Fig. 35.1
Real pediatric survival rates (right scale) related to the number of mock codes (left scale). CPA cardiopulmonary arrest (Reprinted with permission from Andreatta et al. [46])
Discipline-Specific Curricula
General and In-Patient Pediatrics
Pediatric training programs have struggled to provide adequate exposure to acute care cases and opportunities for advanced procedural skills for residents in training. Traditional “mock code” programs with static mannequins lacked realism and relied heavily on the instructor to provide timely feedback during the scenario. Over the years, educators have recognized the benefits of simulation and how a higher level of environmental and physical realism can enhance the learning outcomes for trainees of all levels. Simulation allows participants to practice in an environment that closely mimics real life, thus allowing learners to be fully immersed and engaged in the learning experience [47].
Recently, leaders in pediatric resuscitation education have incorporated more simulation-based education into both the Neonatal Resuscitation Program (NRP) and the Pediatric Advanced Life Support (PALS) course. The use of increased realism and debriefing in this context will provide pediatric healthcare providers the foundation of knowledge and skills necessary to look after acutely ill children. The growth of simulation around the world has also lead to the genesis of novel simulation curricula that have been integrated into preexisting pediatric curricula. These curricula have been developed to enhance three primary skill sets: cognitive skills (content knowledge), technical skills, and crisis resource management.
Pediatric simulation educators recently developed a curriculum which integrated modular, training year-specific simulation courses with a longitudinal just-in-time mock code program. The program was developed based on a thorough needs assessment, which included a comprehensive review of Royal College of Physicians and Surgeons of Canada (RCPSC) and Accreditation Council for Graduate Medical Education (ACGME) objectives for pediatric residency training. Three year-specific simulation-based courses were then developed and implemented which were run once a year for residents in years 1, 2, and 3 of pediatric residency training. These 1-day courses included a mix of learning formats—including lecture, high-realism simulation, debriefing, and small group discussions. Table 35.1 outlines the curriculum content for each year-specific course [48].
Table 35.1
Pediatric simulation curriculum outline
Longitudinal just–in–time mock codes | ||
Realistic, multidisciplinary response to acute deterioration of patients | ||
Year–1 course | Year–2 course | Year–3 course |
Critical first 5 min | Complex medical management | Crisis resource management |
Knowledge | Knowledge | Knowledge |
Respiratory failure | Rapid sequence intubation | Leadership |
Shock | Inotropic support | Communication |
Seizures | Postarrest management | Resource management |
Situational awareness | ||
Skills | Skills | Skills |
Bag-valve-mask ventilation | RSI and intubation | Code team leader role |
IV fluid setup and administration | Intraosseous needle insertion | Post-resuscitation debriefing |
Chest compressions | ||
Simulation cases | Simulation cases | Simulation cases |
Hypovolemic shock | Status asthmaticus | Status asthmaticus |
Bronchiolitis | Septic shock | Septic shock |
Anaphylaxis | Status epilepticus | Status epilepticus |
Status asthmaticus | Cardiogenic shock | Cardiogenic shock |
Trauma | ||
Aspiration pneumonia | ||
Status epilepticus |
In addition to the modular, year-specific courses, the curriculum included longitudinal in situ just-in-time mock codes using high-realism simulation in an interprofessional setting. The topic of the mock code was determined by selecting the sickest patient on the clinical teaching unit, and simulating their status should they deteriorate. This approach allowed the residents (and the entire HCP team) to be prepared for a potential deterioration of that patient, familiarized them to the equipment for resuscitation, and provided them the opportunity to practice in a multidisciplinary team-based environment. The use of pediatric mock codes is reviewed further in section “Pediatric Critical Care Medicine”.
