Fig. 21.1
The vertebral column
Although vertebrae differ in their structure and function depending on their location, most of the articulating vertebrae are comprised of a body, an arch, and seven processes (Fig. 21.2). The vertebral body is the largest and most anterior structure, providing strength to the vertebral column. The intervertebral discs, which function as shock absorbers to the axial skeleton, separate the vertebral bodies. Pedicles arise from the vertebral body and project posterior to join paired, adjoining laminae. Together, these form the vertebral arch that provides the bony protection of the spinal column.


Fig. 21.2
Structure of a vertebra
Seven processes arise from the vertebral arch. At the junction of the right and left laminae, the spinous process projects posteriorly. A spinous process overlaps the process below it with progressively steeper projections from the lumbar to the thoracic regions. This often makes placement of an epidural via the midline approach challenging in the mid- to upper thoracic region. Transverse processes arise from the vertebral arch at the junction of the lamina and pedicle and project posterolaterally. Superior and inferior articular processes project from the junction of the lamina and pedicle. Each articular process has an associated articular facet, enabling extension and flexion of the spine. The spinous and transverse processes allow for the attachment of the deep back muscles, while the articular process restricts movement in particular directions.
The vertebral column has four normal curvatures—cervical, thoracic, lumbar, and sacral. The thoracic and sacral curvatures are concave anteriorly, while the cervical and lumbar are concave posteriorly. This importance becomes apparent when considering the baricity of anesthetic solutions and their distribution in the intrathecal space depending on the position of the patient immediately following intrathecal injection of an anesthetic.
Ligaments
Multiple ligaments link the bony components of the spinal column. They provide a path through which the epidural or intrathecal space may be accessed by a traversing needle. The most posterior of these ligaments and, therefore, the first encountered is the strong supraspinous ligament. The weaker interspinous ligament immediately follows. Together, these ligaments unite adjacent spinous processes in a vertical fashion. Encountered next, the ligamentum flavum (Fig. 21.3) links adjacent lamina and is the final ligament encountered prior to entering the epidural space. It is the strongest and most elastic of the ligaments, often described as having a hard, rubber-like feel as the needle transverses its strong fibers. The posterior longitudinal ligament is anterior to the epidural space and the dural sac, but posterior to the vertebral bodies, so it is not traversed in placement of neuraxial anesthesia. Finally, the anterior longitudinal ligament is anterior to the vertebral bodies.


Fig. 21.3
Spinal ligaments
Spinal Cord
The spinal cord originates from the medulla oblongata in the brainstem and extends to the lumbar region of the spinal canal. It serves as a major neural conduction pathway between the body and the brain, as well as a major reflexive center. In newborns, the cord terminates between the L2 and L3 vertebrae, while in adults it usually extends only to the disc space between L1 and L2. However, as evidenced by MRI scans, the spinal cord extends to L3 in approximately 2 % of adults.
Spinal Nerves
Thirty one (31) pairs of spinal nerves (C1–8, T1–12, L1–5, S1–5, and one coccygeal nerve) emerge from the spinal cord and exit the spinal canal via the intervertebral foramina, except for the coccygeal nerve that exits through the sacral hiatus. Each spinal nerve is comprised of an anterior and posterior nerve root. These are formed by the convergence of anterior and posterior rootlets that arise from the surface of the spinal cord. The part of the spinal cord from which rootlets emerge to form nerve roots comprises a segment of the spinal cord and forms the basis of dermatomal distribution of sensation. Although the spinal cord terminates at L2 in most adults, vertebral discs below this level have corresponding spinal nerves. These nerves emerge as the cauda equina from the inferior aspect of the spinal cord, called the lumbosacral enlargement. The fibers of the cauda equina travel in the lumbar cistern (subarachnoid space), bathed in CSF, until they emerge from the spinal canal at the corresponding vertebral level.
