KEY POINTS
Sleep is necessary for life, and disrupted sleep and circadian rhythmicity have been linked to a variety of adverse health outcomes. It is likely that critically ill patients are harmed by poor sleep, although evidence to support this contention does not yet exist.
Until further research is conducted, recommendations for improving sleep in critically ill patients are drawn upon limited evidence and extrapolations from other patient populations.
Critically ill patients exhibit disordered circadian timing, which may contribute to poor sleep and adverse health outcomes. These abnormalities may reflect an inability of critically ill patients to synchronize their internal clocks to the ICU environment, although it is likely that acute illness and medications also contribute to these abnormalities.
Exposure to bright light during the day (particularly the morning) and avoidance of nocturnal light exposure may strengthen circadian rhythmicity and promote sleep.
Patients receiving continuous intravenous sedation are likely at increased risk of disordered circadian rhythmicity due to low retinal light exposure from sedative-induced eye closure.
The typical ICU environment is hostile to sleep, consisting of excessive light and noise at night, insufficient light during the day, and frequent sleep disruption due to monitoring and patient care activities.
In general, the sleep of critically ill patients is highly fragmented and nonconsolidated, and rapid eye movement (REM) sleep is scarce.
Sleep and mechanical ventilation is a bidirectional interaction in which changes in the level or pattern of mechanical ventilatory support may affect sleep, while changes in sleep state affect respiration and therefore patient-ventilator interaction.
Patients receiving mechanical ventilation are susceptible to sleep disruption if air hunger is not sated, if neural and mechanical inspiratory times differ, if periodic breathing is induced, and probably through other mechanisms as well.
Sleep fragmentation from periodic breathing is most likely to occur when pressure support ventilation is used, particularly if the patient has also heart failure. Proportional assist ventilation may also induce periodic breathing and sleep fragmentation, but appears less likely to do so. Volume-cycled assist control ventilation may be the mode of ventilation that is least likely to disrupt sleep in critically ill patients.
Traditional sleep scoring criteria are unreliable in assessing sleep in mechanically ventilated patients receiving intravenous sedation.
Patients receiving continuous mechanical ventilation and continuous intravenous sedation exhibit pronounced temporal disorganization of the sleep EEG. The extent to which sedation is itself restorative is not clear.
Sleep may play an important role in the short- and long-term physical, cognitive, and psychological recovery of recent survivors of critical illness.
Absent high-quality evidence to guide the clinician, efforts to enhance sleep, and circadian rhythmicity in the ICU should focus initially on relatively low-hanging fruit: for instance, administering baths during the daytime or early evening hours, and ensuring adequate light exposure during the day.
INTRODUCTION
It is still not clear exactly why we sleep, although there are many theories. What is clear is that sleep is a ubiquitous and highly conserved evolutionary process in nature, with cyclic alternations of rapid eye movement (REM) sleep and nonrapid eye movement (NREM) sleep being found in every mammal studied to date, and with variations of the rest-activity cycle found throughout nature.1 We spend roughly one-third of our time asleep; in the words of the sleep research pioneer Allan Rechtschaffen, “If sleep does not serve an absolutely vital function, then it is the biggest mistake the evolutionary process has ever made.” In fact, a rapidly accumulating body of evidence indicates that sleep is not only necessary for maintaining alertness and performance but is highly involved in processes as diverse as metabolism, heart disease, and cancer surveillance.2-6
It is therefore somewhat surprising that the sleep of critically ill patients has received so little attention to date. Every year in the United States more than 5 million persons are admitted to intensive care units, nearly 1 million of whom will receive mechanical ventilation. All of these patients have a biological need to sleep. If the sleep of critically ill patients matters even a little in overcoming critical illness, treatment strategies that improve sleep quality have the potential to save thousands of lives annually while simultaneously reducing costs to society. Even if sleep quality were wholly irrelevant to survival in this setting—and this would be surprising indeed—sleep disruption is in itself distressing to patients, and therefore worthy of our efforts to ameliorate this problem.
