The editors and publisher would like to thank Drs. Kenneth Drasner and Merlin D. Larson for contributing to this chapter in the previous edition of this work. It has served as the foundation for the current chapter.
Principles
Spinal, epidural, and caudal blocks are collectively referred to as central neuraxial blocks . Significant technical, physiologic, and pharmacologic differences exist between the techniques, although all result in one or a combination of sympathetic, sensory, and motor blockade. Spinal anesthesia requires a small amount of drug to produce rapid, profound, reproducible, but finite sensory analgesia. In contrast, epidural anesthesia progresses more slowly, is commonly prolonged using a catheter, and requires a large amount of local anesthetic, which may be associated with systemic side effects and complications unknown to spinal anesthesia. Combined spinal and epidural techniques blur some of these differences but add flexibility to clinical care.
Practice
Neuraxial blockade is widely utilized in surgery, obstetrics, acute postoperative pain management, and chronic pain relief. Single-injection spinal or epidural anesthesia is commonly used for surgery to the lower abdomen, pelvic organs (e.g., prostate), and lower limbs and for cesarean deliveries. Continuous catheter-based epidural infusions are used for obstetric labor analgesia and to provide postoperative pain relief for days after major surgery (e.g., thoracic, abdominal, lower limb). Neuraxial analgesia can reduce pulmonary and possibly cardiac morbidity, although the mortality benefits appear minimal. More recently, the goals of epidural analgesia have shifted to facilitation of fast-track surgery recovery. Caudal blocks are mostly performed for surgical anesthesia and analgesia in children (also see Chapter 34 ) and for therapeutic analgesia in adults with chronic pain (also see Chapter 44 ). Indwelling long-term spinal catheters may be inserted for chronic malignant and nonmalignant pain.
Anatomy
The spinal cord is continuous with the medulla oblongata proximally and terminates distally in the conus medullaris as the filum terminale (fibrous extension) and the cauda equina (neural extension) ( Fig. 17.1 ). This distal termination varies from L3 in infants to the lower border of L1 in adults.

The spinal cord lies within the bony vertebral column, surrounded by three membranes: from innermost to outermost the pia mater, the arachnoid mater, and the dura mater ( Fig. 17.2 ). Cerebrospinal fluid (CSF) resides in the subarachnoid (or intrathecal) space between the pia mater and the arachnoid mater. The pia mater is a highly vascular membrane that closely invests the spinal cord and brain. The arachnoid mater is a delicate, nonvascular membrane that functions as the principal barrier to drugs crossing into (and out of) the CSF. The dura is a tough fibroelastic membrane.

Surrounding the dura is the epidural space, extending from the foramen magnum to the sacral hiatus. The epidural space is bounded anteriorly by the posterior longitudinal ligament, laterally by the pedicles and intervertebral foramina, and posteriorly by the ligamentum flavum. Contents include nerve roots, fat, areolar tissue, lymphatics, and blood vessels.
The ligamentum flavum (the so-called “yellow ligament”) also extends from the foramen magnum to the sacral hiatus. Although classically portrayed as a single ligament, it is actually composed of the right and left ligamenta flava, which join to form an acute midline angle with a ventral opening (see Fig. 17.2 ). The ligamentum flavum thickness, distance to the dura, and skin-to-dura distance vary with the area of the vertebral canal. The vertebral canal is triangular and largest in area at the lumbar levels, and it is circular and smallest in area at the thoracic levels. Immediately posterior to the ligamentum flavum are either the lamina of vertebral bodies or the interspinous ligaments (that connect the spinous processes). Finally there is the supraspinous ligament, which extends from the external occipital protuberance to the coccyx and attaches to the vertebral spines (see Fig. 17.2 ).
