Sleep Issues in the Intensive Care Unit Setting
Kim L. Goring
Nancy A. Collop
Overview of Normal Sleep
Adequate quantity and quality of sleep is necessary for normal physiologic function. The average adult is recommended to obtain 7.5 to 8.5 hours of consolidated sleep within a 24-hour cycle to sustain normal neurohormonal function. The ability to achieve a normal duration and pattern of sleep rests on several factors internal and external to the subject. The important internal factors include the homeostatic and circadian drive to sleep, volitional control of the length of sleep, age, drugs, and comorbid illness. External factors include ambient temperature, noise, and light exposure, all of which influence the quantity and sleep-stage distribution throughout the night [1].
Sleep is divided between non-rapid eye movement (NREM) and rapid eye movement (REM) cycles. The normal human adult begins sleep in NREM with REM sleep occurring approximately 90 to 120 minutes later. NREM sleep is composed of stages 1 (N1), 2 (N2), and 3 (N3). Slow-wave sleep (stage 3), a deeper and more restful sleep, predominates in the first third of the night and tends to occur soon after sleep onset. As noted, REM sleep (R) occurs after 90 to 120 minutes then alternates with NREM at a periodicity of approximately 90 minutes. In a young adult, the percentage of total sleep time is broken down into N1, 2% to 5%; N2, 45% to 55%; N3 (slow-wave sleep), 13% to 24%; and REM, 20% to 25%.
Sleep is regulated through both circadian and homeostatic mechanisms. The circadian rhythm, through a 24-hour cycle of internal pacemaker activity originating in the suprachiasmatic nucleus of the hypothalamus, helps to determine the sleep–wake cycle. This cycle tends to be synchronized to the 24-hour day predominantly by environmental stimuli, specifically light exposure, and can easily be disrupted in an environment devoid of light/dark shifts. Melatonin is a hormone involved in the regulation of the sleep–wake cycle. It is synthesized in the pineal gland and its secretion underlies a strong circadian periodicity designed to promote sleep at night. Maximum secretion tends to be associated with an absence of light, almost exclusively at night between 9 PM and 3 AM, with lowest baseline values between 7 AM and 9 AM [2], thereby increasing the drive to sleep at night. Hormone secretion (e.g., cortisol) and body temperature changes throughout the day are other examples of systems exhibiting circadian activity.
Homeostatic mechanisms also influence sleep–wake cycles. Homeostatic drive is similar to thirst: the longer you are without sleep, the sleepier you become. Voluntary control of sleep onset and prior sleep deprivation enhance the homeostatic drive to sleep, regardless of environmental cues, and also affects sleep regulation.
Sleep onset is associated with a loss of wakefulness stimuli that, even in normal, healthy people is associated with an initial instability in ventilation. N1 sleep is characterized by a decrease in respiratory drive with irregular breathing, decrease in muscle activity, and an increase in upper airway compliance. As sleep progresses through N2 and N3, respirations become stable with a regular rate and tidal volume but a fall in minute ventilation of 0.5 to 1.5 L per minute (approximately 13% decrease) occurs. This is thought to be secondary to resetting of the central chemoreceptor set point that controls the ventilatory drive [3]. The result is a gradual increase in PaCO2 by 4 to 6 mm Hg and a decrease in pH by 0.03 to 0.05 units across the night (Fig. 70.1). On awakening, the central chemoreceptor set point returns to normal and minute ventilation increases.
Sleep is a necessary part of life. Sleep deprivation is associated with decreased or abnormal immune function [4,5], impaired motor and cognitive function [6,7], and has been shown to influence morbidity and mortality outcomes in critically ill patients [8]. Animal data show that extremes of sleep deprivation lead to failure to thrive and eventual death [9]. Metabolic consequences of fragmented sleep in humans are increasingly being recognized. The development of insulin resistance with increased blood pressure, heart rate, cortisol level, and sympathetic activity have been recognized to occur with short-term sleep deprivation. There is an abnormality in thermal regulation and an increase in inflammatory cytokines (tumor necrosis factor-α [TNF-α], interleukin 1 [IL-1], and IL-6) and C-reactive protein, which are known to cause vascular injury and increase insulin resistance [10,11]. This serves to highlight the necessity of good-quality sleep for normal metabolic and physiologic function, especially important in a critically ill population.