Blood Supply
Not surprisingly, the spinal cord is dependent on a rich blood supply. One anterior and two posterior longitudinal spinal arteries feed the anterior and posterior aspects of the spinal column, respectively. Rather than forming a continuous longitudinal blood supply to the spinal cord, interruption of the anterior spinal artery occurs with segmental blood supply provided by penetrating medullary arteries that arise from the aorta and transit through the intervertebral foramina. In general, three large and discrete areas of distribution along the anterior spinal cord exist, the cervicothoracic area, the mid-thoracic area, and the thoracolumbar area. In addition, these arteries provide blood supply to the posterior and anterior roots of the spinal nerves and their coverings. The largest anterior radicular artery, also known as the artery of Adamkiewicz or anterior radicularis magna, arises from T9 to T12 in 75 % of individuals but may originate as high as T5 or as low as L2. Spinal veins form plexuses that run longitudinally inside and outside the vertebral canal and can often be engorged during pregnancy.
Meninges
The spinal meninges, which consist of the dura mater, arachnoid mater, and pia mater, encase and support the spinal cord and spinal nerve roots. Tough, fibrous tissues comprise the dura mater, the outer most layering of the meninges. The spinal dura arises from and is continuous with the cranial dura mater and extends to the coccyx to form the dural sac. The caudal end of the dural sac is tethered to the coccyx by the filum terminale. The dura extends into the intervertebral foramina enclosing the anterior and posterior nerve roots to form the dural root sleeves.
The arachnoid mater is a lacelike matrix of avascular, fibrous, and elastic tissue that encloses the subarachnoid space. The arachnoid is not attached to the dura but is held against the outer meningeal layer by the pressure of the CSF. Under normal conditions, a spinal needle transverses both the dura and the arachnoid, simultaneously. The subdural space is therefore a potential space in which bleeding may occur (subdural hematoma) or accidental deposition of anesthetic. The pia mater, the innermost meningeal layer, is a thin, delicate yet impermeable layer of fibrous tissue that closely adheres to the surface of the spinal cord. The pia continues to the filum terminale.
The subarachnoid space, filled with cerebral spinal fluid, resides between the arachnoid and the pia maters. Denticulate ligaments, approximately 20 lateral extensions of the pia mater, suspend the spinal cord in the dural sac by adhering to the internal surface of the dura.
Spinal Versus Epidural Blockade
Both spinal and epidural anesthesia occur as a result of inhibition of sensory, motor, and autonomic fibers at the level of the nerve root. However, the two techniques differ in the location of anesthetic deposition and in a number of attributes that may make one technique preferred over the other.
Spinal (also referred to as intrathecal or subarachnoid) anesthesia occurs with the injection of an anesthetic solution into the cerebrospinal fluid. Given the spinal cord does not extend beyond the level of L2 in most adults, injections are limited to interspaces below this level to reduce the risk of spinal cord damage. The technique essentially provides an “anesthetic transection” of the spinal cord with loss of neurologic function below a certain segmental distribution. In contrast, epidural anesthesia occurs with injection of anesthetic into the epidural space, the potential space just outside of the dura through which the spinal nerves traverse. Rather than producing a transection of neural transmission, epidural anesthesia allows for segmental anesthesia with the possibility of continued neurologic function caudal to a band of neural interruption.
Spinal anesthetics are often easier to perform, require less time, and are less dependent on optimal patient positioning during placement. Moreover, aspiration of cerebrospinal fluid at the time of placement provides a quick, real-time assessment of accurate needle position necessary for a successful block. As the anesthetic is delivered within the dural sac, less anesthetic solution is required compared with epidural injection, while producing a more rapid and profound motor and sensory blockade.
Epidural anesthesia has several advantages compared to spinal anesthesia. Epidural anesthesia is more easily titrated, in terms of both the segmental location of the block and the block’s intensity. Epidural anesthesia is often accompanied by less profound hypotension than would be seen with spinal anesthesia. Finally, the routine placement of catheters with epidural techniques allows for easy re-dosing of the block and its transition into the postoperative period as a means of acute, postoperative pain management. Although intrathecal catheter placement is an option, the FDA ordered the withdrawal of all intrathecal microcatheters (27–32 gauge) in 1992. Large bore epidural catheters (19–20 gauge) may be used for intrathecal infusion and newer spinal catheters are now just entering practice.