Pediatric Emergency Medicine
There has been a significant implementation of simulation-based learning in pediatric emergency medicine (PEM) training. Adler et al. described the implementation of a 1-day, six-case simulation-based pediatric emergency medicine curriculum and concluded that focused, frequent, and effortful instructional interventions are necessary to achieve substantial performance improvements [49]. A longitudinal approach to the integration of simulation into the PEM curriculum was taken by Cheng et al. who developed a package of 43 different acute care scenarios that were delivered over 2 years of PEM training, packaged as 12 different core and subspecialty modules. Each module, consisting of three or four simulation cases, was designed to be delivered over 3–4 h, with learning targeted at a specific theme or specialty. Knowledge, technical skills, and behavioral skills were discussed and reviewed after each simulation with a 30–40-min facilitated debriefing session. Although trainee performance and patient outcomes were not measured after the implementation of the curriculum, those PEM residents participating in the training reported very high satisfaction ratings for this method of acute care education. Table 35.2 provides an overview of the curriculum, along with a description of the cases in each module [50]. Other centers have used simulation to improve the performance of pediatric trauma teams noting improved subsequent performance in the simulated scenarios [35].
Table 35.2
Pediatric emergency medicine curriculum outline: scenario list by modules
Year 1 | Core modules | Total of 23 scenarios |
Respiratory emergencies | Status asthmaticus, aspiration pneumonia, upper airway obstruction, acute chest syndrome | |
Cardiac emergencies | Supraventricular tachycardia, unstable ventricular tachycardia, ventricular fibrillation, PEA/asystole | |
Shock | Septic shock, hypovolemic shock, anaphylactic shock, cardiogenic shock | |
Blunt trauma | Abdominal trauma, major head injury, orthopedic trauma, thoracic trauma | |
Environmental emergencies | Near drowning/hypothermia, electrical injury, smoke inhalation/carbon monoxide poisoning | |
Infant/neonatal emergencies | Nonaccidental injury, bronchiolitis, congenital diaphragmatic hernia, congenital heart disease | |
Year 2 | Subspecialty modules | Total of 20 scenarios |
Toxicology emergencies | Sympathomimetic, anticholinergic, cholinergic and opioid toxidromes | |
Endocrinological emergencies | Diabetic ketoacidsosis, adrenal crisis, thyroid storm | |
Oncological emergencies | Mediastinal mass, hyperleukocytosis/stroke, tumor lysis syndrome | |
Nephrological emergencies | Hypertensive emergency, acute renal failure/hyperkalemia, hyponatremia | |
Neurological emergencies | Status epilepticus, coma/depressed level of consciousness, combative/encephalopathy | |
Penetrating trauma | Thoracic trauma, neck trauma, spinal cord trauma, abdominal trauma |
Neonatal Intensive Care
Simulation-based training is gaining momentum in neonatal intensive care units (NICU) across the world. Although neonatal resuscitation is common, neonates requiring aggressive resuscitation at birth are rare. This low-frequency but potentially high-risk event lends itself well to simulation-based training [51]. The traditional NRP program improves cognitive and technical skills but gaps in behavioral skills and other areas have been identified [52–56] and knowledge retention is poor [57]. Simulation is now tightly integrated into the NRP curriculum [58].
Research to determine the impact of simulation-based education on neonatal resuscitation is accumulating. Two studies have explored the use of a single high-fidelity simulation session compared to either low-fidelity simulation or self-study and found no difference in multiple choice examination scores post-learning though the high-fidelity groups did require less prompting during simulated resuscitations [59, 60]. These studies are limited by the use of a single simulation session and only cognitive knowledge as the outcome variable. More frequent simulation sessions may be required to improve knowledge transfer [61].
Simulation is also being developed and used in novel methods for neonatal intensive care. It has been studied as a “booster” to previous NRP training to remote communities to prevent knowledge decay [62]. A group consortium has formed to develop a “living text book” and “living world” for neonatal nurse practitioner education [63]. Simulation is also being used as part of a larger initiative to improve communication in the labor and delivery environment and during end-of-life care [64, 65].
Research evaluating the impact of neonatal simulation on patient outcome is limited to date. One study in neonatal airway management taught to pediatric residents using a single session revealed that although the learners demonstrated improved skills on a neonatal mannequin, a benefit in actual clinical performance was not found (although the study was likely underpowered for this outcome) [66]. In a landmark study, Draycott et al. developed an annual simulation-based training intervention for obstetrical and neonatal teams [67]. Their intervention decreased the incidence of newborn hypoxic-ischemic encephalopathy from 27.3 to 13.6 per 10,000 births over a 5-year period, demonstrating the potential benefit of simulation-based training on actual patient outcomes. Future neonatal simulation studies need to continue to evaluate changes in actual clinical performance.
