Fig. 33.1
Physical temporal bone simulator with electronic interface (Stryker, Kalamazoo, MI) providing metrics and image guidance
Kuppersmith et al. were among the first proponents of the power of virtual reality simulators for otology training by otolaryngology trainees. They specifically discussed the development of an anatomically accurate virtual 3D environment whereby trainees could practice surgical skills with force, vibratory, and auditory feedback [9]. Several other groups have developed virtual reality (VR) temporal bone dissection simulators including Agus et al., the Ohio State VR simulator, the Stanford VR simulator, the VOXEL-MAN TempoSurg VR simulator (Fig. 33.2), the Visible Ear Surgery VR simulator, the CSIRO/Melbourne VR simulator, and the Karl Storz Surgical Cockpit ENT VR simulator [10–15].
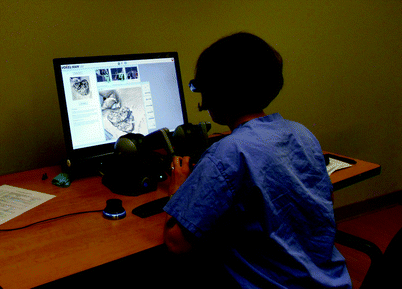
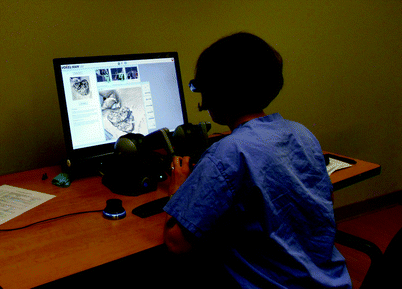
Fig. 33.2
Virtual reality temporal bone simulator (VOXEL-Man, Hamburg, Germany), providing auditory information, haptic interface, and metrics
Most of the VR simulators incorporate haptic and auditory feedback. Kerwin et al. reported on increasing the realism of VR temporal bone drilling by rendering fluid dynamics including bleeding effects, meniscus rendering, and refraction [16]. The neurosurgical literature also reports a VR simulator designed for transpetrous extended approaches [17]. Unfortunately, many of these simulators are prototypes and not commercially available for purchase.
Besides the challenges of development and production, there is also the burden upon the developers to undertake the great monetary and time investment of intensive validation. Wiet et al. have recently published a manuscript detailing the validation of the Ohio State VR temporal bone simulator at eight different institutions across the USA; practice on their VR simulator was found to be equivalent to practice on cadaveric temporal bones [18].
Similar to physical models with electronic interfaces, VR simulators allow unlimited opportunities for practice including some components of competency assessment and feedback without requiring the presence of an expert surgeon during each dissection. Time for dissection, identification and violation of key structures, and efficiency of movement can all be measured. Additionally, comparing VR temporal bone simulators to both cadaveric and physical models, there is not a physical limit as to the number of temporal bones available for dissection, mistakes can be “reversed,” and the trainee does not have to discard a temporal bone to start over. Moreover, by comparing the “pixels” or areas of bone removed by a trainee to compiled data from expert dissections, one can score adequacy of dissection [12, 18]. Incorporation of these competency measurements into the simulator is an active area of research. As computer hardware and software technology advances, so to will the realism of current VR platforms. Some have proposed that during the early stages of training, three-dimensional models and virtual reality temporal bone simulators are more than adequate to meet trainees’ needs…[and] the holistic benefits of cadaveric dissection would be better utilized once trainees have reached the “autonomous” stage [7].
Although there is a lot of interest and great potential for the use of technology-augmented simulators, several low technology otologic simulators also exist. Mathews et al. describe an incus and stapes footplate simulator for stapes prosthesis placement comprised of a disposable drinking cup, toothpicks, and a tongue depressor [19]. Similarly, Owa et al. describe another low-cost simulator for stapes prosthesis placement made out of ward-based materials [20].