Physiologic Effects of Neuraxial Blockade
Depending on the technique and agents administered, neuraxial blockade can produce profound systemic homeostatic changes. Both spinal and epidural techniques produce similar physiologic consequences although the incidence and severity vary between the techniques.
Cardiovascular
Hypotension, the most common side effect associated with a subarachnoid block, occurs with an observed incidence of 33 % in a non-obstetric population. Decreased venous and arterial vascular tone leads to the pooling of venous blood, a diminished cardiac output, and decreased systemic vascular resistance. Significant hypotension more often occurs with sensory blocks above T5. This phenomenon likely results from the blockade of sympathetic fibers to the upper extremities that otherwise reflexively constrict to mitigate the vasodilatory effects of the block in the lower extremities. In addition, a sensory block above T1 inhibits the sympathetic cardioaccelerator fibers, thus blunting the reflexive tachycardia that accompanies acute drops in blood pressure.
Hypovolemia exaggerates this response, while other risk factors for significant hypotension during spinal anesthesia include advanced age and the combination of general and spinal anesthesia. Epidural blockade may also induce systemic hypotension with the level blockade likely contributing to the significance of the hemodynamic changes. However, the changes are generally less profound as the onset of sympathetic blockade is more gradual than with spinal anesthesia.
Bradycardia, with an incidence of 13 %, and rarely asystole may occur as a result of spinal anesthesia. Again, the blockade of cardioaccelerator fibers may contribute to this occurrence, although decreased preload seems to be the most significant contributor to bradycardia. Risk factors for bradycardia include a baseline low heart rate, the use of beta-blockers, and ASA physical status I. The latter occurrence is likely due to the rather high vagal tone of young, healthy patients. Other dysrhythmias may occur during spinal anesthesia but with much less frequency.
Respiratory
Neuraxial anesthesia minimally alters respiratory physiology in healthy patients. Given that the phrenic nerve with fibers originating from C3–C5 innervates the diaphragm, high thoracic sensory blocks only minimally affect respiratory mechanics. Tidal volume is largely preserved with small decreases in vital capacity, likely reflecting blockade of accessory muscles of respiration (intercostal and abdominal muscles) and thus decreasing the expiratory reserve volume. Elderly patients undergoing lumbar and thoracic epidural anesthesia experience similarly limited alterations in respiratory mechanics.
Patients with preexisting pulmonary disease and limited respiratory reserve, such as those with severe chronic obstructive pulmonary disease, may be more dependent on accessory muscles to maintain adequate ventilation. Therefore, they may be more susceptible to significant alterations in ventilatory mechanics during neuraxial anesthesia. Mild decreases in forced expiratory volume at one second (FEV1) and vital capacity (VC) are noted in patients with moderate to severe COPD undergoing thoracic epidural or spinal anesthesia. However, the mechanics of quiet breathing appear to be little changed compared to healthy patients and neuraxial anesthesia is often well tolerated.
Gastrointestinal
Neuraxial blockade between T5 and L1 effectively eliminates sympathetic outflow to the abdominal organs producing intestinal hyperperistalsis and thus a small, contracted gut. Nausea and vomiting occur frequently with neuraxial techniques, with an incidence of 18 % and 8 %, respectively, during spinal anesthesia in a non-obstetric population. Etiology of this occurrence likely reflects unopposed parasympathetic activity as atropine appears to be a more effective treatment compared to blood pressure elevation alone. A high sensory blockade, the use of procaine, a history of motion sickness, and hypotension during subarachnoid block appear to be associated with an increased risk for the development of nausea and vomiting.
Renal and Urinary tract
Decreases in blood pressure produce little change in the glomerular filtration rate due to autoregulation of renal blood flow. Delay in micturition and urinary retention are common occurrences during neuraxial blockade for both spinal anesthetics and lumbar epidurals. The potency and dose of anesthetic solution and the addition of opioids, especially long-acting variants, appear to increase the time for return to normal bladder function.