There are 7 cervical, 12 thoracic, and 5 lumbar vertebrae and a sacrum ( Fig. 17.3 ). The vertebral arch, spinous process, pedicles, and laminae form the posterior elements of the vertebra, and the vertebral body forms the anterior element ( Fig. 17.4 ). The vertebrae are joined together anteriorly by the fibrocartilaginous joints with central disks containing the nucleus pulposus, and posteriorly by the zygapophyseal (facet) joints. Thoracic spinous processes are angulated more steeply caudad as opposed to the almost horizontal angulation of the lumbar spinous processes. The differences between the caudal and lumbar spinous processes are clinically important for needle insertion and advancement ( Fig. 17.5 ).



The sacral canal contains the terminal portion of the dural sac, which typically ends at S2 in adults and lower in children. The sacral canal also contains a venous plexus.
Spinal Nerves
Dorsal (afferent) and ventral (efferent) nerve roots merge distal to the dorsal root ganglion to form spinal nerves ( Fig. 17.6 ). There are 31 pairs of spinal nerves (8 cervical, 12 thoracic, 5 lumbar, 5 sacral, and 1 coccygeal). The nerves pass through the intervertebral foramen, becoming ensheathed by the dura, arachnoid, and pia, which, respectively, become the epineurium, the perineurium, and the endoneurium. Preganglionic sympathetic fibers originate in the intermediolateral gray columns between T1 and L2 and pass via the ventral nerve root to the paravertebral sympathetic ganglia and more distant plexuses ( Fig. 17.7 ).


Blood Supply
Two posterior spinal arteries supply the posterior one third of the spinal cord, whereas the anterior two thirds of the spinal cord are supplied by a single anterior spinal artery ( Fig. 17.8 ). One of the largest anastomotic feeder arteries to the anterior system is the artery of Adamkiewicz, which arises from the aorta and enters an intervertebral foramen between T7 and L4 on the left. Ischemia within the anterior system leads to anterior spinal artery syndrome, manifested as anterior horn motor neuron injury along with disruption of pain and temperature sensation below the level affected. Ischemia may result from any one or a combination of profound hypotension, mechanical obstruction, vasculopathy, or hemorrhage.

Longitudinal anterior and posterior spinal veins communicate with segmental anterior and posterior radicular veins before draining into the internal vertebral venous plexus in the medial and lateral components of the epidural space. These drain into the azygous system.
Anatomic Variations
Variations exist in size and structure of the spinal nerve roots as well as CSF volume, both of which may contribute to variability in spinal block quality, height, and regression time. Similarly, the epidural space is more segmented and less uniform than previously believed, which may be a factor in the unpredictability of drug spread. Finally, contents of the epidural space also vary and can influence the volume of local anesthetic required.
Mechanism of Action
Local anesthetics disrupt nerve transmission within the spinal cord, the spinal nerve roots, and the dorsal root ganglia. Nerves in the subarachnoid space are easily anesthetized, even with a small dose of local anesthetic, compared with the extradural nerves, which are often ensheathed by dura mater (the “dural sleeve”). The speed of neural blockade depends on the size, surface area, and degree of myelination of the nerve fibers exposed to the local anesthetic. The small preganglionic sympathetic fibers (B fibers, 1 to 3 μm, minimally myelinated) are most sensitive to local anesthetic blockade. The C fibers (0.3 to 1 μm, unmyelinated), which conduct cold temperature sensation, are blocked more readily than the A-delta pinprick sensation fibers (1 to 4 μm, myelinated). The A-beta fibers (5 to 12 μm, myelinated), which conduct touch sensation, are the last sensory fibers to be affected. The larger A-alpha motor fibers (12 to 20 μm, myelinated) are the most resistant to local anesthetic blockade. Regression of blockade (“recovery”) follows in the reverse order. Maximum block height varies according to each sensory modality, termed differential sensory block . Therefore, cold sensation (also an approximate level of sympathetic blockade) is most cephalad and is on average one to two spinal segments higher than the level of pinprick anesthesia, which in turn is one to two segments higher than anesthesia to touch.