For the novice otolaryngologist, even myringotomy tube insertion, which typically includes the use of a microscope, is challenging and can be simulated. Several otolaryngologists have constructed simple models designed to allow beginners to practice hand-eye or hand-eye-microscope coordination (Fig. 33.3). Volsky et al. describe a physical anatomic model of a head with tympanic membrane cartridge components which fit inside the ear canal, allowing for repeated attempts at performing myringotomy and pressure equalization tube placement. Construct validity has been demonstrated for this simulator, as it has been shown to discriminate novice from non-novice users [21]. There are also virtual reality simulators for performing a myringotomy [22, 23].
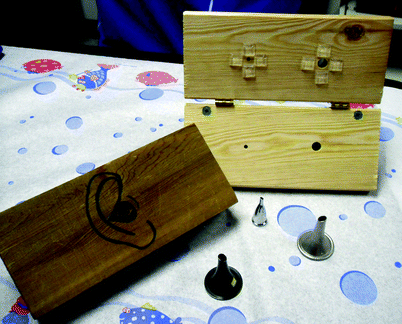
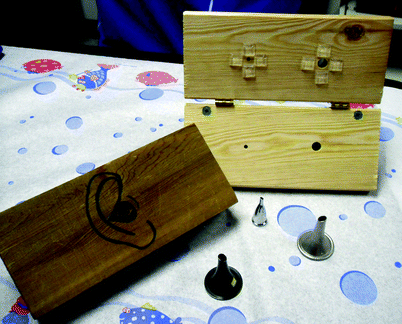
Fig. 33.3
Constructed otology simulator, for practicing myringotomy tube insertion and office-based procedures. A piece of plastic inserted between the wooden blocks functions as a tympanic membrane. Foreign bodies, or lotion to represent otorrhea, can be placed in the external ear canal. The narrower aperture represents a stenotic ear canal. Simulator developed by Steve Handler, MD
Sinus Simulators
Rhinologic and sinus simulators have some characteristics which are similar to otology simulators, in that the underlying anatomic framework which the simulator replicates is bone, but there are also important differences. In contrast to the rigidity of much of the temporal bone, many of the bones in the nose and sinuses are thin and are manipulated or displaced (i.e., “outfractured”) during surgical procedures, so it would be optimal to represent them as non-static structures. The delicate soft tissue interface within rhinosinus anatomy is also complex. Sinus simulator development has parallels with otology simulator development, in that both 3-dimensional and virtual models have been built.
The ES3 simulator, a virtual sinus simulator with an anatomic, haptic interface, has been extensively studied by Weghorst, Rudman, Edmond, Fried, and several coauthors, and is one of the most comprehensively validated extant simulators [1]. Learning exercises with this simulator include both nonanatomic skills (e.g., using a surgical orientation, but manipulating abstract shapes) and specific anatomic exercises, such as middle turbinate medialization and uncinectomy [1]. Additional virtual simulators include the Nasal Endoscopy Simulator [24, 25], the Voxel Man SinuSurg [26], and a simulator described by Caversaccio et al. [27]. Most of the virtual sinus simulators include haptic feedback and an anatomic interface; and most are in the prototype phase. Caversaccio et al. found no improvement in actual procedures on patients [27]. Edmond suggested and Fried et al. documented improved operative technique and performance during actual surgery related to training with the ES3 simulator [1, 28].
Several physical rhinosinus models have been developed [29–31]. Leung et al. describe both a physical and cadaveric model which can be used for both nonanatomic and anatomic skill training [32]. Malekzadeh et al. have developed and validated a low cost, low technology gelatin model which is semi-anatomic (Fig. 33.4) [33].