Pediatric Critical Care Medicine
The challenges that exist in the neonatal intensive care environment are mirrored in the pediatric intensive care unit (PICU). In a recent survey of 76 pediatric residents, it was clear that although many of them were involved in the resuscitation of an acutely ill child, they were not adequately trained to respond, especially in basic life support and the use of a defibrillator [9]. These gaps were noted again in a simulator-based study evaluating the performance of pediatric residents during a resuscitation and in airway management [68]. Given these studies one could consider it unethical to solely rely on clinical experience to educate our trainees in pediatric resuscitation.
A number of simulation-based curricula have been developed and studied in pediatric critical care. Nishisaki and colleagues developed a multi-institutional “boot camp” for PICU fellows [69]. Learners participated in whole and small group discussions, task training, and high-fidelity simulation sessions covering airway management, vascular access, sepsis, resuscitation, traumatic brain injury, and delivering bad news. In the latter half of the course, each simulation session was followed by a debriefing session and then a second simulation scenario similar to the first to allow for deliberate practice. On a follow-up survey, participants reported increased perceived confidence and competence following the training. The use of the “train-to-success” strategy significantly improved the learners’ perception of the training effectiveness.
Education provided just prior to a clinical event may be more effective than standard courses. A “just-in-time” simulator-based refresher training on airway management in the PICU was developed and clinical outcomes assessed [70]. Although this approach had no effect on first-time intubation success rate by pediatric residents, there was increased resident participation as the initial laryngoscopist with no concomitant increase in adverse events when compared to historical controls. Such training may make senior PICU staff more comfortable in allowing junior trainees to perform tasks in the PICU. A similar study examined frequent just-in-time simulator training in CPR (Fig. 35.2). It was well received by staff and those that were refreshed ≥2/month demonstrated significantly shorter time to effective CPR on subsequent mannequin assessment [71]. Subsequent studies demonstrated that instructor-led mannequin-based CPR booster sessions of pediatric ward staff could improve CPR skill acquisition rates and maintain retention of CPR skills [72, 73]. The use of frequent simulation-based updates may be a useful method for providers to maintain their skills in dealing with rare events, such as pediatric intubation and cardiac arrest.
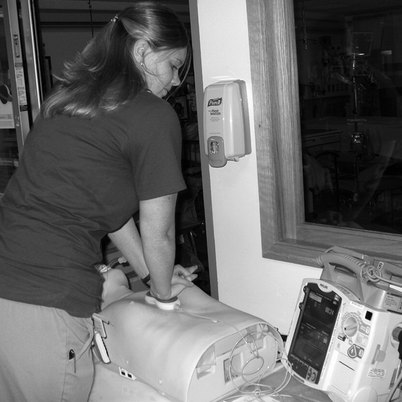
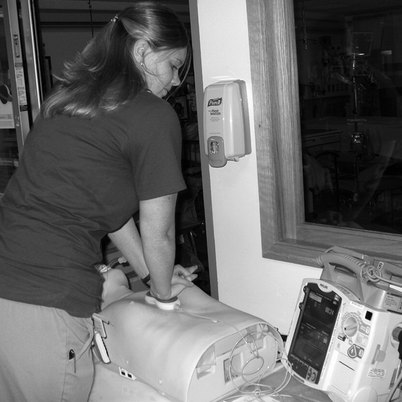
Fig. 35.2
“Rolling Refresher” CPR skill training using “just-in-time” and “just-in-place” simulation (Reprinted from Niles et al. [71]. Copyright 2009, with permission from Elsevier
In order to increase availability of simulation in critical care, in situ simulation is becoming more prevalent, either permanently located in the PICU, or mobile systems, useable virtually anywhere [74, 75]. One popular use of this technique is mock codes in which resuscitation teams are called to surprise simulations [76]. These exercises can identify learning needs or systemic latent errors that need to be addressed [26, 77]. Niebauer et al. found a very high incidence of hyperventilation by healthcare providers during mock resuscitations with a mean ventilation rate of 40.6 breaths per minute, a problem which has recently been confirmed in the clinical environment [78, 79]. Hunt and colleagues found that there were deviations from standardized basic life support protocols in 75% of resuscitations [28]. These programs have the potential of a large clinical impact as demonstrated by Andreatta’s study discussed previously.