Retention may lead to bladder distension and even rupture in extreme cases. Therefore, careful consideration should be given to intermittent or continuous catheter drainage especially in the setting of intravascular expansion necessary to maintain preload during a neuraxial anesthetic. However, a common misconception is that an epidural catheter requires the retention of a Foley catheter throughout the epidural’s use in the postoperative period. Thoracic epidurals normally have little effect on innervation of the bladder and therefore will not contribute to postoperative urinary retention. A trial to discontinue a Foley catheter early in the postoperative period during an epidural’s continued use should be considered with careful monitoring of the patient’s fluid status.
Spinal Anesthesia
Spinal anesthesia often proves an ideal anesthetic technique for surgeries involving the lower extremities, pelvis, perineum, and lower abdominal area, while a reduced dose of anesthetic produces a block ideal for labor analgesia. Most often, a single injection via a spinal needle delivers anesthetic into the subarachnoid space. Additionally, a catheter may be placed to allow for intermittent or continuous delivery of anesthetic. This technique allows for tighter control in the titration of anesthetic and the ability for re-dosing.
Mechanism
Delivery of local anesthetic into the subarachnoid space induces a rapid and dense blockade of sensory, motor, and autonomic neural transmission. Compared to epidural anesthesia, only small doses of local anesthetic are required to abolish neural transmission due to the lack of dural and arachnoid coverings of nerves within the intrathecal space. Studies revealing the intrathecal distribution of local anesthetic implicate a number of potential sites of action. Not surprisingly, high concentrations of local anesthetics can be found in the posterior nerve roots as they exit the dura. Local anesthetics also diffuse through the pia mater and into the spinal cord, with higher concentrations noted in the posterior and lateral columns, as well as the gray matter of the spinal cord.
Anatomic differences among nerve fibers, including size and myelination, account for their differing sensitivities to blockade by local anesthetics. Blockade of unmyelinated, small diameter sympathetic fibers precedes blockade of the larger, myelinated sensory and motor fibers. The sympathetic block usually exceeds the somatic and motor block by two dermatomal levels, but sometimes by as many as six. This may help to explain the hypotension that accompanies even low sensory blockades. As for the sensory nerve fibers, the C-fibers, which are sensitive to temperature, are blocked first and remain blocked the longest (Fig. 21.4). A-delta fibers, which are responsible for pinprick sensation, are blocked next but are faster to recover than the C-fibers. The fibers that give sensation to touch, the A-beta fibers, are blocked last and recover the fastest. The length of blockade of the A-beta fibers correlates with the length of surgical anesthesia. Finally, the motor fibers are the least sensitive to blockade and typically are blocked two to four levels below the sensory blockade.


Fig. 21.4
Progression of blockade during spinal anesthesia
Besides the dose of local anesthetic injected, several other factors influence the extent of spread of an anesthetic in the subarachnoid space, including the curvature of the spinal canal, the patient’s position for and following injection, and the baricity of the anesthetic solution. An individual’s volume of cerebrospinal fluid as determined by MRI estimation appears to be the most important factor in determining extent of anesthetic dermatomal distribution. This proves to have little clinical utility as a patient’s volume of CSF is neither routinely measured nor easily predicted based on a patient’s characteristics. However, it may help to explain why higher peak sensory levels often occur in patients who are older, obese, or pregnant. In these patients CSF volume is often, although not always, diminished compared to younger, leaner patients.
Intrinsic characteristics of cerebrospinal fluid may play a role in the effects of a subarachnoid block, as the CSF serves as the solvent in which the anesthetic must act. The density of CSF is not constant among patients and varies with characteristics commonly encountered in patients during spinal anesthesia, including age, pregnancy, and illness. Even small changes in the density of CSF affect the baricity of the anesthetic solution—defined as the relative density of the anesthetic solution in relation to its solvent. This may help to explain the observed clinical differences among these patient populations in the extent of anesthetic spread.
Elimination of local anesthetic from the intrathecal space depends on vascular absorption of the anesthetic solution. Intrathecal metabolism does not occur. Blocks covering wider dermatomal areas regress faster than blocks covering fewer dermatomes when the same anesthetic dose is used. The increased surface area allows for faster absorption of the anesthetic by the blood vessels of the pia mater. Toxic blood levels of local anesthetics do not occur because of the relatively small doses required for spinal anesthesia.