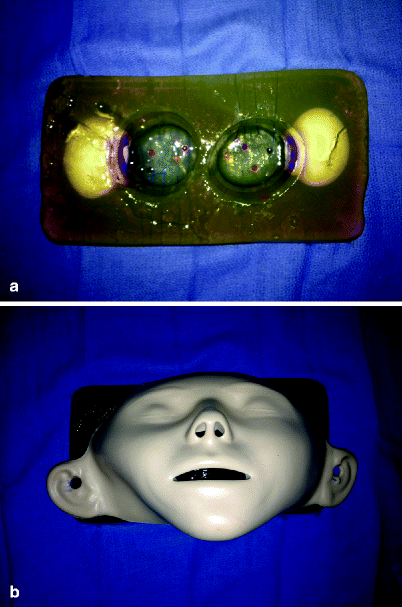
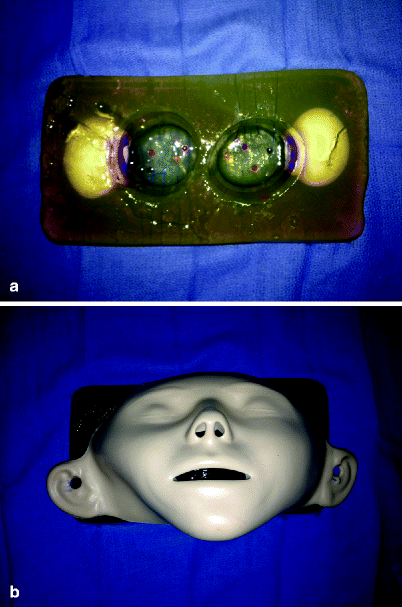
Fig. 33.4
Sinus surgery model constructed from gelatin and other items. The gelatin model (a) is placed behind the mannequin face (b). Entering the maxillary sinuses releases the contents of the raw eggs, which can be suctioned. Simulator developed by, and photos courtesy of, Sonya Malekzadeh, MD
An epistaxis simulator can be constructed from material generally available in simulation centers and is useful for novices who are learning how to utilize a headlight as well as how to construct and apply nasal packing (Fig. 33.5) [34]. Thin tubing inserted through the back of the mannequin’s head and into its nose allows simulated blood to flow until the learner inserts packing materials or an epistaxis control device to staunch the bleeding. Both anterior and posterior nasal packs can be inserted.
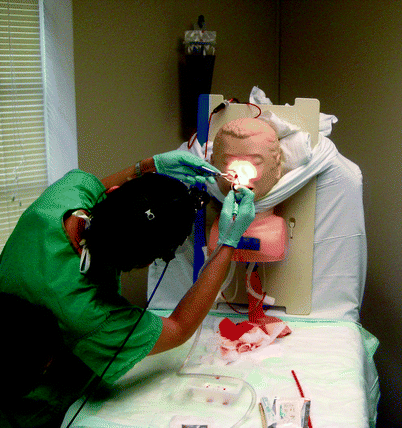
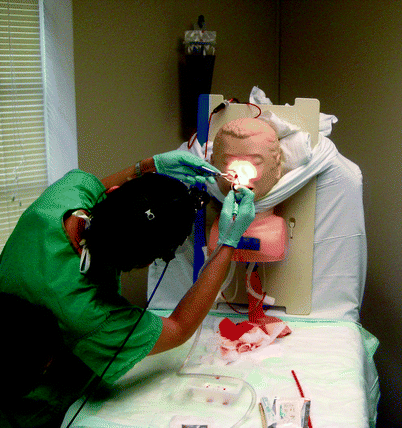
Fig. 33.5
Epistaxis model. Faculty controls the flow of “blood” through the tubing inserting from the back of the head into the nasal cavity
Airway Simulators
Although all surgical procedures require skill and involve risk for patients, airway work exemplifies the challenges which make simulation valuable to the otolaryngologist, the anesthesiologist, and their patients. For example, a typical resident would be expected to manage between 0.2 and 3.9 cases of foreign body aspiration per year, depending on their training location [35]. Traditionally, simulation used to learn these techniques occurred in an animal laboratory. Currently the management of aspirated foreign bodies can also be practiced by inserting a foreign body into a variety of full body mannequins, and this exercise can be repeated as many times as desired (Fig. 33.6) [36].