Pediatric transport is another domain using simulation-based training. Although research in this area to date is limited, the development of untethered portable pediatric mannequins allows simulation-based training to be done in multiple environments including elevators, ambulances, and helicopters.
As cardiac intensive care develops as a separate subspecialty of pediatric critical care, simulation has also been incorporated there as an educational technique. Several studies have demonstrated increased learner self-confidence in resuscitation following simulation training [39, 80]. Pye and colleagues used simulation to introduce parental presence during resuscitation to their cardiac ICU staff [81].
In addition to more traditional simulations, cardiac-specific interventions have been studied. An echocardiography trainer for identification of various pediatric congenital heart defects has been developed, and though some technical challenges remain, it was felt to be realistic and useful [82]. Lo and colleagues have developed a reusable chest opening task trainer and have shown improvement in team skills with serial simulations of urgent sternal opening in a decompensating postoperative patient [83].
Simulation-based training has also been used in extracorporeal life support and continuous renal replacement therapy (CRRT). Lopez-Herce and colleagues used a multimodal educational intervention including high-fidelity simulation, didactic lectures, and task training with both CRRT and peritoneal dialysis machines. Their intervention improved cognitive measures and performance by learners as demonstrated by MCQ and simulated performance assessment [84]. Conventional pediatric mannequins have also been modified to interact with extracorporeal membrane oxygenation (ECMO) circuits [85]. Simulation-based training with these modified simulators has improved performance in the simulated environment as well as some modest improvements in the clinical environments [86, 87].
Pediatric Surgery and Pediatric Anesthesia
Another domain adopting simulation as an educational tool is pediatric surgery. It has been used to develop cognitive and behavioral skills as well as more specific surgical task training. Various task trainers using physical models and virtual reality have been developed to allow surgical trainees to develop competence in such procedures such as bronchoscopy, gynecological examinations, cleft lip repair, and laparoscopic surgery [88–92]. Patient-specific anatomy can also be created in a task trainer to allow practice prior to complex surgeries, such as repair of congenital heart conditions [93]. However, widespread adoption for task training has been somewhat limited by the relative lack of pediatric-specific mannequins and a recent survey of pediatric surgical trainees and program directors noted that currently available simulators may not be adequate to improve their skills [94]. More work is required to create more realistic surgical task trainers. In a recent survey of pediatric surgical trainees and their program directors, although 86.2% of respondents felt that simulation-based minimally invasive surgery training improves training efficiency, only 31.1% actually felt that current simulators had actually improved their skills [94]. In the adult population, the use of simulator-based minimally invasive surgery training did reduce error rates in the clinical environment [95].
Simulation has also been used to improve surgical team communication. Auguste reported using simulation to prepare surgical teams for a hospital’s first EXIT procedure for a baby with hypoplastic left heart syndrome [96]. Surgical handover is another area where errors are common and simulation is being used to train residents in strategies to improve the quality of handover [97].
Although simulation has been used prominently in adult anesthesia pediatric data is limited [98, 99]. Howard-Quijano et al. used simulation to evaluate the ability of pediatric anesthesia trainees to manage intraoperative cardiac arrest [100]. Shavit and colleagues assessed a simulation-based sedation course that they developed and noted that simulation-trained physicians demonstrated improved performance in actual patient sedations [101]. However, more research is clearly needed.
Adolescent Medicine/Psychiatry
A final area where the use of simulation has been adopted as an important educational tool is in the area of adolescent medicine. In particular, issues pertaining to the assessment of mental health have been explored and measured. The simulation used in this group is exclusively standardized patients (SP). Some programs have even started to train and use actual adolescents in the role of SP. The use of actual adolescents as SP has been evaluated positively by students interacting with adolescents in this role [102]. However, authors warn that the adolescents must be chosen carefully and trained well [103].
In general, using standardized patients to practice interviewing and counseling skills has a positive effect on students. These students have been shown to score significantly higher on both measures of knowledge and clinical skills [104]. Additionally, the use of standardized patients has been evaluated in both medical students and residents who have been trained in programs that supplemented traditional mental health teaching with simulated interactions with a standardized patient in the role of an adolescent with mental health issues. Several studies have shown positive effects in the areas of knowledge, clinical skills, confidence, and interpersonal skills. These positive effects have been shown in the mental health areas of major depressive disorders and suicide risk assessment [105, 106]. The future of simulation in this patient group will focus on other mental health conditions. More research is needed into the factors that improve the selection and training of adolescents to play the role of SP.