Drug Uptake and Distribution
Local anesthetic injected directly into the CSF diffuses from areas of high concentration toward other segments of the spinal cord. Rostral spread, often evident within 10 to 20 minutes, is related to the CSF circulation time. Local anesthetic also diffuses through the pia mater and penetrates through the spaces of Virchow-Robin (extensions of the subarachnoid space accompanying the blood vessels that invaginate the spinal cord from the pia mater) to reach the deeper dorsal root ganglia. A portion of the subarachnoid drug diffuses outward to enter the epidural space, and some is taken up by the blood vessels of the pia and dura maters.
Drug penetration and uptake are directly proportionate to the drug mass, CSF drug concentration, contact surface area, lipid content (high in spinal cord and myelinated nerves), and local tissue vascular supply, but inversely related to nerve root size.
Epidural drug uptake and distribution are more complex. Some of the injected local anesthetic (<20%) moves from the epidural space into the CSF to exert its neural blocking effect, whereas some is lost through either vascular absorption, uptake into epidural fat, or exit via the intervertebral foramina. Other local anesthetic spreads by bulk flow longitudinally and circumferentially within the epidural space. Factors that may enhance the distribution of local anesthetic within the epidural space are small caliber (greater spread in the thoracic space), decreased epidural space compliance, decreased epidural fat content, decreased local anesthetic leakage through the intervertebral foramina (e.g., in the elderly and those with spinal stenosis), and increased epidural pressure (e.g., during pregnancy). The direction of drug spread also varies with the vertebral level. Spread is mostly cephalad in the lumbar and low thoracic region, but caudad after a high thoracic injection.
Drug Elimination
No drug metabolism takes place in the CSF. Regression of neural blockade results from a decline in the CSF drug concentration caused by non-neural tissue uptake and, most importantly, vascular absorption. Increased spread exposes the drug to a larger area for vascular absorption and thus a shorter duration of action. Lipid-soluble local anesthetics (e.g., bupivacaine) bind to epidural fat to form a depot that can slow vascular absorption.
Physiologic Effects
Neuraxial anesthesia evokes blockade of the sympathetic and somatic (sensory and motor) nervous systems. The physiologic effects of epidural anesthesia are similar to those of spinal anesthesia, with the exception that local anesthetic blood levels reach concentrations sufficient enough to produce systemic effects on their own.
Cardiovascular
Blockade of the peripheral (T1-L2) and cardiac (T1-T4) sympathetic fibers as well as adrenal medullary catecholamine secretion reduces systemic vascular resistance (SVR) and, to a much lesser extent, cardiac output. The degree to which arterial blood pressure decreases with either spinal or epidural technique depends on multiple factors.
Systemic Vascular Resistance
The vasodilatory changes depend on both baseline sympathetic tone (i.e., higher sympathetic tone in the elderly equates to a greater hemodynamic change) and the extent of the sympathectomy (i.e., the height of the block). The sympathectomy typically extends for two to six dermatomes above the sensory block level with spinal anesthesia but the same level with epidural anesthesia. If normal cardiac output is maintained, SVR should decrease only 15% to 18% after neuraxial blockade in healthy normovolemic patients, even with near total sympathectomy.
Cardiac Output
Cardiac output is the product of heart rate and stroke volume and it is generally either maintained or slightly decreased during the onset of spinal anesthesia. Venous and arterial vasodilation reduces preload (venous return) and afterload (SVR), respectively. Because 75% of the total blood volume resides in the venous system, the venodilation effect predominates and stroke volume is reduced. Despite a compensatory baroreceptor-mediated sympathetic response (vasoconstriction and increased heart rate) above the level of blockade, the reduction in venous return and right atrial filling reduce signal output from intrinsic atrial and great vein chronotropic stretch receptors, thereby increasing parasympathetic activity. The two opposing responses result in a minimal change in heart rate unless neuraxial anesthesia is extended to the T1 level when blockade of the cardioaccelerator fibers (in addition to a marked reduction in venous return) may result in severe bradycardia and even asystole. The Bezold-Jarisch reflex can also cause profound bradycardia and circulatory collapse after spinal anesthesia, especially in the presence of hypovolemia, when a small end-systolic left ventricular volume may trigger a mechanoreceptor-mediated bradycardia.
