The chronic pain associated with injury or disease of the central nervous system (CNS) represents a long-standing puzzle, presents a significant challenge to scientific and health care communities, and is consequently a significant barrier to optimal rehabilitation and quality of life in these patients. The mystery of how a lesion in systems that mediate pain perception can actually produce pain continues to baffle those searching for an underlying mechanism. The evidence that we have clearly points to a complicated and heterogeneous pathophysiology and, as in all chronic disease, involvement of psychosocial components. Because therapy for pain from spinal cord injury (SCI) is challenging, it is clear that a more comprehensive understanding of the mechanisms of disease (treatment targets) is crucial. This chapter focuses on clinical identification of the mechanisms and subsets of this condition, its pathobiology, and the current (rather weak) evidence base for treatments.
Prevalence and Incidence
Pain is a frequent consequence of paraplegia and tetraplegia following both complete and incomplete spinal cord lesions. The development of pain after SCI was first described over 100 years ago, and currently more than 600,000 individuals in the United States live with SCI, with approximately 11,000 new such injuries occurring per year. Historically, the loss of sensory, motor, bowel, bladder, and sexual function was viewed as the most significant consequences of SCI. However, the onset and presence of pain directly relate to the ability of patients to regain optimal levels of function and quality of life, and unmanaged pain may be a principal cause of the failure of optimal rehabilitation. An early study reported that 37% of SCI patients with cervical or high thoracic lesions would trade relief of pain for any chance at regaining motor function. In one survey, 11% of respondents reported that pain rather than loss of motor function prevented them from working. In another study, 37% of patients experiencing SCI pain rated the pain as “very difficult to deal with” (rating it from 7 to 10 on a scale from 0 to 10). These and many other studies lend support to the pervasive and negative impact of pain on function and quality of life following SCI.
Historical prevalence analyses of any type of pain after SCI have found it to range from 34% to 96% (approximate mean of 67%) in studies published between 1947 and 1991. More recent studies using more comprehensive pain assessment strategies indicate that the overall prevalence of all types of SCI pain ranges from 70% to 80%.
Classification/Taxonomy
Clinical observations suggest that the variety of pain syndromes associated with SCI together with the lack of a unified taxonomy has been the principal impediment to not only accurate epidemiologic studies but also effective clinical research. The International Spinal Cord Injury Pain classification was proposed after review by major SCI and pain organizations and validation using vignettes. In this system, the type of SCI pain is classified as nociceptive (visceral, musculoskeletal, or other) or neuropathic (resulting from a disease or lesion of the somatosensory nervous system). Neuropathic pain following SCI is further classified as neuropathic pain directly related to the SCI (at-level or below-level pain, where level refers to the neurologic level of injury) or other neuropathic pain (indirectly related or unrelated to the SCI). The SCI Pain Task Force of the International Association for the Study of Pain has proposed a similar taxonomy, which represents a further step toward a mechanism-based classification. This taxonomy is divided into three tiers. In the first tier, pain is divided into nociceptive pain (i.e., pain attributable to non-neurogenic tissue damage) and neuropathic pain (i.e., pain attributable to damage to peripheral or central nervous tissue, or both).
The most straightforward and logical classification scheme for clinical assessment simply categorizes pain below the lesion (true “central pain” phenomena), pain at the level of the lesion (most like radiculopathic/neuropathic), and pain above the lesion (often called “musculoskeletal” in the literature). Another important source or cause of pain in clinical diagnosis is pain evoked in a viscus (“visceral pain”). In one study of the incidence and prevalence of different types of pain in a cohort of 100 patients, musculoskeletal pain (above the lesion, 59%) was the most common type, followed by at-level (41%) and below-level (34%) neuropathic pain. Visceral pain (5%) was the least common. The mean time until onset of any type of SCI pain was 1.6 years. The shortest onset times were recorded for at-level pain (1.2 years) and pain above the level of injury (“musculoskeletal,” 1.3 years). Below-level neuropathic pain (1.8 years) and visceral pain (4.2 years) developed later. The delayed onset of pain can potentially cause some confusion for patients who believe that the onset of sensations corresponds to neurologic recovery and thus may present a minor clinical difficulty for physicians who are aware of the reality that these sensations may represent the development of a pathologic condition that complicates the day-to-day management of patients.