Unfortunately, progress in understanding the sleep and circadian rhythms of critically ill patients has been slowed by a variety of logistical, technical, and methodological challenges (Table 23-1). Fortunately, the engagement of increasing numbers of researchers from diverse disciplines has begun to bear fruit.
Challenges to the Study of Sleep in Critical Illness
|
NORMAL SLEEP: A BRIEF REVIEW
Sleep and wakefulness are regulated by two basic processes: the homeostatic process (process S) and the circadian process (process C).7,8 The homeostatic drive to sleep increases with increasing duration of wakefulness and is dissipated by sleep. It appears that process S is mediated at least in part by the neurotransmitter adenosine, the product of adenosine triphosphate (ATP) metabolism, which accumulates in the arousal centers of the brain with prolonged neural activity. Once a certain threshold is passed, drowsiness and/or sleep ensue, thereby homeostatically adjusting the amount of sleep experienced by the organism. In contrast, the circadian process, or process C, is an endogenously driven and roughly 24-hour cycle that is largely independent of process S, and which is characterized by alternating periods of high and low sleep propensity. The circadian timing system helps maintain wakefulness throughout the day as the homeostatic drive to sleep increases. Similarly, the nadir in circadian sleep propensity during the early morning hours helps consolidate sleep. Circadian processes are also involved in the regulation and expression of a multitude of biological processes related to metabolism, cardiovascular regulation, cognition, and immune function, as considered later.
Synchronization of the human endogenous circadian timing system to the external environment is chiefly accomplished through exposure to light,9 allowing individuals to adjust their rest and activity cycles to the light-dark cycle and to the activities of their community
While individual requirements for sleep vary, a typical night’s sleep for a healthy young adult consists of an essentially uninterrupted 8-hour period of sleep comprised of 90-minute periods of alternating REM sleep and NREM sleep. NREM sleep is divided into three stages of sleep of progressively increasing depth, from stage N1 (transitional sleep or drowsiness) to stage N2 to stage N3 (deep or slow wave sleep [SWS]).10 The sleep electroencephalogram (EEG) of the latter consists of ≥20% delta waves and includes stages 3 and 4 in the prior classification scheme. REM sleep comprises approximately 20% to 25% of total sleep time and is associated with dreaming, skeletal muscle atonia, rapid eye movements, muscle twitches, and cardiovascular and respiratory instability. Healthy adult subjects who are not sleep deprived typically progress from wakefulness to NREM sleep, beginning in the lighter stages and progressing to deeper stages. The first episode of REM sleep typically occurs approximately 70 to 100 minutes after sleep onset and lasts fewer than 10 minutes. Subsequent episodes of REM sleep are progressively longer in duration and occur in alternation with bouts of NREM sleep at approximately 90-minute intervals, for a total of 4 to 5 sleep cycles per night. Thus, SWS predominates in the first part of the night while REM sleep predominates in the latter part of the night, coincident with the circadian trough in body temperature.
In healthy young adults, SWS constitutes approximately 20% of total sleep time. Normal aging is associated with compromised sleep that is manifest in a variety of ways, including marked decreases in SWS, greater sleep fragmentation, and earlier awakening (Fig. 23-1).11,61 In contrast, REM sleep is generally well preserved throughout life, with significant declines in the elderly typically being associated with organic brain syndromes or medications.
Sleep deprivation is considered to be present when the quantity or quality of sleep is insufficient for maintaining alertness, performance, and emotional and physical health. While it is thought that most individuals require approximately 8 hours of sleep for normal functioning, individual sleep requirements vary. While acute sleep deprivation may occur, it is more common in modern society for patients to experience chronic partial sleep deprivation as a result of voluntary sleep curtailment. Other causes of sleep deprivation and of sleep disruption generally are numerous and include insomnia, poor sleep hygiene, sleep disordered breathing, parasomnias, medical and psychiatric illnesses, pain, medication, and excessive light and noise. Disorders of circadian timing may disrupt sleep by causing a misalignment between the circadian alerting signal and the homeostatic sleep mechanism, a phenomenon that is familiar to anyone who has experienced jetlag or been employed as a shift worker. It is also possible for total sleep time to be normal but for the patient to be deprived of the more restorative stages of sleep, such as SWS and REM sleep.