Coronary Blood Flow
A decrease in mean arterial pressure is paralleled by a decrease in coronary blood flow. However, a high thoracic block in patients with ischemic heart disease may be beneficial, with improvement in global and regional myocardial function and reversal of ischemic changes likely owing to reduced myocardial oxygen demand and left ventricular afterload.
Central Nervous System
Spinal anesthesia–induced hypotension may decrease regional cerebral blood flow (CBF) in elderly patients and those with preexisting hypertension. However, in studies that demonstrated a decrease in cerebral perfusion, there was no postoperative change in cognitive function in any of the patients. Nevertheless avoiding hypotension would seem prudent.
Respiratory
Alterations in pulmonary variables during neuraxial block are usually of little clinical importance. A decrease in vital capacity follows a reduction in expiratory reserve volume related to paralysis of the abdominal muscles necessary for forced exhalation rather than a decrease in phrenic or diaphragmatic function. These changes are more marked in obese patients and may affect patients with severe respiratory disease. Respiratory arrest can rarely occur owing to hypoperfusion of the respiratory centers in the brainstem but this almost always disappears once cardiac output and arterial blood pressure are restored.
Gastrointestinal
Neuraxial blockade from T6 to L1 disrupts splanchnic sympathetic innervation to the gastrointestinal tract, resulting in a contracted gut and hyperperistalsis because of unopposed parasympathetic (vagal) activity. Nausea and vomiting may occur in as many as 20% of patients. Atropine is effective in treating nausea associated with extensive (T5) subarachnoid anesthesia.
Thoracic epidural anesthesia (TEA) has a direct arterial blood pressure–dependent effect on intestinal perfusion. Correction of systemic hypotension by vasopressor therapy (e.g., norepinephrine) reverses impaired colonic perfusion. Nevertheless, TEA may improve anastomotic mucosal blood flow in patients undergoing esophagectomy and may even reduce the rate of anastomotic leak after emergency laparotomy, esophageal surgery, and other gastrointestinal interventions.
Renal
Despite a predictable decrease in renal blood flow accompanying neuraxial blockade, this decrease is of little physiologic importance. The belief that neuraxial blocks frequently cause urinary retention is questionable (see “ Complications ” later in the chapter).
Indications
Neuraxial Anesthesia
Single injection spinal anesthesia is useful for procedures of known duration that involve the lower extremities, perineum, pelvic girdle, or lower abdomen. It may also be indicated when patients wish to remain conscious or when some comorbid condition, such as severe respiratory disease or an airway that may be difficult to manage, increases the risks of using general anesthesia. Epidural anesthesia allows for more prolonged surgical anesthesia by catheter-based local anesthetic delivery. Indwelling catheter-based spinal anesthesia is less conventional, but may be useful when insertion of an epidural catheter is challenging or in the setting of severe cardiac disease when the reliability of a single-shot spinal anesthetic must be combined with more hemodynamically stable incremental dosing.
Neuraxial Analgesia
Intrathecal or epidural local anesthetics along with other additives, such as opioids either alone or in combination, can provide excellent quality, long-lasting intra- and postoperative analgesia in labor and delivery (also see Chapter 33 ), during and after hip or knee replacement (also see Chapter 32 ), in laparotomy, in thoracotomy (also see Chapter 37 ), and increasingly even in cardiac surgery (also see Chapter 25 ). They may also be used in the management of chronic pain (also see Chapter 44 ).
Contraindications
Absolute
The most important problems include patient refusal, localized sepsis, and an allergy to any of the drugs to be administered. A patient’s inability to maintain stillness during needle puncture (which could expose the neural structures to traumatic injury), as well as increased intracranial pressure (which may theoretically predispose to brainstem herniation) may be absolute contraindications to a neuraxial technique.