Among all types of SCI pain, below-level pain (central pain) is the most common (23% to 52%) and could be the most difficult to treat. Below-level pain is described as the “most severe or excruciating” of all SCI pain reported. Often accompanying below-level pain is autonomic dysreflexia, which is a potentially life-threatening condition triggered by sensory input below the lesion (spasticity, sympathetic nervous system afferent input). Symptoms associated with autonomic dysreflexia include increased or decreased blood pressure and pulse, headache, and a risk for cerebral hemorrhage, cardiovascular collapse, and seizures.
At-level pain is also common (12% to 42%) and “severe” and can likewise be quite difficult to treat. Pain at the level of the lesion may result not only from damage to peripheral nerves near the site of entry into the cord but also from damage to central neural elements and tracts; thus, the pain mechanisms associated with this type of pain are probably various and complicated.
Above-level pain generators are common (60%) and heterogeneous, such as those of “ergonomic” pathology secondary to overuse of the shoulders and arms causing myofascial pain in the shoulder joints from wheelchair use, or “crutch palsy” as a result of mechanical compression of nerves from orthotics. Above-level pain may also be due to mechanics such as secondary changes following fractures and fixation. Above-level pain is important to identify because it is often the easiest to treat effectively.
Visceral pain is usually manifested as dull or cramping abdominal sensations that may be associated with nausea and autonomic reactions. The pain may be related to bowel, bladder, or kidney dysfunction or distention but may also represent a neuropathic type of pain. It is not known whether there is a central component responsible for visceral pain, but damage to central afferent terminals is known to have peripheral consequences. Because SCI patients may not have the typical signs of abdominal illness, a high index of clinical suspicion should be maintained, which requires careful examination whenever any new pain or changes in existing pain occur. Increases in spasticity, pain at any location, or an autonomic reaction may be the only indication of abdominal organ dysfunction. Autonomic dysfunction may complicate the management of SCI patients with a lesion above the splanchnic outflow (sixth thoracic level). At-level and below-level allodynia, hyperalgesia (evoked pain), and spontaneous pain historically represent the primary targets of both basic and clinical research.
Classification/Taxonomy
Clinical observations suggest that the variety of pain syndromes associated with SCI together with the lack of a unified taxonomy has been the principal impediment to not only accurate epidemiologic studies but also effective clinical research. The International Spinal Cord Injury Pain classification was proposed after review by major SCI and pain organizations and validation using vignettes. In this system, the type of SCI pain is classified as nociceptive (visceral, musculoskeletal, or other) or neuropathic (resulting from a disease or lesion of the somatosensory nervous system). Neuropathic pain following SCI is further classified as neuropathic pain directly related to the SCI (at-level or below-level pain, where level refers to the neurologic level of injury) or other neuropathic pain (indirectly related or unrelated to the SCI). The SCI Pain Task Force of the International Association for the Study of Pain has proposed a similar taxonomy, which represents a further step toward a mechanism-based classification. This taxonomy is divided into three tiers. In the first tier, pain is divided into nociceptive pain (i.e., pain attributable to non-neurogenic tissue damage) and neuropathic pain (i.e., pain attributable to damage to peripheral or central nervous tissue, or both).
The most straightforward and logical classification scheme for clinical assessment simply categorizes pain below the lesion (true “central pain” phenomena), pain at the level of the lesion (most like radiculopathic/neuropathic), and pain above the lesion (often called “musculoskeletal” in the literature). Another important source or cause of pain in clinical diagnosis is pain evoked in a viscus (“visceral pain”). In one study of the incidence and prevalence of different types of pain in a cohort of 100 patients, musculoskeletal pain (above the lesion, 59%) was the most common type, followed by at-level (41%) and below-level (34%) neuropathic pain. Visceral pain (5%) was the least common. The mean time until onset of any type of SCI pain was 1.6 years. The shortest onset times were recorded for at-level pain (1.2 years) and pain above the level of injury (“musculoskeletal,” 1.3 years). Below-level neuropathic pain (1.8 years) and visceral pain (4.2 years) developed later. The delayed onset of pain can potentially cause some confusion for patients who believe that the onset of sensations corresponds to neurologic recovery and thus may present a minor clinical difficulty for physicians who are aware of the reality that these sensations may represent the development of a pathologic condition that complicates the day-to-day management of patients.