Abnormalities of Sleep in the Intensive Care Unit
Abnormal Quantity and Quality of Sleep
Sleep research in the ICU is a murky pond made cloudy by the multitude of confounding variables and questionable reliability of methods used to evaluate sleep during critical illness. The sleep architecture of ICU patients has been well documented to be abnormal. However, there is a great variation in reported abnormalities in both NREM and REM sleep amongst investigators. This may in part be due to the tools used to measure sleep which range from polysomnography (PSG) (the gold standard) to actigraphy, bedside observation, and patient recall. The PSG is expensive and time consuming resulting in the utilization of the other methods to detect sleep. The significant limitations of the other methods include an inability to describe sleep architecture and the occurrence of sleep state misperception. Their strengths include the ability to study large numbers and over extended periods [12]. PSG results using Rechtschaffen and Kales methodology for sleep staging may be unreliable because of discrepancies in analyzing electroencephalographic (EEG) waveforms in part due to distortion of waveforms from medication, underlying illness (e.g., sepsis, encephalopathy), and measurement artifact due to the ICU environment [13,14]. An observational methodologic study evaluating the reproducibility
of manual versus computer-based sleep assessment concluded that more reproducible results of EEG analysis may be obtained with the use of computer-based spectral analysis as opposed to the manual method of scoring [15]. Notwithstanding the above discussion, sleep architecture in the ICU has generally been reported to have higher quantities of lighter N1 and N2 sleep and reduced slow wave and REM sleep. In addition, some patients have abnormal EEG characteristics of stage 2 sleep (absence of spindles and K complexes) [8]. Partial arousal states have been reported; the EEG shows slow-wave sleep but accompanied by movements typical of wakefulness [8]. Studies with 24-hour PSG recordings have found total sleep time to range between 3.6 and 6.2 hours a day in patients both with and without sedatives or hypnotics [16,17,18]. Other studies reveal average total sleep times of 7 to 10.4 hours per day, but with large variations in total sleep time among patients, with some sleeping for less than an hour and others for 12 to 15 hours [8,19,20]. Many studies highlight that patients spend almost equal amounts of time asleep during the day as at night [8,18]. Estimations of patient’s total sleep time based on observation alone tend to overestimate the amount of sleep and have no way of detecting more subtle episodes of sleep disruption [17,21].
of manual versus computer-based sleep assessment concluded that more reproducible results of EEG analysis may be obtained with the use of computer-based spectral analysis as opposed to the manual method of scoring [15]. Notwithstanding the above discussion, sleep architecture in the ICU has generally been reported to have higher quantities of lighter N1 and N2 sleep and reduced slow wave and REM sleep. In addition, some patients have abnormal EEG characteristics of stage 2 sleep (absence of spindles and K complexes) [8]. Partial arousal states have been reported; the EEG shows slow-wave sleep but accompanied by movements typical of wakefulness [8]. Studies with 24-hour PSG recordings have found total sleep time to range between 3.6 and 6.2 hours a day in patients both with and without sedatives or hypnotics [16,17,18]. Other studies reveal average total sleep times of 7 to 10.4 hours per day, but with large variations in total sleep time among patients, with some sleeping for less than an hour and others for 12 to 15 hours [8,19,20]. Many studies highlight that patients spend almost equal amounts of time asleep during the day as at night [8,18]. Estimations of patient’s total sleep time based on observation alone tend to overestimate the amount of sleep and have no way of detecting more subtle episodes of sleep disruption [17,21].
Critically ill patients were found to spend 6% or less of total sleep time in REM [8,20,22]. By contrast, Gabor and colleagues reported REM sleep to be as much as 14.3% of total sleep time [18]. It is unclear if this represented REM rebound from prior ICU-associated sleep deprivation or may have been a rebound from REM-suppressant medication. Patients were also found to experience frequent arousals and awakenings, with investigators reporting a range of 35 to 54 arousals per hour of total sleep time [8,23].
This highlights that not only is sleep in the ICU different from normal in terms of less time spent in deeper stages but the lighter stages of sleep have abnormal EEG patterns of uncertain clinical significance and more frequent arousals throughout total sleep time. Sleep patterns remain altered with continued ICU stay. It may take several days for sleep architecture to normalize after the patient is transferred to the general medical ward [18].
More recently, the effect of continuous versus intermittent sedation versus neuromuscular blocking agents on sleep in mechanically ventilated patients was evaluated. In all groups, there was an increase in total sleep time coupled with an increase in slow-wave sleep of low amplitude. The authors speculated that the increased delta activity may have been the result of a metabolic process or medication effect [24]. This highlights the fact that an elevated total sleep time in the ICU resulting from medication is not identical to physiologic sleep and cannot be assumed to deliver the same restorative function.
The sequelae of abnormal sleep in the ICU ranges from patient perception of excessive sleepiness while in the ICU coupled with an appreciation of poor-quality sleep as compared to home [25] to abnormalities in ventilation and even acute psychoses.
Causes of Sleep Disruption
Noise and Hospital Staff
The environmental stimulus most reported in the literature as disturbing sleep is noise [25,26,27,28,29]. The level of noise in the ICU ranges from 50 to 85 decibels (dB) through a 24-hour period [26,27,28,29]. This level of noise is comparable with a busy office (70 dB) or a pneumatic drill heard 50 ft away (80 dB) [28,29,30]. These noise levels exceed that recommended by the US Environmental Protection Agency for hospital settings suggested to be 45 dB during the day and 35 dB at night [31]. The sources of noise as identified by ICU patients include equipment and conversation among staff [24]. Noise may disrupt sleep in two ways: either arouse/awaken the patient or produce a change to a lighter sleep stage. There is some debate, however, as to what degree the noise causes the arousal or whether noise may simply coincide with many of the frequent awakenings ICU patients experience.