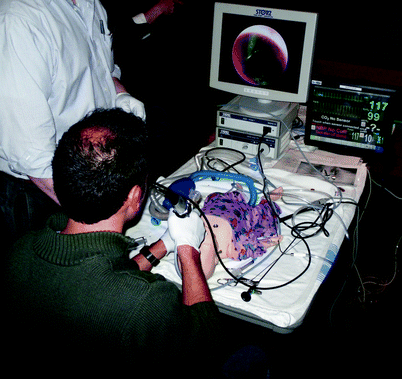
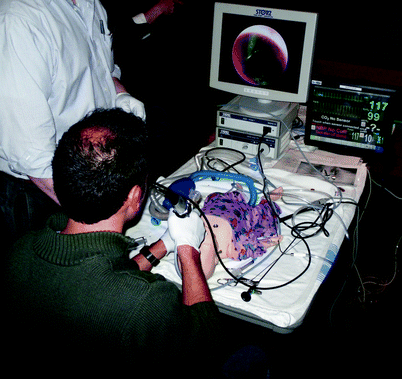
Fig. 33.6
Resident removing an “aspirated” foreign body from a high-technology infant simulator (SimBaby, Laerdal, Wappinger Falls, NY) in an “ad hoc” simulation setting during an Airway Foreign Body Course. Note the image from the bronchoscope on the endoscopy monitor, and the vital signs monitor displaying physiologic data; the pulse oximeter also provides audible information. If the mannequin is not adequately ventilated, the pulse oximetry reading will decrease; if the endoscopist does not adequately coordinate with the “anesthesiologist,” the mannequin will demonstrate laryngospasm
In general, the mannequins’ airway characteristics are not identical to those of humans, but they are sufficiently representative, particularly for novices who are also learning how to manipulate the appropriate instruments. When a physical model with representative laryngeal, tracheal and endobronchial anatomy is used (whether low or high technology), airway anatomy can be learned using actual equipment, such as laryngoscopes, rigid telescopes or fiberoptic bronchoscopes. Howells et al found that students who had trained on a simulator were able to develop intubation skills in actual patients more quickly and safely than those without simulator training [37]. Rowe and Cohen demonstrated improved skills during bronchoscopy on real patients after residents trained on a VR bronchoscopy simulator [38]. Jabbour et al. are developing fabricated organosilicate airway complexes which provide additional anatomic fidelity [39].
After the learner manages basic instrument manipulation skills, the complexity of the simulation can be increased to represent a more comprehensive experience, including reviewing radiographs prior to performing the procedure, planning and coordinating with the anesthesiologist, etc. If a high-technology mannequin is used, additional conditions and characteristics can be modeled, such as oxygen desaturation, asymmetric chest wall expansion, and laryngospasm.
Burns et al. have adapted a model which takes advantage of the small caliber vascular network present in the chorioallantoic membrane of chicken eggs, providing a responsive biologic platform for laser and microlaryngeal procedures [40, 41]. Several models have been developed for simulating tonsillectomy; most attempt to replicate the challenge of accessing distant structures (e.g., the tonsils in the oropharynx) through a relatively narrow aperture in a very representative fashion [42, 43].
Head and Neck Simulators
Recent advances in Head and Neck Surgery with Transoral Robotic Surgery has created a new need for training both new and experienced otolaryngologists in these novel techniques. This has spawned the creation of robotic skill training simulators including da Vinci Skills Simulator (Intuitive Surgical, Sunnyvale, CA); and Robotic Surgical Simulator (Simulated Surgical Systems, Williamsville, NY) for robot-assisted surgical procedures. The da Vinci Skills Simulator is a training device that integrates into an existing surgeon’s console and includes abstract and anatomic virtual reality exercises that can be projected to the trainee through the binocular optics (Fig. 33.7). A simulator can also be created with the robotic arms and the surgeon’s console. Physical task training exercises can also be performed using the actual robotic instrumentation. The virtual reality exercises have all the aforementioned advantages of virtual reality simulators, including the ability to repeat exercises, the ability to practice skills without the need of an attendant experienced surgeon, and the ability to compile data regarding competency assessment, such as time to completion of tasks, instrument collisions, and movement efficiency, with real-time feedback.