Among all types of SCI pain, below-level pain (central pain) is the most common (23% to 52%) and could be the most difficult to treat. Below-level pain is described as the “most severe or excruciating” of all SCI pain reported. Often accompanying below-level pain is autonomic dysreflexia, which is a potentially life-threatening condition triggered by sensory input below the lesion (spasticity, sympathetic nervous system afferent input). Symptoms associated with autonomic dysreflexia include increased or decreased blood pressure and pulse, headache, and a risk for cerebral hemorrhage, cardiovascular collapse, and seizures.
At-level pain is also common (12% to 42%) and “severe” and can likewise be quite difficult to treat. Pain at the level of the lesion may result not only from damage to peripheral nerves near the site of entry into the cord but also from damage to central neural elements and tracts; thus, the pain mechanisms associated with this type of pain are probably various and complicated.
Above-level pain generators are common (60%) and heterogeneous, such as those of “ergonomic” pathology secondary to overuse of the shoulders and arms causing myofascial pain in the shoulder joints from wheelchair use, or “crutch palsy” as a result of mechanical compression of nerves from orthotics. Above-level pain may also be due to mechanics such as secondary changes following fractures and fixation. Above-level pain is important to identify because it is often the easiest to treat effectively.
Visceral pain is usually manifested as dull or cramping abdominal sensations that may be associated with nausea and autonomic reactions. The pain may be related to bowel, bladder, or kidney dysfunction or distention but may also represent a neuropathic type of pain. It is not known whether there is a central component responsible for visceral pain, but damage to central afferent terminals is known to have peripheral consequences. Because SCI patients may not have the typical signs of abdominal illness, a high index of clinical suspicion should be maintained, which requires careful examination whenever any new pain or changes in existing pain occur. Increases in spasticity, pain at any location, or an autonomic reaction may be the only indication of abdominal organ dysfunction. Autonomic dysfunction may complicate the management of SCI patients with a lesion above the splanchnic outflow (sixth thoracic level). At-level and below-level allodynia, hyperalgesia (evoked pain), and spontaneous pain historically represent the primary targets of both basic and clinical research.
Pathology and Mechanisms
SCI pain is related to the nature of the lesion, damage to neurologic structures, secondary pathophysiologic changes in surviving tissue (neural and non-neural tissue), and associated psychosocial syndromes. Because pain is a significant consequence of SCI, it is crucial to understand the mechanisms involved to target therapy more efficiently. Experimental models reveal crucial insights related to the potential mechanisms responsible for pain following SCI. These models and methods try to explain a complicated series of anatomic and functional changes seen in spinal and supraspinal locations. There are many similarities between the cellular and chemical events that occur after SCI and those that follow peripheral nerve injury. Furthermore, neuropathic lesions are easier to study (both with animal models and clinically), and there is considerably more evidence in the treatment of neuropathies. Thus, because of the absence of much useful evidence on the treatment of SCI pain, we must often extrapolate mechanisms and therapy from the more extensive peripheral neuropathy literature.