Reports have suggested that the response to noise may vary based on the age and gender of the patient. Healthy men and women were monitored for changes in sleep stages with EEG in a controlled environment. The environmental noise in these studies included simulated sonic booms and jet aircraft flyover noise. Older individuals and women had lower sleep thresholds and were more likely to be awakened or shift to a different sleep stage [29]. However, findings in healthy subjects may not apply to critically ill patients whose sleep threshold is altered by sleep deprivation, drugs, or encephalopathic states. One study showed no difference in perceived ICU sleep quality or daytime sleepiness by way of gender nor were there significant correlations between perceived sleep quality and age or length of ICU stay. However, in this study, information was gained by way of a sleep questionnaire without EEG monitoring [25].
Freedman et al. [25], using a questionnaire distributed to ICU patients on the day of their discharge from the unit, showed perceived differences in individual environmental factors in terms of their degrees of ICU sleep disruption. Checking vital signs and phlebotomy were perceived as most disruptive. These were followed by noise, diagnostic tests, nursing interventions (e.g., bathing), ambient light, and medication
administration. In terms of environmental noise, talking and telemetry alarms were perceived as significantly more disruptive to sleep than the phone or beepers. The investigators also compared perceived sleep disruption between patients discharged from medical, surgical, and cardiac ICUs and concluded that medical ICU patients perceived their sleep to be more disrupted by environmental factors than did patients in other units. The study’s design was again limited by lack of EEG verification of sleep architecture and the lack of control for severity of illness and medications used. This study highlighted that environmental noise, although an important contributor to sleep disruption, is but one of many environmental factors causing poor sleep in the ICU.
administration. In terms of environmental noise, talking and telemetry alarms were perceived as significantly more disruptive to sleep than the phone or beepers. The investigators also compared perceived sleep disruption between patients discharged from medical, surgical, and cardiac ICUs and concluded that medical ICU patients perceived their sleep to be more disrupted by environmental factors than did patients in other units. The study’s design was again limited by lack of EEG verification of sleep architecture and the lack of control for severity of illness and medications used. This study highlighted that environmental noise, although an important contributor to sleep disruption, is but one of many environmental factors causing poor sleep in the ICU.
Another study using a questionnaire administered to ICU patients within the first week of their stay reported that the physical stressors patients considered the most important included pain and inability to sleep due to noise and nasogastric and endotracheal tubes. Loss of self-control, autonomy, and lack of understanding about attitudes and procedures were the main psychological stressors [32].
Does reduction in noise levels result in better sleep in the ICU? Investigators evaluated sleep outcomes in 18 female patients in an acute obstetrics/gynecologic unit and found a statistically significant improvement in subjective outcome measures in those who wore earplugs as compared with those who did not [33]. A PSG study in healthy volunteers randomly exposed to either simulated ICU noise or earplugs during sleep showed an increase in sleep disruption associated with ICU noise as compared with use of earplugs. There was also an increase in REM sleep in those using earplugs [34]. Whether it is the peak noise level or a change in the noise level that causes sleep disruption is unclear. Investigators added white noise to the background noise and reported a decrease in arousal frequency from 48 per hour to the no noise baseline of 13 per hour [35]. This supports the thought that maintenance of a certain level of unobtrusive background noise in the ICU may be helpful in reducing arousal frequency.
Sleep in the ICU is poor in terms of quality and lack of continuity. Nursing care activities, phlebotomy, noise, the presence of invasive tubes, pain, and patients’ lack of understanding of their medical care all contribute to the poor sleep. Despite this, the cause of 68% of arousals could not be identified by Gabor and coworkers [18] in a PSG study assessing noise as a cause of disrupted sleep in seven mechanically ventilated patients. Other potential culprits include underlying medical illness, mechanical ventilator mode, and medications.
Table 70.1 Drugs Commonly Used in Intensive Care Units and Their Effects on Sleep Pattern | ||||||||||||||||||||||||||||||||||||||||||||||||||||||
---|---|---|---|---|---|---|---|---|---|---|---|---|---|---|---|---|---|---|---|---|---|---|---|---|---|---|---|---|---|---|---|---|---|---|---|---|---|---|---|---|---|---|---|---|---|---|---|---|---|---|---|---|---|---|
|

Full access? Get Clinical Tree
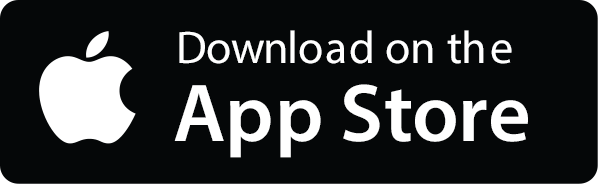
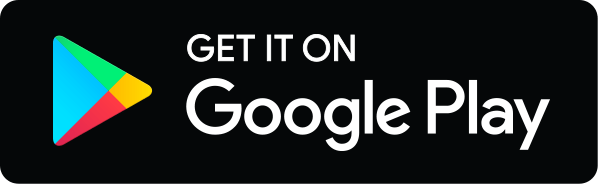