Preoperative Evaluation and Consent
As with all anesthetics, a thorough preoperative history and physical exam should identify absolute and relative contraindications for spinal anesthesia (Table 21.1). Particular attention should be focused on a history of cardiovascular, neurologic, and hematologic conditions that may preclude the placement of a neuraxial block. Routine testing of platelet concentration and coagulation studies are not recommended in the absence of clinical suspicion of a bleeding abnormality. The anesthetist should consult with the surgical team regarding the appropriateness of a spinal technique.
Table 21.1
Contraindications for Neuraxial anesthesia
Absolute |
1. Patient refusal |
2. Abnormal coagulation |
3. Thrombocytopenia |
4. Localized infection over needle insertion site |
5. Significant elevation of ICP |
Relative |
1. Severe aortic stenosis |
2. Severe hypovolemia |
3. Idiopathic hypertrophic cardiomyopathy |
4. Mitral stenosis |
5. Bacteremia |
6. Preexisting neurologic disease |
As part of the preoperative visit, a discussion regarding the benefits and potential complications associated with a subarachnoid anesthetic should be undertaken. It may be helpful to stratify risks according to their relative risk of occurrence. That is, it may be more helpful to first describe relatively common occurrences such as treatable hypotension, nausea and vomiting, backache, and post-dural puncture headache. This can be followed by a discussion of the more serious, yet uncommon complications such as nerve damage and infection. Providing rough data on the relative occurrence may help to allay the fears of patients who come to surgery or labor and delivery with misconceptions regarding the risks of spinal anesthesia.
Preparation
As with induction of general anesthesia, monitors are applied prior to the placement of a spinal block. These should include standard ASA monitors, including noninvasive blood pressure cuff and pulse oximetry. When ECG and capnography are not applied they always should be immediately available. With the administration of anxiolytics or opioids, supplemental oxygen is often desirable. Emergency equipment including suction, advanced airway equipment, induction agents, and vasoactive medications should be immediately available. Intravenous access should be established and readily accessible for administration of premedication or emergency vasoactive medications and fluids.
Single-use, disposable spinal trays are commercially available. One should note the agent, concentration, and baricity of the local anesthetic available in the tray, as the formulation may not be appropriate for all situations. Adjunct agents, such as opioids or epinephrine, may be added to local anesthetic solutions as clinically indicated and may require an assistant to pass the drug off in a sterile fashion.
Sterile technique with hand washing, hat, mask, and sterile gloves is universally required. The insertion site should be broadly prepped with antiseptic solution. Currently, most prepared kits are prepackaged with betadine. Care should be taken not to contaminate gloves, work surfaces, or equipment with the solution due to its potential neurotoxicity. Time for drying must be adequate to ensure proper skin sterilization. Chlorhexidine may also be used as a skin prep agent, as it has several advantages over betadine including faster onset of action, extended duration of action, and rare bacterial resistance. Although it lacks FDA approval for use prior to lumbar puncture due to lack of clinical safety regarding its potential for neurotoxicity, a retrospective analysis of more than 12,000 spinal anesthetics did not reveal an increased risk of neurologic complications associated with its use.
Spinal Needles
Spinal needles are classified on how they transverse the dura—those that cut the dura (Quincke) and those that spread the dural fibers (Sprotte or Whitacre). Cutting needles have a bevel tip, while non-cutting needles have a pencil point with the opening on the side of the needle rather than at its tip (Fig. 21.5). Post-dural puncture headache occurs less frequently with smaller-gauged non-cutting needles. All of the needles are designed with stylets to avoid coring out a track of tissue and the potential contamination of the intrathecal space.