Traumatic or ischemic damage to the spinal cord triggers a dynamic cascade of molecular, biochemical, anatomic (“plasticity”), and cellular responses. These events in turn produce physiologic changes at both the spinal and supraspinal level that contribute to the onset of dysesthetic sensations, prominently pain. With evolution of the concept of the “central injury cascade” and its role in initiation and maintenance of SCI pain, there has been good progress in determining certain characteristics of each step involved. Injury to the spinal cord ultimately results in abnormal sensory processing in most cases. Contributing to this are changes in the level of neuronal excitability (the “irritable focus”), denervation supersensitivity (and loss of diffuse noxious inhibitory controls), inactivation or activation of cellular signaling pathways, and glial-neuronal interactions. Cord injuries obviously cause structural damage but can also lead to reorganization of the spinal and supraspinal circuits that integrate process and transmit sensory information. There are also changes in the supralesional centers and their chemical mediators that maintain the balance between inhibition and excitation. Primary and secondary events also ultimately result in afferent dysesthesias and efferent modulation, which result in pathologic changes in the initiation, perception, and maintenance of injury-induced pain. There is an expectation that the completeness (as in surviving tracts) or level of injury should correlate with the onset and type of pain. However, despite a hypothetical correlation between incomplete as well as thoracolumbar lesions and a higher incidence of pain, studies have not identified any other consistent predictors. One very significant problem in understanding and studying pain in SCI is the vagaries of the clinical designations of “completeness.” The American Spinal Injury Association (ASIA) system, though superficially useful in physiatric practice, is not helpful in determining the actual physiologic status of cord structures. Thus, clinically “complete” lesions by ASIA criteria are rarely physiologically or anatomically complete in the sense that there are often surviving tracts (afferent and efferent) and frequently intact and disinhibited sympathetic chains. Reliance on the ASIA system for research categorization is a dead end. Research cannot progress, particularly treatment research, without a surviving tract and functional status subset analysis by formal psychophysical and biometric measurements.
Central pain can be characterized as spontaneous or evoked, persistent or intermittent, and localized or diffuse. A significant number of patients report pain sensations such as “burning,” “shooting,” “radiating,” “ice-like,” “squeezing,” and “stabbing.” There may be allodynia, hyperalgesia, paresthesias, and various dysesthesias, sometimes evoked in “numb” areas. The pain can summate with repeated stimuli (wind-up) and linger with prolonged aftersensations. Pain may be evoked or worsen with many peripheral sensory stimuli such as touch, vibration, and cold. It may arise from visceral or somatic structures or be evoked by infections, sudden noises, jarring movements, and distention of visceral structures, as well as by various affective conditions. Evoked pain can be dramatic and typically persists long after the stimulation ceases (aftersensation). All these features are consistent with so-called central sensitization/augmentation of cerebral sensory processing. Spontaneous pain varies greatly in character and is aggravated by stress and emotion, especially anxiety. It can be provoked or exacerbated by auditory, visual, olfactory, and visceral stimuli. Thus, when evaluating pain, it is critical to assess intensity, sensory signs and symptoms, physical and emotional triggers, mood, sociologic situations, and the quality of life of patients.
Multiple hypotheses have been advanced to explain central pain, including the loss of spinal inhibitory mechanisms, the presence of pattern generators (irritable focus) within the injured cord, sensitization of supraspinal relay nuclei, synaptic plasticity (rewiring), activation of spinal and supraspinal microglia and astrocytes, changes in cellular signaling pathways at spinal and supraspinal sites, involvement of irritative or excitatory generators of the spinothalamic and lemniscal pathways, and loss of thermosensory inhibitory control over pain pathways (one type of diffuse noxious inhibitory control). There is also a possible contribution from altered gene expression.
Another challenge in researching abnormal sensations following SCI is the development of experimental models of injury that are consistent with the pathologic pain characteristics seen in humans. A number of models have been developed, including mechanical trauma, isolated lesions, complete transection, chemical lesions, and ischemic injury. The events that follow SCI are dynamic, progress rostrally and caudally from the core of the lesion, and affect spinal, subcortical, and cortical structures. Since there is a broad spectrum of pathophysiologic changes initiated by SCI, it is a challenge to identify which events have a causal relationship with the onset of pain and which are merely secondary consequences of the injury process in the evolution of pain. In human SCI, a variety of critical methods are available for studying and quantifying psychophysical function. Examples of these methods include thermal quantitative sensory testing (QST; small fibers, lateral spinothalamic tracts), vibration QST (large fibers, dorsal columns), and sympathetic skin response.