Relative
Relative contraindications can be approached by system and must be weighed against the potential benefits of neuraxial blockade.
Neurologic
Myelopathy or Peripheral Neuropathy
Although preexisting central or peripheral neurologic deficit has never been definitively demonstrated to increase susceptibility of injury following neuraxial anesthesia or analgesia (the double-crush phenomenon), the risk-benefit ratio of performing neuraxial techniques should be considered especially in patients with preexisting central or peripheral neurologic diseases such as multiple sclerosis (MS) or diabetic polyneuropathy. Chronic low back pain without neurologic deficit is not a contraindication to neuraxial blockade.
Spinal Stenosis
There is an association between the presence of spinal stenosis and nerve injury following neuraxial techniques, but the relative contribution of surgical factors and natural history of the spinal disease itself is unknown.
Spine Surgery
Previous spine surgery does not predispose patients to an increased risk of neurologic complications. However, in the presence of scar tissue, adhesions, hardware, or bone grafts, needle access to the CSF or epidural space and epidural catheter insertion may be challenging or impossible. In addition, the resultant spread of local anesthetic in the CSF or epidural space in particular can be unpredictable and incomplete.
Multiple Sclerosis
Patients with MS may be more sensitive to neuraxial local anesthetics and exhibit a prolonged motor and sensory blockade. Any association between neuraxial anesthesia and exacerbation of MS symptoms is not based in evidence.
Spina Bifida
Depending on the severity of the neural tube defect the potential for traumatic needle injury to the spinal cord may be increased. The spread of local anesthetic in the CSF and epidural space (if present) can be markedly variable. In any of these circumstances, a careful evaluation of neurologic status must first be undertaken and noted along with documentation of the discussion of the risks and benefits.
Cardiac (Also See Chapter 25 )
Aortic Stenosis or Fixed Cardiac Output
The potential rapid and significant reduction in SVR after spinal anesthesia is in theory a risk in preload-dependent patients and may dangerously decrease coronary perfusion. In the presence of aortic stenosis, neuraxial anesthesia must be considered on an individual patient basis in the context of disease severity, left ventricular function, and case urgency. A catheter-based neuraxial anesthetic with repeated small doses of local anesthetic may allow better hemodynamic control.
Hypovolemia
An exaggerated hypotensive response because of vasodilatory effects may occur.
Hematologic
Thromboprophylaxis and Anticoagulants
Catastrophic cases of spinal hematoma causing paralysis associated with low-molecular-weight heparin (LMWH) have occurred. A summary of the American Society of Regional Anesthesia and Pain Medicine (ASRA) and other professional society guidelines regarding neuraxial techniques (including catheter removal) in patients receiving antithrombotic or thrombolytic therapy is reproduced in Table 17.1 .