Fig. 21.5
Tip designs of common spinal needles. Note: the Whitacre and Sprotte are both pencil-point needles, but the Sprotte needle has a more proximal opening than the Whitacre needle
Technique
Patient Positioning and Anatomic Landmarks
Patient positioning and appreciation of anatomy through palpation of landmarks are essential to the successful, safe placement of a subarachnoid block. Blocks may be performed in the seated, lateral, or prone position. Patient position should be chosen to optimize successful placement, patient comfort and safety, and spread of anesthetic to cover appropriate surgical targets. Consideration may also be given to the eventual surgical position required.
Spinals are generally performed at an interspace level below the conus medullaris to prevent traumatic damage to the spinal cord. Below this level, once the spinal needle enters the subarachnoid space, it is able to push aside fibers of the cauda equina although direct trauma is still possible. The choice of interspace generally does not affect the maximum height of the block, but it may play a role in other characteristics of the block. With hyperbaric bupivacaine, injection of anesthetic at the L2–3 interspace compared to L4–5 produces no higher peak dermatomal levels, but the speed of onset to the peak is faster. However, spread with isobaric bupivacaine is more unpredictable and the choice of interspace likely influences the maximum height of the block.
The midline should be identified with palpation of spinous processes with specific focus at the levels surrounding the site of proposed injection. Drawing a line from the iliac crest to midline may help to identify the L3–L4 intervertebral space, although anatomic landmarks accurately identify the correct interspace only 30 % of the time. A tendency occurs to indentify the L3–4 interspace higher than its actual location. This implies that at least one-third of subarachnoid blocks could unknowingly be placed at the L2–L3 interspace or above. This may place the spinal cord at risk of traumatic damage in a small but significant proportion of patients. Thus, it is not recommended to knowingly attempt a subarachnoid block above the level of L3. Ultrasonography, a quick, noninvasive technique, improves the identification of the correct interspace with a reliability of approximately 70 % and may improve patient safety.
Sitting Position
The seated position facilitates placement of a subarachnoid block by increasing flexion of the spine and thus increasing the size of the lumbar interspinous spaces. Patients should be encouraged to relax their shoulders, slouch forward, and push their lower backs toward the practitioner. This position may also aid in the identification of midline in obese patients. Gravity favors distension of the dural sac, thus making the target for the spinal needle larger, while the increased intra-dural CSF pressure facilitates the identification of free-flowing CSF.
The seated position may not be the most appropriate position for all patients undergoing a spinal block. Patients who require heavy sedation or those with fractures that preclude easy movement to the seated position are poor candidates. Vasovagal syncope may complicate the placement of the block and put the patient at risk for a traumatic fall. In addition, sitting favors the caudal distribution of a hyperbaric anesthetic solution, thus producing a “saddle block” of the perineum if the patient remains in a seated position for a prolonged period. To avoid this, as in the case of a cesarean section, use of an isobaric solution or the timely transition of the patient to the supine position may be necessary.
Lateral Decubitus
The lateral decubitus positioning provides the most patient comfort and is most appropriate for heavily sedated or frail patients. Landmarks are often more difficult to discern, specifically the identification of midline and interspinous spaces. Flexion of the spine by positioning the patient in the fetal position with legs and head tucked toward the body may help to increase the interspinous space and facilitate success.
Prone Position
The prone position, also known as the jackknife position, is primarily used for patients undergoing perineal procedures. This position poses several challenges for the practitioner, including limited flexion of the spine and decreased dural sac pressure sometimes requiring aspiration of CSF to confirm needle placement. In addition, access to the airway is limited should emergent airway management be required. However, once the block is complete little additional maneuvering is required for surgical positioning.
Approach
Prior to insertion of the spinal needle, the skin and soft tissue overlying the intended entry point are anesthetized with a local anesthetic, typically lidocaine. The intrathecal space may be accessed either by a midline or paramedian approach (Fig. 21.6).


Fig. 21.6
Spinal anesthesia: midline and paramedian approach
Midline Approach
The midline approach is generally easier as it passes through less sensitive tissue and requires less angulation of the needle in three dimensions. In this approach, the local anesthetic needle can be used has a “finder” needle, although deep infiltration of local anesthetic is unnecessary and should be avoided especially in thin individuals whose intrathecal space may be as shallow as 3 cm below the surface of the skin. With the paramedian approach, deeper local anesthetic infiltration improves patient comfort. It may be helpful to contact lamina with the local anesthetic needle to anesthetize the periosteum that will be contacted by the spinal needle.
