasoactive drugs may be needed to maintain SBP and cardiac output (e.g., dopamine, dobutamine, milrinone) depending on the patient’s past medical history and the presence of preexisting or new-onset cardiac dysfunction.
Hypovolemia is a complication of the initial IA rupture. Management of fluids to avoid vasospasm is a balancing act to provide an adequate fluid volume status (i.e., at least maintaining a euvolemic state) and avoid hypervolemia that may induce cerebral edema, pulmonary edema, cardiac arrhythmias, CHF, or sepsis. Research has compared hypervolemia with euvolemia and found no significant differences in the clinical setting. Use of normal saline (0.9%) works well to maintain volume status; however, hypertonic saline (1.5%, 3%, or 7.5% concentrations) may be used for patients with hyponatremia (Green et al., 2013).
Recommendations for care of the patient with vasospasm include intravascular fluid monitoring with the use of invasive and/or noninvasive devices. Examples of invasive devices are central lines or pulmonary artery (PA) catheters. Central venous pressure can be measured with a central line or PA catheter, and caution should be used with central venous pressure values because use of these values has not been shown to be a reliable indicator of volume or of the cardiac response (Bautista, 2012). PA catheters are not recommended due to the high risk of complications with the catheter and the lack of evidence that its use have a positive impact on the patient’s overall care. Noninvasive monitoring devices such as an arterial pressure–based monitor (Flo-Trac, Edwards Lifesciences, Irvine, CA) are available. It should be noted that the limitations with an arterial pressure–based monitoring device require a patient to be intubated and control-ventilated with an arterial line that has an accurate, consistent waveform.
Considerations for critical care nurses are blood pressure monitoring and maintaining blood pressure within the ordered parameters to optimize cerebral perfusion. Hypotension can be treated with bolus infusions of IV fluids and use of vasoactive infusions if hypotension persists. Obviously, hypertension should be avoided to prevent rebleeding if the IA has not been secured, and antihypertensive medications are indicated to maintain SBP less than 160 mm Hg.

Full access? Get Clinical Tree
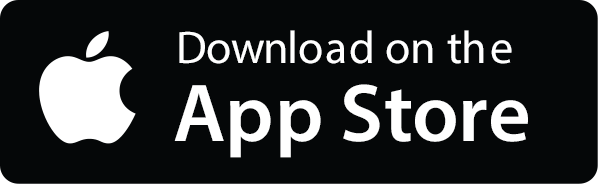
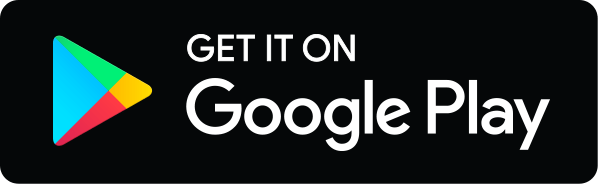