Evidence of dysesthesia or pain responses at the level of spinal lesions has been provided by animal models of excitotoxicity, ischemia, and contusion injury. The excitotoxic model simulates the elevation in excitatory amino acids that occurs after injury. The most common experimental model is the weight drop or contusion model, and it is believed to be the most clinically relevant but is difficult to use in studies of sensory function because of hind limb paresis or paralysis interfering with behavioral responses. Although the contusion model has many characteristics in common with the human injury, it has been challenged as an inappropriate model for study of the altered sensation following injury. An alternative approach involves selected spinal lesions (such as cord hemisection) alone or combined with other interventions. These various experimental models produce distinct pathologic or behavioral changes (or both) that appear to be relevant to human SCI, create opportunities to study mechanisms, and may prove useful in drug screening.
Critical events in the aftermath of experimental SCI include a transient elevation in excitatory amino acids and the production of a variety of potentially toxic mediators such as endogenous opioids (dynorphin), prostaglandins, cytokines, nitric oxide, and reactive oxygen compounds. Other consequences of SCI include upregulation of mRNA for tumor necrosis factor-α and dynorphin and activation of transcription and nuclear factors. Activation of the NF-κB family of transcription factors is significant since it has a role in inducing more than 150 genes involved in inflammatory, proliferative, membrane excitatory, and cell death responses.
Within the first 15 minutes after SCI, cell membranes start to break down and the phospholipase, arachidonic acid, and eicosanoid cascades are activated. Reactive oxygen species are destructive by-products of these injury cascades. There are significant increases in intracellular calcium. Calcium is directly involved in multiple intracellular signaling pathways (including pain sensitization). One hour after SCI, DNA microarray analysis has identified changes in the mRNA levels of 165 genes involved in regulating transcription factors, inflammatory processes, cell survival, and membrane excitability. Within hours of injury, ion shifts result in cellular swelling and local edema, expansion of the extracellular space, loss of integrity of the blood–spinal cord barrier, and sustained depolarization in damaged cells. This collection of cellular and molecular events can have a profound impact on the functional state of spinal neurons and ultimately promote the onset of chronic pain. Since there are many possible contributing factors and processes, it is not likely that events occur in a uniform, orderly, or sequential manner.
Descending influences may be involved in the at-level pain phenomena, such as pathways emanating from the rostral ventromedial (RVM) medulla. The abnormal firing of sensory neurons reaches RVM neurons and completes a spinobulbospinal feedback loop that increases the hyperexcitability of neurons in the spinal cord. Central sensitization and synaptic plasticity in the CNS are thought to contribute to acute and potentially persistent pain, although most research in this regard has been done on peripheral neuropathic pain. Central sensitization is thought to involve changes in the excitability of spinal neurons and eventually in the development of spinal and potentially supraspinal pain generators and amplifiers.
Despite evidence supporting the hypothesis that cellular or axonal damage predisposes an individual to both at-level and below-level pain, it may be clinically important to distinguish between these regionally distinct categories of pain. It is interesting to point out that SCI pain may be reported in a progressive sequence from at-level to below-level pain, thus implying that the two clinical entities interact. This suggests the possibility that the abnormal neural activity (spinal or supraspinal) associated with at-level pain predisposes an individual to the development of below-level pain (SCI-type central pain). Importantly, the hypothetical influence of abnormal activity in gray matter nuclei may also influence the development of below-level pain after specific white matter damage.
Following complete spinal transection, pain is reported below the injury with common descriptions such as “uncomfortable sensations,” “tightness,” or “burning.” Severe pain may also follow hemisection. One of the most illustrative conditions that give rise to central pain is syringomyelia. More than half of patients with delayed-onset central pain after a spinal injury have syringomyelia, and it appears that the pathophysiologic changes in spinal tissue surrounding the syrinx itself are responsible for the pain. Damage to Lissauer’s tract by root avulsion can also cause very intense and difficult-to-treat pain syndromes.
Pathologic damage to the central cord is particularly prominent following vertebral dislocation, an injury that compresses the spinal cord and destroys gray matter, typically with variable white matter involvement. In contrast, surgical interruption or compression of long spinal pathways usually involves only portions of the gray matter either directly or by disruption of the blood supply to injured segments. The gray matter damage resulting from these types of lesions more often results in pain. However, when central damage to the cord is minimized by making superficial lesions in one anterolateral column, prolonged contralateral hypoalgesia is produced without chronic pain, allodynia, or hyperalgesia.