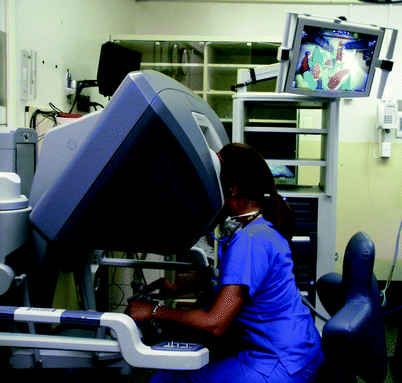
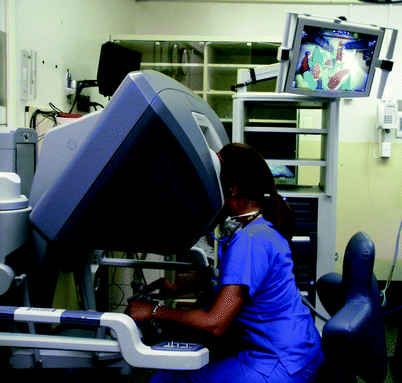
Fig. 33.7
Learner at a robotic console (da Vinci, Intuitive, Sunnyvale, CA), demonstrating practice of robotic manipulation skills with nonanatomic tasks
Despite these recent advances, not all head and neck simulators require expensive or complex technology. Simulators valuable in training head and neck techniques of local flaps include the traditional use of pig’s feet and photographs. Local rotation and advancement flaps can be marked out and planned on pictures of patients with actual lesions in various anatomic regions. Trainees can then go a step further and actually practice executing these local flaps using pigs’ feet, surgical instrumentation, and suturing techniques (Fig. 33.8) [44].
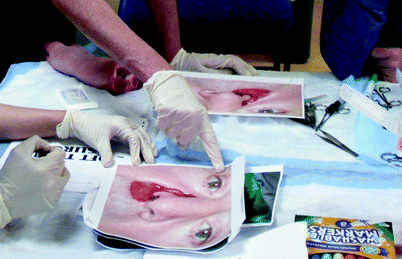
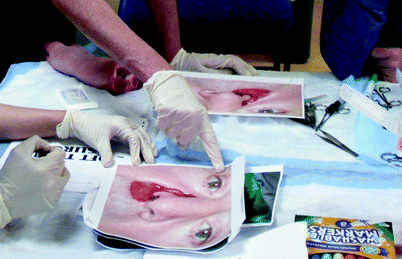
Fig. 33.8
Participants design local rotation and advancement flaps on photographs of lesions and then execute the flaps on biologic tissue (pigs’ feet)
Microvascular anastomosis is another skill set with an available simulator. Biomet (Warsaw, Indiana) has a kit which is a physical task trainer with a delicate synthetic vessel that must be anastomosed with appropriate suture (Fig. 33.9). Following the completion of the anastomosis, the trainee can inject simulated blood through the vessel to check for leaks. Trainees ideally should wear surgical loupes or use surgical microscopes to increase the realism of the simulation.