Resource | Antiplatelet Medications | Subcutaneous UFH | Intravenous UFH | LMWH |
---|---|---|---|---|
German Society for Anaesthesiology and Intensive-Care Medicine b | NSAIDs: no contraindication; hold LMWH, fondaparinux 36-42 h Thienopyridines and GPIIb/IIIa are contraindicated | Needle placement 4 h after heparin; heparin 1 h after needle placement or catheter removal | Needle placement and/or catheter removal 4 h after discontinuing heparin, heparinize 1 h after neuraxial technique; delay bypass surgery 12 h if traumatic | Neuraxial technique 10-12 h after LMWH; next dose 4 h after needle or catheter placement Delay block for 24 h after therapeutic dose |
Belgian Association for Regional Anesthesia c | NSAIDs: no contraindication Discontinue ticlopidine 14 d, clopidogrel 7 d, GPIIb/IIIa inhibitors 8-48 h in advance | Not discussed | Heparinize 1 h after neuraxial technique Remove catheter during normal aPTT; reheparinize 1 h later | Neuraxial technique 10-12 h after LMWH; next dose 4 h after needle or catheter placement Delay block for 24 h after therapeutic dose |
American Society of Regional Anesthesia and Pain Medicine | NSAIDs: no contraindication. Discontinue ticlopidine 14 d, clopidogrel 7 d, GPIIb/IIIa inhibitors 8-48 h in advance | No contraindication with twice-daily dosing and total daily dose <10,000 U, consider delay heparin until after block if technical difficulty anticipated. The safety of neuraxial blockade in patients receiving doses greater than 10,000 units of UFH daily, or more than twice daily dosing of UFH has not been established. | Heparinize 1 h after neuraxial technique, remove catheter 2-4 h after last heparin dose; no mandatory delay if traumatic | Twice-daily dosing: LMWH 24 h after surgery, regardless of technique; remove neuraxial catheter 2 h before first LMWH dose Single-daily dosing: according to European statements BUT with no additional hemostasis-altering drugs Therapeutic dose: delay block for 24 h |
American College of Chest Physicians d | NSAIDs: no contraindication Discontinue clopidogrel 7 d before neuraxial block | Needle placement 8-12 h after dose; subsequent dose 2 h after block or catheter withdrawal | Needle placement delayed until anticoagulant effect is minimal | Needle placement 8-12 h after dose; subsequent dose 2 h after block or catheter withdrawal Indwelling catheter safe with twice-daily dosing Therapeutic dose: delay block for 18+ h |
a For patients undergoing deep plexus or peripheral block, follow American Society of Regional Anesthesia (ASRA) recommendations for neuraxial techniques.
b Modified from the German Society of Anesthesiology and Intensive Care Medicine Consensus guidelines.
c Modified from the Belgian Association for Regional Anesthesia. Working party on anticoagulants and central nerve blocks.
Inherited Coagulopathy
Hemorrhagic complications after neuraxial techniques in patients with known hemophilia, von Willebrand disease, or idiopathic thrombocytopenic purpura appear infrequently when factor levels are more than 0.5 IU/mL for factor VIII, von Willebrand factor, and ristocetin cofactor activity, or when the platelet count is more than 50 × 10 9 /L before block performance. The minimum safe factor levels and platelet count for neuraxial blockade remain undefined in both the obstetric and general populations.
Infection
Theoretical concerns exist regarding iatrogenic seeding of the neuraxis in the setting of a systemic infection, particularly if a catheter is left in situ. This, along with profound vasodilation, may be sufficient reason to avoid neuraxial techniques in patients with significant bacteremia or septic shock. Some providers avoid neuraxial techniques in febrile patients yet a lumbar puncture is a critical component of the investigation of fever of unknown origin. Patients with evidence of systemic infection may safely undergo neuraxial anesthesia once antibiotic therapy has demonstrated a response.
Spinal Anesthesia
Factors Affecting Block Height
The dermatomal levels required for various surgical procedures are outlined in Fig. 17.9 . Intra-abdominal structures such as the peritoneum (T4), bladder (T10), and uterus (T10) have a spinal segment innervation that may be much more cephalad than the corresponding skin incision used to operate on these structures. Drug, patient, and procedural factors can all affect the distribution of local anesthetic spread within the intrathecal space, but not all are controllable by the anesthesia provider, leading to significant interpatient variability ( Table 17.2 ). Ultimately dose, baricity, and patient positioning are most important.