One of the challenges in studying below-level pain is finding a behavioral measure that effectively engages the neuronal substrates thought to be involved in producing this type of pain. It is generally agreed that below-level pain has spinal as well as supraspinal (including thalamic and cortical) components. Therefore, to effectively evaluate below-level pain, it is necessary to use a behavioral measure that engages both these components. At present, behavioral measures that use operant tasks as the basis for behavioral responses are the only outcome measures that meet these criteria. For this reason, to effectively study below-level pain one must use an operant behavioral task. Unlike reflex responses, these tasks require cortical processing, an important component required for below-level pain. One difficulty in studying pain conditions in SCI is selection of suitable behavioral measures. For example, if the assumption is that to experience below-level pain the cortex must be engaged, the behavioral measures selected to be used in evaluating this type of pain must produce cortical activation. An example paradigm to test this would be through operant testing that requires cortical processing of afferent input, decision making based on environmental cues, and adapting behavior in response to nociceptive stimuli. An example of an error in this regard would be to consider segmental reflex responses below the lesion as relevant in evaluating below-level pain.
Gray matter damage appears to be an important factor in the development of below-level pain. A recent clinical study in patients with and without below-level pain showed that a common pathologic feature in patients with below-level pain was the involvement of lesions that included a central core of spinal gray matter. Although reduced temperature and pain sensations have been used to support the involvement of damaged spinothalamic connections in the development of central pain, recent evidence has shown that neuronal hyperexcitability is also important in the development of below-level pain. Furthermore, loss of spinothalamic function did not appear to predict this type of pain. This work complements previous magnetic resonance imaging findings showing that patients with below-level pain have larger gray matter lesions than do patients without pain. Additional evidence supporting this conclusion comes from studies showing that anterolateral cord lesions result in evoked pain caudal to the spinal injury only when gray matter is involved and the fact that spontaneous pain behavior can be elicited with spinal lesions restricted to the gray matter. Below-level pain may therefore be expressed when portions of supraspinal sensory processing targets are deprived of input from the classic pain pathways and then indirectly activated by other sources of alternative input from a dysfunctional neuronal core (i.e., pain-generating mechanism) rostral to the site of injury.
Although the condition of pain after SCI is believed to originate from abnormal activity in the spinal cord, there is also support for the involvement of supraspinal structures, such as the diencephalon, that receive abnormal input from the injured cord. The contribution of this input, together with the effects of deafferentation (secondary to the death of spinal projection neurons), sprouting of undamaged fibers, and functional unmasking of nonfunctional local circuits, could contribute to the development of focal generators or amplifiers of abnormal discharges at supraspinal sites. Two reports describing elevated blood flow in thalamic nuclei (possibly reflecting changes in metabolic demand and the functional state of thalamic neurons) lend support to thalamic involvement in supraspinal responses to SCI. Complementing these observations are descriptions of changes in the concentration of metabolites (determined with in vivo magnetic resonance spectroscopy) in the ventral posterolateral nucleus of patients with and without SCI pain and reports of dysrhythmic thalamic activity in hyperreflexic rats following SCI. Changes in the excitability of thalamic neurons represent a potential source of abnormal activity, as well as a site for amplification of incoming information from the spinal cord. Thus, below-level neuropathic pain may be expressed when portions of supraspinal targets are deprived of input from the spinothalamic and other spinal pathways and are activated by abnormal (spontaneous or evoked) activity from levels of the spinal cord above the level of injury.
One factor that remains unclear in the mechanism of below-level pain is the precise role of spinal influences (i.e., abnormal activity near the site of the lesion—the penumbral region) in the maintenance of this type of pain. The transient relief of below-level pain in humans following the application of local anesthetic to the proximal stump of a spinal lesion or removal of segments of cord (cordectomy) rostral to the site of injury suggests that the presence of a spinal generator plays a significant role in maintaining chronic below-level pain. Thus, the supraspinal changes secondary to deafferentation and the emergence of a spinal generator of abnormal activity are two significant events that potentially contribute to the development and maintenance of below-level pain.