Team Training in Pediatrics
As specialized teams deliver increasing amounts of pediatric care, the importance of teamwork is receiving increased attention. Greater evidence is becoming available on the widespread prevalence of human factor errors during pediatric resuscitations [26, 28] and the importance of good teamwork skills in improving resuscitation team performance [54]. These skills are not explicitly taught in standard training programs. An alert from the Joint Commission for the Accreditation of Healthcare Organizations (JCAHO) noted that of 47 cases of neonatal mortality or severe morbidity reviewed, ineffective team dynamics played a role in almost 75% of them [107]. The Commission recommended that all healthcare organizations provide team training, clinical drills, and debriefings to evaluate team performance and identify gaps. Simulation-based training provides an opportunity to provide this education [108] and has been recommended by the International Liaison Committee on Resuscitation [109].
To date, there is little data on the use of team training in pediatrics. Gilfoyle and colleagues developed a workshop with a plenary session followed by simulation sessions to teach teamwork skills to pediatric residents [42]. They demonstrated that residents had improved teamwork skills both following the initial training and in a 6-month follow-up when compared to a control group. A similar improvement in collaboration between physicians and nurses was noted after multidisciplinary simulation sessions in another study [110].
A more recent study randomized participants into three groups: standard NRP, standard NRP plus 2 h of team training or high-fidelity NRP with team training [111]. As expected, participants receiving team training demonstrated more team skills, and this improvement was maintained at 6-month follow-up. However, team training with low-fidelity simulation did not improve teamwork skills as compared to control suggesting the value of fidelity in simulation-based training. Riley and colleagues developed a perinatal team training intervention based on the Team STEPPS® program developed by the Agency for Healthcare Research and Quality [112]. A didactic-only and simulation plus didactic education program was compared to control in this small cluster randomized trial. The simulation-based cluster had a 37% reduction in neonatal adverse outcomes. No improvement was noted in the didactic-only group when compared to control. These studies demonstrate that team training can improve patient outcomes, but didactic training alone is not enough.
Assessment Tools in Pediatrics
Many evaluation tools have been developed for use in simulation-based education. These assessment tools have been developed to evaluate clinical performance, leadership skills, and teamwork skills. Several assessment tools to evaluate crisis resource management (both team and leader performance) were recently reviewed in the literature [113]. However, until recently, very few of these tools have been developed or validated in pediatrics. Table 35.3 summarizes a variety of assessment tools developed in pediatrics.
Table 35.3
Pediatric assessment tools
Author | Focus of assessment | Environment where studied/validated | Scale used | Psychometrics measured/reported |
---|---|---|---|---|
Donoghue et al. [114] | Clinical skills | Pediatric residents performing PALS scenarios | 3-point scale | Interrater reliability (IRR); r = 0.82 |
Score variance attributable to rater: 1.4% | ||||
Lockyer et al. [115] | Clinical skills | Students taking an NRP course | 3-point scale | Internal consistency; α = 0.70 |
van der Heide et al. [116] | Clinical skills | Resident adherence to NRP guidelines | Weighted scoring system | IRR; r = 0.77 |
Ishman et al. [117] | Technical skills | Resident performance with pediatric direct laryngoscopy/rigid bronchoscopy | 5-point scale (two checklists: surgical checklist and global assessment of surgical performance) | IRR; intraclass coefficient = 0.49 for surgical checklist and = 0.70 for global assessment |
Internal consistency; α = 0.97 | ||||
Brett-Fleegler et al. [118] | Hybrid (clinical/leadership skills) | Pediatric residents | Dichotomous (Yes/No) | IRR; intraclass coefficient = 0.8 |
Grant et al. [119] | Leadership (2 subscales: leadership/communication skills and knowledge and clinical skills) | Pediatric residents leading PALS-based resuscitations | 4-point scale | Internal consistency; α = 0.82 |
Generalizability coefficient = 0.76 (acceptable for testing purposes) | ||||
Reid et al. [120] | Team assessment | Pediatric “experts” vs pediatric residents | 3-point scale | IRR; intraclass coefficient = 0.81 |
Good discrimination: “expert” 84% vs residents 66% | ||||
Anderson et al. [121] | Team assessment | Neonatal resuscitation providers | 5-point scale | Internal consistency; α = 0.83–0.92 |
Team Assessment | Multiple pediatric providers | 5-point scale | IRR; intraclass coefficient = 0.97–0.98 | |
Internal consistency; α = 0.97 | ||||
Sigalet et al. [124] | Team Assessment | Undergraduate students from medicine, nursing, and respiratory therapy | 5-point scale with defined anchors | IRR; intraclass coefficient = 0.87–0.91 |
Internal consistency; α = 0.90 |
The “Art” of Pediatric Simulation
There is no area of simulation where artful creativity is better expressed than in the area of pediatrics. The unique elements of caring for pediatric patients and their families present unique opportunities to apply simulation in very novel ways. The following section reviews some of the very unique and novel ways that simulation has been used in pediatrics. We also present innovative collaborations and networks that have been developed for the provision and assessment of simulation-based education. Finally, we present some of the unique challenges and barriers to providing pediatric simulation education.