In most patients, the midline approach is the most popular technique for accessing the intrathecal space. With the patient prepped and draped, reestablishment of landmarks is often helpful. Obesity may obscure the identification of midline. In this case, it may be helpful to ask the patient if the intended site feels midline or off to one side. Insertion of the spinal needle through the skin should occur either midway between two adjacent spinous processes or just cephalad to the superior aspect of the inferior spinous process of the interspace being traversed.
Many techniques involve the initial placement of an introducer needle into the spinous ligament through which a smaller-caliber spinal needle is inserted. This helps to stabilize the spinal needle through the skin and soft tissue to prevent deviation from midline that can occur with beveled needles. As the needle is advanced, the practitioner often appreciates a characteristic change in resistance as the spinal needle traverses the ligamentum flavum. This normally is followed by a classic “pop” sensation as the needle pierces the dura and enters the subarachnoid space. Removal of the stylet should allow the free flow of clear CSF. If CSF does not flow freely, reorientation of the needle by 90° increments may improve flow. Aspiration of CSF by a syringe attached to the spinal needle may be required with very small gauge spinal needles or with patients in the prone position.
Paramedian Approach
The paramedian approach is the ideal technique for patients in whom traversing the interspinous space proves difficult, such as those with degenerative disease of the spine or patients in whom ideal positioning may be difficult. Rather than entering midline, entry of the spinal needle occurs 1.5 cm lateral to midline of the spinous process below the intended interspace. Entering skin and contacting the lamina with the spinal needle help to establish landmarks. The needle is then withdrawn slightly and redirected midline by 10–15° and slightly cephalad. If periosteum is contacted, redirection of the needle cephalad often allows the needle to “walk off” the lamina and into ligamentum flavum. Thus, in this technique the supraspinous and interspinous ligaments are bypassed. In cases where the intrathecal space cannot easily be entered, it is best to reestablish landmarks or move to another interspace.
The Taylor technique involves a paramedian approach at the level of L5–S1. Midline approach at this level is often difficult due to the acute downward angulation of the L5 spinous process. The insertion site is 1 cm medial and caudal to the posterior superior iliac spine. The needle is then directed in a medial and cephalad orientation. Again, if periosteum is contacted, the needle is walked off the sacrum into the subarachnoid space.
Anesthetic Administration
Once placement of the needle in the intrathecal space is confirmed, the practitioner’s nondominant thumb and index finger should stabilize the spinal needle against the patient’s back to prevent dislodgement of the needle from the intrathecal space during anesthetic injection. Firm attachment of the anesthetic syringe is key to avoid accidental spillage of anesthetic. Prior to injection, visualization of a “swirl” is confirmation of CSF aspiration. Half of the anesthetic solution is injected followed by a second aspiration to confirm continued placement of the needle within the subarachnoid space. Following injection of anesthetic, the needle and introducer are removed from the patient’s back and the patient is repositioned, if necessary, to the appropriate position for ideal distribution of anesthetic to achieve a specific anesthetic level. Important considerations of anesthetic administration are discussed below.

Full access? Get Clinical Tree
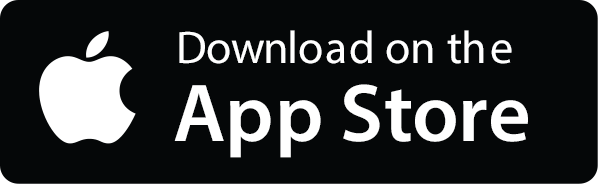
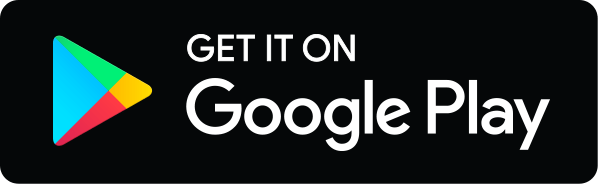