Although the majority of SCI pain research has focused on spinal mechanisms of enhanced sensitivity, the potential role of descending (supraspinal) modulation (both facilitatory and inhibitory) should not be ignored. Given that neurokinin-1 receptor (NK1R)-expressing lamina I neurons represents the spinal effector of a bulbospinal projection system involving µ receptor–expressing neurons in the RVM medulla, one can speculate that this feedback system contributes to the condition of SCI pain, similar to the mechanism proposed following peripheral nerve and tissue damage. The fact that RVM neurons undergo significant functional changes following spinal injury suggests that this region contributes to a central pain–generating mechanism following spinal injury. Accordingly, experiments targeting lamina I NK1R-expressing neurons with the neurotoxin saporin conjugated to substance P showed that elimination of these neurons significantly reduced the onset and severity of injury-induced at-level spontaneous pain behavior.
A critical factor in the initial onset of at-level behavioral changes in response to mechanical and thermal stimuli is believed to be loss of inhibitory tone within the injured cord. Loss of spinal inhibition enhances the recruitment of surrounding neurons and spread of abnormal at-level sensations, including pain. The second condition involves emergence of a “pattern-generating mechanism” (spinal and supraspinal). This hypothesis led to the conclusion that not all postinjury pain is due to noxious input; some may be due to changes in the firing patterns, including burst activity and long afterdischarges, of neuronal pools adjacent to a site of injury. Results consistent with a “pattern-generating” mechanism include (1) the existence of focal regions of hyperactivity in the spinal cord region surrounding the site of injury (penumbral region) and thalamus of spine-injured patients, (2) the effectiveness of cordectomy and local anesthetics in alleviating pain when delivered to the injured cord, and (3) sensitization and prolonged afterdischarges of spinal sensory neurons following SCI injury.
Patients with central pain can be studied with neurometabolic techniques such as single-photon emission computed tomography (SPECT), positron emission tomography (PET), functional magnetic resonance imaging (fMRI), and magnetic resonance spectroscopy (MRS), which together with pharmacologic dissection can be helpful in classifying patients according to the pathophysiologic mechanism or mechanisms responsible for producing the central pain. In PET studies patients with chronic pain show a decrease in thalamic activity. These findings may be compatible with the decrease in thalamic neuronal activity between bursts observed in patients with central pain secondary to spinal injury. Cesaro and colleagues, in a SPECT study using an amphetamine tracer, found hyperactivity in the thalamus contralateral to the pain. In another SPECT study, Canavero and associates observed hypoactivity in the parietal cortex of a patient with central pain, thus suggesting that under normal conditions the cortex exerts an inhibitory control over thalamic structures. Consistent with this, four patients with central post-stroke pain (two with hyperpathia) exhibited hyperactivity in the thalamus contralateral to the hyperpathic side. Pattany and colleagues used proton magnetic spectroscopy to study alterations in metabolites resulting from injury-induced functional changes in thalamic nuclei following SCI. In patients with pain the concentrations of N -acetylinositol and myoinositol were different from those without pain, thus suggesting anatomic and functional changes in the region of the thalamus. Recently, there has been a focus on cortical and biochemical changes associated with pain following SCI. Using spectroscopy measurements from the brain and processing involving wavelet-based feature extraction, it was possible to identify biochemical changes that distinguish control subjects from subjects with SCI, as well as to subdivide the SCI group into those with and without chronic pain. Thalamic changes appeared to be linked more strongly to SCI, whereas changes in the anterior cingulate cortex and prefrontal cortex appeared to be specifically linked to the presence of pain. Cortical reorganization related to central pain following SCI was observed with fMRI, consistent with the changes in regional brain anatomy and connectivity observed with diffusion tensor imaging.