Barriers and Challenges
Pediatric simulation education programs face a barrier unique to other areas of simulation: the diverse range of sizes of pediatric patients. From preterm infants, to toddlers, to school-aged children, and finally to adolescents, the significant range of patient sizes creates a potential problem for simulation educators in terms of maintaining contextual realism. This problem exists across the whole spectrum of simulation, from standardized patients through to high-fidelity patient mannequins. Many programs have successfully integrated adolescent standardized patients into their training and evaluation (OSCEs), while fewer have incorporated school-aged children. The younger the child, the more difficult it is for them to be truly standardized making it impossible to use young children and toddlers as standardized patients. In terms of mannequin-based simulation, and in particular high-fidelity patient mannequins, mannequin vendors have responded to the problem of a diverse patient population by creating different versions of mannequins to represent different sizes of patients required within pediatric simulation. Although a detailed discussion of the pediatric mannequins can be formed in Chapter 15, Table 35.4 provides a summary of the main mannequin vendors with their high-fidelity pediatric versions.
Table 35.4
Summary of pediatric simulation mannequins
Vendor | High-fidelity mannequin | Age ranges represented |
---|---|---|
Laerdal | SimNewB™ | Premature infant |
SimBaby™ | Infants (<1 year) | |
SimJunior™ | 5–9 year olds | |
SimMan® | Adult (surrogate for adolescent) | |
CAE/METI | BabySIM® | Infants/young toddler (<2 years) |
PediaSIM® | 5–9 year olds | |
iStan®/HPS®/ECS® | Adult (surrogate for adolescent) | |
Gaumard | Premie HAL® | Premature infant |
Newborn HAL® | Infants (<1 year) | |
Pediatric HAL® One Year | 1–3 year olds | |
Pediatric HAL® Five Year | 5–9 year olds | |
HAL®/Susie® | Adult (surrogate for adolescent) |
One of the most significant barriers to new and growing pediatric simulation programs is the initial cost of purchasing enough simulators to span the age ranges desired. Most adult-based simulation programs will often choose one model of simulator that they find useful and use that in their center. The advantage of this is that facilitators and educators only have to learn one mannequin platform. In pediatrics, a program that wants to cover all available ages would have be proficient with at least two mannequin platforms, which can be very stressful for program staff, facilitators, and educators.

Full access? Get Clinical Tree
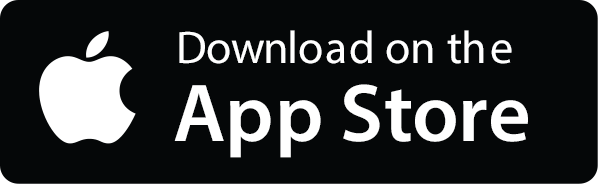
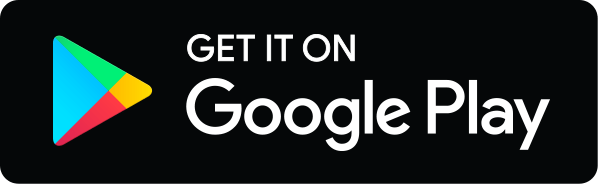