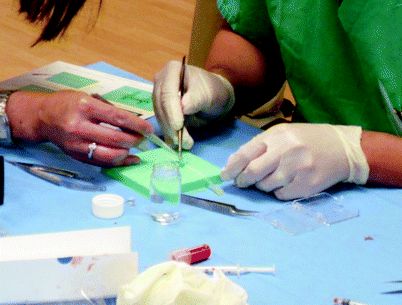
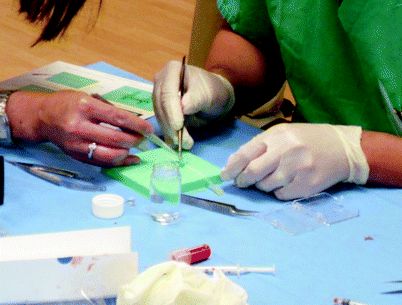
Fig. 33.9
Task trainer for practicing microvascular anastomosis (Biomet, Warsaw, IN)
Plating courses sponsored by organizations such as the AO Foundation (Davos, Switzerland) or plating manufacturers such as Synthes (West Chester, PA) or Stryker (Kalamazoo, MI) can provide hands-on training with physical task trainers in which craniofacial plates can be affixed to simulated skulls to practice treating various craniofacial fractures. This allows the trainees to become familiar with not only the plating technology and instrumentation but also the techniques of facial fracture repair prior to performing on real patients.
Cricothyroidotomy simulators are abundant and varied in their characteristics. They range from full body, high-tech mannequins with the capability of simulating a “can’t ventilate, can’t intubate” scenario requiring cricothyroidotomy or tracheotomy to physical task trainers which can be constructed more economically. Liu et al. have created a virtual simulator with haptic feedback [45] which employs a Phantom haptic interface device (SensAble Technologies, Wilmington, MA) and can simulate cervical landmark identification, incision with a scalpel blade with bleeding and resistance as the scalpel goes through skin, and the resistance of inserting an airway into the trachea. Physical task trainers can be constructed from mannequins (without using the high-fidelity capabilities) [46–48], corrugated tubing [48], cadaveric tissue, a porcine airway model [49], sheep trachea model [50], and converted/recycled mannequin heads [51] to name a few. Some would argue that there is a role for high-fidelity mannequins that these low-fidelity options are not able to fulfill.
John et al. examined the role of stress by comparing the time to perform a cricothyroidotomy on a physical task trainer in a classroom setting to a “medium-fidelity” simulator incorporated in a “can’t intubate, can’t ventilate” scenario including pharyngeal edema, laryngospasm, and restricted neck extension as well as a vital signs display in a simulated operating room setting [47]. Seventy-seven percent of tested anesthesiologists in this randomized crossover study took longer with the medium-fidelity simulator as compared to the physical task trainer suggesting that stress and time pressures in real-life scenarios may affect the performance of cricothyroidotomy. From a strictly skills acquisition perspective, Friedman et al. suggest in their study that a simple inexpensive model constructed from corrugated tubing achieved the same effect on objectively rated skill acquisition as did an expensive high-fidelity simulator [48].
Interpersonal and Professional Clinical Skills Simulation
Besides simulating surgical skills, simulations can be designed to help learners acquire or hone communication skills, including obtaining informed consent, and delivering “bad news” (which includes any sort of information which is unpleasant or uncomfortable for the person receiving the information). The most advanced of these simulations employ “standardized patients,” who are professionals trained not only to interact with the learner but also to evaluate and provide feedback to the learner. Less rigorous methods to simulate a “delivering bad news” situation include using faculty, or even other learners, to role-play as a reluctant patient, an anxious family member, or even a difficult faculty member who must be awakened in the middle of the night with unpleasant information [52].

Full access? Get Clinical Tree
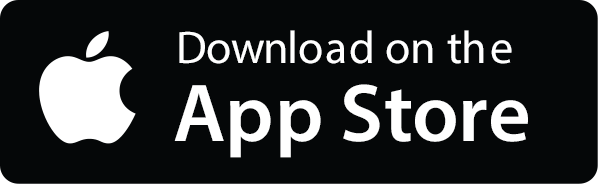
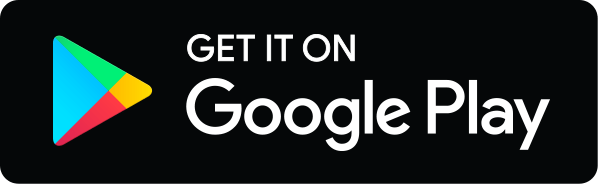