Studies have also demonstrated increased frontal blood flow, cortical expression of cholecystokinin (CCK) and opioid peptides, functional changes in thalamic neurons, and cortical neuronal death following SCI, all of which could contribute to the central mechanism responsible for the onset of pain. Throughout the midbrain there are changes in the expression of CCK (an endogenous opioid antagonist) and several isoforms of protein kinase C (an enzyme that phosphorylates opioid receptors and makes them unavailable for binding). These studies demonstrate that following SCI, multiple chemical and anatomic changes (plasticity) take place in the endogenous pain control system. These changes affect cortical structures involved in pain processing and affective motivational/reward areas and begin to provide an explanation for the effects on opioid ligands, receptors, and second messengers. Initiation of the process of synaptic plasticity probably involves glial elements, especially activation of microglia. Microglia may be a target for preventing and treating SCI pain.
The role of the sympathetic nervous system in causing or maintaining SCI pain is very poorly understood, but the fact that many types of SCI injury may spare and perhaps disinhibit the paravertebral sympathetic chain, as well as the awareness of sympathetic dysregulation with cervical and high thoracic injuries (“autonomic dysreflexia”), suggests that this is an area that should be studied.
The pervasive psychosocial factors associated with SCI pain are not well studied. In a study of 42 patients with SCI pain, anger, “negative cognitions,” and less acceptance of the condition were found to be related to greater pain severity, as in most chronic pain conditions. The level of the lesion, completeness of the injury, surgical fusion or instrumentation, and (in this study) veteran status were not associated with pain severity. The authors concluded that “overall, psychosocial factors, not physiological factors, were most closely associated with the experience of pain” and that treatment should be directed at the emotional and cognitive sequelae of chronic SCI pain.
Treatment
At present, because a proven long-term effective treatment of central pain is not available, the strategy for treatment is to consider multiple treatment modalities by extrapolating from similar diseases, especially neuropathy, to systematically determine the best approach for an individual patient. There are varieties of treatment modalities. The realistic goal of treatment of SCI pain is to reduce the intensity of the pain to a tolerable level with interventions that have acceptable risk and cost. In brief, tricyclic antidepressants, gabapentin, and pregabalin are considered first-line drugs (see Table 28.1 ). Mixed serotonin-noradrenaline (and dopamine) reuptake inhibitors may be considered when tricyclic antidepressants are not tolerated. Opioids, including tramadol, are considered second-line treatments. In refractory cases, implantation treatment of central pain may be considered, but the risk and cost of these unproven approaches are of concern. Alternative strategies that may complement existing treatments include comprehensive educational, cognitive, and behavioral approaches, as well as the full array of pain rehabilitation techniques.
Mild to moderate pain | Simple analgesics (e.g., NSAIDs or “Weak Opioids”) |
---|---|
Excruciating, intractable pain | Opioids and adjunctive medications, psychotherapy and rehabilitation techniques; later, more experimental interventions |
Regional inflammation or swelling and edema | Steroids, systemic or targeted (acutely), or NSAIDs (chronically); later, immunomodulators |
Depression, anxiety, insomnia | Sedatives, analgesic antidepressants/anxiolytics with analgesic properties (e.g., TCAs), and psychotherapy. Consider SNDRIs |
Significant allodynia or hyperalgesia | Anticonvulsants (e.g., gabapentinoids, Na + channel blockers), TCAs; consider NMDA receptor antagonists. Baclofen? |
Significant osteopenia and regional trophic changes | Calcitonin or bisphosphonates |
Profound vasomotor disturbance associated with pain in the region affected | Calcium channel blockers, sympatholytics, and/or blocks |

Full access? Get Clinical Tree
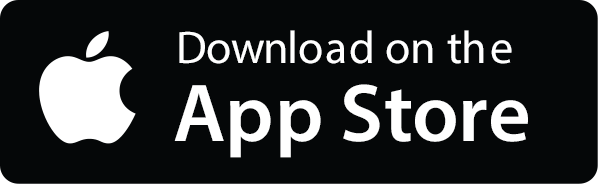
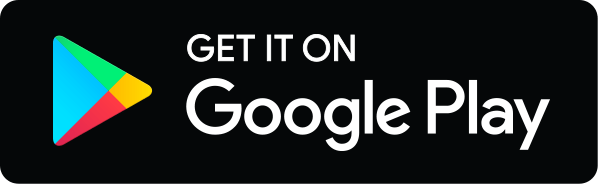