Central to the problem of measuring sleep in critically ill patients is the existence of a variety of pathologic states that confound classification into the normal categories of wakefulness, NREM sleep, and REM sleep. These pathologic states may be a result of illness, medications (particularly sedatives and narcotics), and disorders of circadian timing, as discussed below.
Sleep elicits physiologic responses throughout the body that vary according to sleep stage and timing. Normal respiration provides an example of the dynamic nature of sleep. Overall, while both alveolar ventilation and the ventilatory responses to carbon dioxide and to hypoxia are reduced during sleep, the pattern of respiratory output varies according to sleep stage. Drowsiness or sleep onset is characterized in many subjects by an unstable breathing pattern, an overall decrease in ventilation and, in some subjects, the occurrence of apneas.12 Subsequently, breathing in deeper stages of NREM sleep—namely SWS—becomes more regular. In contrast, breathing during REM sleep is typically more irregular, and both tidal volume and respiratory rate can vary considerably. REM sleep is also associated with a reduction in upper airway dilator tone,13 contributing to the greater tendency for obstructive respiratory events to occur during this stage. The effects of sleep on respiration may contribute to ventilator dyssynchrony and to sleep disruption, as considered below.
The cardiovascular system also changes dynamically throughout sleep.14 As with respiration, the cardiovascular system is relatively stable during NREM sleep, a stage characterized by autonomic stability and an increase in parasympathetic tone. Blood pressure and pulse both tend to decrease, and the normal fluctuation in heart rate with breathing— normal respiratory sinus arrhythmia—is augmented. In contrast, REM sleep causes autonomic and cardiovascular instability as reflected by fluctuations in heart rate and blood pressure. Surges in parasympathetic tone may result in sinus pauses and episodes of bradycardia, while surges in sympathetic outflow increase heart rate and blood pressure. While firm data are lacking, such changes may provoke cardiac arrhythmias and alterations in blood flow and myocardial oxygen demand that lead to coronary and cerebrovascular events.
Body temperature, and thermoregulation generally, is subject to both sleep and circadian influences. Normally, body temperature reaches its nadir in the early morning hours, begins to rise before waking, and peaks in the late afternoon. Body temperature is less tightly controlled during NREM sleep than during wakefulness, while in REM sleep the thermoregulatory responses are essentially absent. Ambient temperature, in turn, influences the quantity and quality of sleep, with maximal sleep propensity occurring at thermoneutrality. REM sleep is more sensitive than NREM sleep to disruption from heat or cold.
A variety of metabolic and endocrine processes are regulated by sleep and by circadian processes. For instance, prolactin secretion increases dramatically during sleep and declines acutely with awakenings. The secretion of growth hormone is similarly enhanced by sleep, while cortisol and thyrotropin secretion are inhibited. Several compelling lines of evidence link sleep deprivation, including chronic partial sleep deprivation experienced by a significant segment of modern society to insulin resistance and weight gain.15 The study of the effects of disrupted sleep and circadian rhythmicity on endocrine processes in critical illness constitutes an exciting avenue of future research.
Of course, the restorative processes conferred by sleep that have long been most obvious are those conferred on the brain. Sleep restores alertness and vigilance and enhances memory consolidation; while sleep deprivation worsens mood, vigilance, reaction time, and cognitive and task performance.2
In a series of experiments performed by Alan Rechtschaffen, rats subjected to total sleep deprivation died within several weeks.16 While no similar such experiment has been performed in humans, disrupted sleep and circadian rhythmicity have been linked to a host of basic physiologic processes and to a variety of adverse health outcomes. While it is surely true that “Sleep is of the brain, by the brain, and for the brain,”17 it is increasingly clear that sleep is for the whole body as well.