Factors | More Important | Less Important | Not Important |
---|---|---|---|
Drug factors | Dose Baricity | Volume Concentration Temperature of injection Viscosity | Additives other than opioids |
Patient factors | CSF volume Advanced age Pregnancy | Weight Height Spinal anatomy Intra-abdominal pressure | Menopause Gender |
Procedure factors | Patient position Epidural injection postspinal | Level of injection (hypobaric more than hyperbaric) Fluid currents Needle orifice direction Needle type |
Drug Factors
Baricity
Baricity is the ratio of the density of a local anesthetic solution to the density of CSF. It is conventionally defined at 37° C because density varies inversely with temperature. Plain bupivacaine 0.5%, for example, may be isobaric at 24° C but is slightly hypobaric at 37° C. The density of CSF is 1.00059 g/L. Local anesthetic solutions that have the same density as CSF are termed isobaric , those that have a higher density than CSF are termed hyperbaric , and those with a lower density are termed hypobaric . Dextrose and sterile water are commonly added to render local anesthetic solutions either hyperbaric or hypobaric respectively. Hyperbaric solutions have a more predictable spread, preferentially moving to the dependent regions of the spinal canal. Hypobaric solutions spread to nondependent regions whereas isobaric solutions tend not to be influenced by gravitational forces. The administration of hyperbaric local anesthetic to patients in the lateral decubitus position will therefore preferentially affect the dependent side. The natural curvatures of the vertebral column influence local anesthetic spread in patients placed in the horizontal supine position immediately after intrathecal administration. Hyperbaric local anesthetics injected at the L3-L4 or L4-L5 interspace will spread from the height of the lumbar lordosis down toward the trough of the thoracic kyphosis, resulting in a higher level of anesthetic effect than isobaric or hypobaric solutions.
Dose, Volume, and Concentration
Dose, volume, and concentration are inextricably linked (volume × concentration = dose), but dose is the most reliable determinant of local anesthetic spread (and thus block height) of isobaric and hypobaric solutions. Hyperbaric local anesthetic injections are primarily influenced by baricity.
The choice of local anesthetic or additive drugs (other than opioids) does not influence spread if all other factors are controlled. Opioids may increase mean spread, possibly as a result of pharmacologic enhancement at the extremes of the spread where the local anesthetic block alone would have been subclinical.
Patient Factors
Many patient factors can influence the number of spinal levels which are anesthetized (blocked) from a spinal anesthetic. These factors include extremes of height (short or tall), weight (thin or obese), age (children or the elderly), and gender. Within the range of “normal-sized” adults, a patient’s height does not affect the spread of spinal anesthesia. However, the vertebral column length, which is related to the local anesthetic spread, should influence the dosage.
Although lumbosacral CSF pressure is fairly constant, the CSF volume varies between patients, which influences peak block height and regression. Although block height varies indirectly with CSF volume, CSF volume itself does not correlate well with simple anthropomorphic measurements other than body weight. The increased abdominal mass in obese patients, and possible increased epidural fat, may in theory decrease CSF volume and therefore increase the spread of local anesthetic and block height.
CSF density varies depending on gender, menopausal status, and pregnancy (also see Chapter 33 ), but the clinical relevance of these factors is probably unimportant.
Advanced age is associated with increased block height (also see Chapter 35 ). In older patients, CSF volume decreases, whereas its specific gravity increases. Further, the nerve roots are likely more sensitive to local anesthetics in the aged population.
In the lateral position, the broader shoulders of males relative to their hips make this position slightly more head-up whereas the reverse is true in females. Despite this, whether males actually have reduced cephalad spread as compared to females in the lateral position is not clear.
Variations of the spine such as scoliosis can make needle insertion more difficult but have little effect on local anesthetic spread if the patient is turned supine. Kyphosis, however, in a supine patient may affect the spread of a hyperbaric solution.
Spread of local anesthetic is enhanced by changes in the lumbar lordosis during pregnancy, as well as by the volume and density of CSF, by twin pregnancies compared with singletons, by intra-abdominal pressure increases (possibly), and by a progesterone-mediated increase in neuronal sensitivity (also see Chapter 33 ).
Procedure Factors
The spread of local anesthetic within the subarachnoid space appears to stop 20 to 25 minutes after injection; thus, positioning of the patient is most important during this period, particularly in the initial few minutes. Although a 10-degree head-up tilt can reduce the spread of hyperbaric solutions without hemodynamic compromise, a head-down tilt does not always increase the spread of hyperbaric bupivacaine. Flexion of the hips in combination with the Trendelenburg position flattens the lumbar lordosis and increases cephalad spread of hyperbaric solutions. A “saddle block” in which only the sacral nerve roots are anesthetized can be achieved with a small dose of hyperbaric local anesthetic while the patient remains sitting for up to 30 minutes. Block height is more extensive with hypobaric solutions if they are administered to patients who are in the sitting position.
The specific needle type and orientation of the orifice may affect block quality. With hypobaric solutions, cephalad alignment of the orifice of Whitacre, but not Sprotte, needles produces greater spread. The orientation of the needle orifice does not appear to affect the spread of hyperbaric solutions. When directing the needle orifice to one side (and using hyperbaric anesthetic), a more marked unilateral block is achieved again when using a Whitacre rather than a Quincke needle.
The level of injection does not affect block height with hyperbaric solutions. With isobaric solutions, the block height is generally higher the more cephalad the injection. Injection rate and barbotage (repeated aspiration and reinjection of CSF) of isobaric and hyperbaric solutions have not consistently been shown to affect block height. The injection of local anesthetic or even saline into the epidural space after a spinal anesthetic increases the block height and is discussed later.
Duration of the Block
Duration is affected primarily by the dose, the intrinsic properties of the local anesthetic (which affect elimination from the subarachnoid space), and the use of additives (if applicable). Hyperbaric solutions have a shorter duration of action than isobaric solutions.
Pharmacology
The clinical effects of intrathecal local anesthetics are mediated by drug uptake and distribution within the CSF, and elimination. These variables in turn are dictated in part by the pKa (dissociation ionization constant), lipid solubility, and protein binding of the local anesthetic solution. Rather than their pharmacologic structure (i.e. amide or ester), local anesthetics are usually classified by their duration of action. The choice and dose of local anesthetic depend on both the expected duration and the nature (location, ambulatory) of surgery. Table 17.3 shows a range of local anesthetics commonly used for spinal anesthesia with corresponding doses, onset times, and durations of action.
Local Anesthetic Mixture | Dose (Mg) | Duration (Min) | Onset (Min) | ||
---|---|---|---|---|---|
To T10 | To T4 | Plain | Epinephrine (0.2 mg) | ||
Lidocaine 5% (with/without dextrose) | 40-75 | 75-100 | 60-150 b | 20-50% | 3-5 |
Mepivacaine 1.5% (no dextrose) | 30-45 c | 60-80 d | 120-180 e | – | 2-4 |
Chloroprocaine 3% (with/without dextrose) | 30-40 | 40-60 | 40-90 f | N/R | 2-4 |
Bupivacaine 0.5-0.75% (no dextrose) | 10-15 | 12-20 | 130-230 g | 20-50% | 4-8 |
Levobupivacaine 0.5% (no dextrose) | 10-15 | 12-20 | 140-230 g | – | 4-8 |
Ropivacaine 0.5-1% (with/without dextrose) | 12-18 | 18-25 | 80-210 h | – | 3-8 |

Full access? Get Clinical Tree
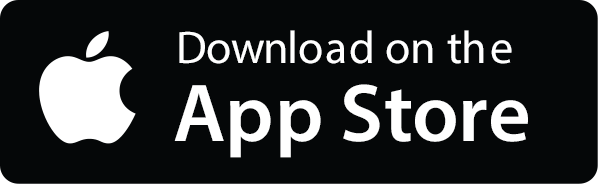
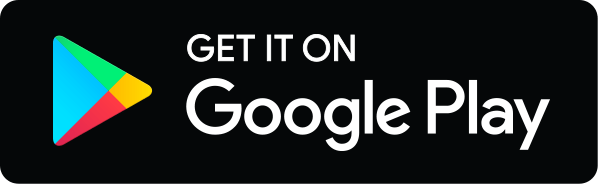