Currently, there are no data demonstrating that the sleep or circadian rhythms of critically ill patients influences either their short-term or long-term outcomes. It is also worth noting, however, that the absence of an effect has not been shown. Based on extrapolation from other populations, sleep disruption could have a myriad of adverse effects on the critically ill patient (Table 23-2). Consider the following hypothetical scenario for a patient admitted to the ICU. In the acute setting, severe sleep deprivation causes the patient to be lethargic and encephalopathic with increased sensitivity to sedatives and narcotics and a depressed ventilatory response to CO2, frustrating physician attempts to liberate him or her from the ventilator. Subsequently, sleep deprivation reduces insulin sensitivity and glycemic control and impairs the immune response, predisposing the patient to infection. After discharge, the profound sleep debt incurred by the patient during critical illness persists as a result of physical illness, anxiety, and medication effect. This sleep deprivation worsens her mood and motivation, reduces her energy expenditure and physical activity, impairs her executive function and memory consolidation, and leads to long-term psychological distress and neurocognitive dysfunction. This hypothetical example is biologically plausible based on extrapolations from basic investigations and from studies in other patient populations but awaits confirmation in the critically ill patient.
Potential Harm From Sleep and Circadian Disruption During and After Critical Illness
|
CIRCADIAN RHYTHMICITY IN THE CRITICALLY ILL PATIENT
Organisms have acquired during evolution a variety of adaptations to their external environment that promote survival. One of the most conspicuous characteristics of the external environment on Earth is the presence of a roughly 24-hour light-dark cycle. Organisms throughout nature therefore developed the ability to synchronize their activities to the light-dark cycle through an endogenously driven timekeeping mechanism that is periodically “reset” to solar time. In mammals, the hypothalamic suprachiasmatic nucleus (SCN) is the central pacemaker for these circadian (derived from the Latin terms circa, around, and diem, day) rhythms. Circadian rhythms can be found in a host of physiological and behavioral processes ranging from somatotropic functions to temperature regulation to endothelial function and to countless other processes. The central pacemaker is synchronized (ie, “reset”) to the external environment daily chiefly through photic cues transmitted through the retinohypothalamic tract to neurons in the SCN.18,19 This process of modifying an organism’s intrinsic rhythm in order to align it with the external environment is called entrainment. Although there are other Zeitgebers (“timegivers”) including food, social interactions, and ambient temperature, these are less potent than light.
Neurons from the SCN project to the pineal gland, which secretes melatonin in a rhythmic pattern that is primarily determined by the SCN and its circadian rhythm. Melatonin thus serves as a practical “hand of the clock,” allowing investigators to determine the circadian rhythm of the central pacemaker by analyzing the 24-hour secretion pattern of melatonin. Normally, serum melatonin levels are high during the subjective night and lower during the subjective day, and a similar pattern is seen in the urinary metabolite of melatonin, 6-sulfatoxymelatonin.20 Bright light during the subjective night acutely suppresses melatonin production, an effect that is independent from light’s phase-shifting effects. Melatonin itself has a variety of pharmacologic properties and has receptors throughout the brain and in a variety of peripheral tissues. The physiological functions of melatonin itself are still being clarified.21

Full access? Get Clinical Tree
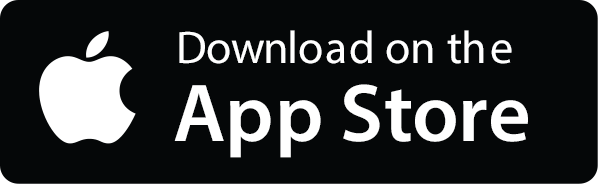
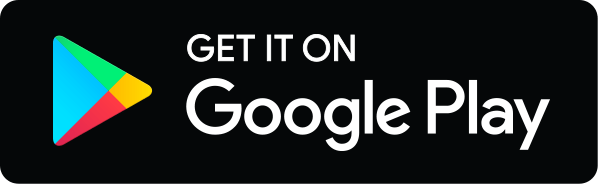