Chapter 11 Shock – an overview
DEFINITION
Shock is a clinical state, with characteristic symptoms and signs, which occurs when an imbalance between oxygen supply and demand results in the development of tissue hypoxia. Although changes in systemic perfusion are always present in shock, oxygen delivery to the tissues is not invariably reduced, and indeed may be increased in shock due to severe sepsis.1
CLASSIFICATION
Physiologically, tissue hypoxia may be considered as hypoxic (low PaO2), anaemic (low haemoglobin level or increased levels of carboxy- or met-haemoglobin), stagnant (low cardiac output) or histotoxic (e.g. cyanide poisoning). Clinically, it is more common to subdivide shock into cardiogenic, obstructive, hypovolaemic or septic. Infrequently it may be neurogenic or anaphylactic in origin (Table 11.1).
Table 11.1 Classification of shock
Physiological | Clinical |
---|---|
Hypoxic | Cardiogenic |
Anaemic | Obstructive |
Stagnant | Hypovolaemic |
Histotoxic | Septic |
Neurogenic | |
Anaphylactic |
Hypovolaemic shock is usually the result of uncontrolled haemorrhage, but may be due to excessive fluid loss from the gastrointestinal and urinary tracts, and even the skin in severe burns. Any type of infection – bacterial, fungal or viral – can be complicated by the development of shock. The clinical findings, perhaps with the exception of the cutaneous manifestations of meningococcal disease, are not specific to the type of organism involved, and it is not generally possible to determine the nature of the infecting organism from clinical examination alone.2
PATHOPHYSIOLOGY
During the initial stages of hypovolaemic and cardiogenic shock, as oxygen delivery begins to fall, the tissues are able to maintain their oxygen uptake (VO2) at a normal level (170 ml/min per m2) by extracting more oxygen from each unit of blood (supply-independent VO2). However, once oxygen delivery falls below a critical value of 330 ml/min per m2, this compensatory mechanism is insufficient and oxygen uptake begins to decline (supply-dependent VO2). This phase is associated with the accumulation of an ‘oxygen debt’, and its severity may be gauged by the degree to which blood lactate is elevated (Figure 11.1).
In septic shock, microbial components or their toxins are recognised by soluble cell-bound receptors, e.g. the lipopolysaccharide of Gram-negative bacteria binds to CD14 and Toll-like receptor 4 (TLR4), whilst the peptidoglycan of Gram-positive bacteria binds to TLR2. This process stimulates the release of proinflammatory (tumor necrosis factor-α (TNF-α), interleukin-1 (IL-1), IL-6) and anti-inflammatory (IL-10, IL-1ra, TNF receptors) cytokines, generation of complement, activation of coagulation and platelet aggregation. There is also increased synthesis of arachidonic acid metabolites, reactive oxygen species and nitric oxide.3 The combined effect of these changes is to produce vasodilatation, increased cardiac output despite impaired contractility and reduced intravascular volume secondary to increased capillary permeability.
DO2 in septic shock is supranormal, mainly as a result of the elevated cardiac output. VO2 is also raised, due to an increase in tissue metabolic activity. At levels of DO2 above the normal critical threshold, although VO2 is increased, it appears to be inadequate and a lactic acidosis develops. Supply dependency is thus observed over a wider range of oxygen delivery values than usual. This may be explained by abnormalities in perfusion at a microcirculatory level, resulting in locally reduced DO2 despite a supranormal global value. Alternatively, sepsis-induced mitochondrial dysfunction may prevent oxygen utilisation at a cellular level.
CLINICAL PRESENTATION
In contrast septic shock is usually hyperdynamic, unless the patient is also significantly hypovolaemic. By definition, the patient must have a proven source of infection, be hypotensive (or requiring vasopressors to maintain a systolic blood pressure of 90 mmHg), exhibit two or more signs of systemic inflammation (tachycardia, tachypnoea, hypo-/hyperthermia, leukocytosis/leukopenia) and have dysfunction of at least one end-organ.4 As with other forms of shock, the patient is often confused, tachycardic, tachypnoeic and oliguric. However, in contrast to hypovolaemic, obstructive and cardiogenic shock, peripheral pulses are bounding and the extremities are warm to touch. In meningococcal septicaemia a characteristic purpuric rash may be visible.
INVESTIGATIONS
LABORATORY, RADIOLOGICAL AND NON-INVASIVE CARDIAC INVESTIGATIONS
In cardiogenic and obstructive shock echocardiography is invaluable, since it provides an objective measure of ventricular function, can identify and quantify abnormalities of regional wall motion and valvular function, and excludes cardiac tamponade or massive pulmonary embolus. Where the history and preliminary findings raise the suspicion of pulmonary embolus, alternative confirmatory tests include spiral computed tomography (CT), which is readily available in most hospitals, and pulmonary angiography.5
Hypovolaemic shock, due to concealed haemorrhage, may require further invasive and radiological investigations such as diagnostic peritoneal lavage, abdominal ultrasound, or CT scanning. When hypovolaemia is due to excessive gastrointestinal or renal losses, electrolyte disturbances can be severe, and urea and creatinine are often markedly elevated. Haemoconcentration may also be noted. Further investigations will depend upon the likely pathology, but may include supine and erect abdominal X-rays in bowel obstruction, abdominal ultrasound in acute cholecystitis and serum amylase and abdominal CT scan in pancreatitis.
Septic shock can lead to a rise or fall in the white cell count, the latter being associated with a particularly poor prognosis. Best practice dictates that blood and other relevant cultures are taken prior to initiating antibiotic therapy wherever possible, and in certain cases measurement of C-reactive protein and procalcitonin may be of value.6,7 Disseminated intravascular coagulation, diagnosed by the combination of prolonged clotting, thrombocytopenia and reduced fibrinogen, is often present. When measured, antithrombin III, protein C and protein S levels are commonly low.
Blood lactate is elevated in all forms of shock, and indicates the presence of tissue hypoxia. The degree to which it is elevated corresponds to the severity of the shock, and it is frequently used as a guide to the effectiveness of therapeutic interventions.8 Furthermore, lactate, base excess or a combination of the two can be used to predict outcome in patients admitted to the intensive care unit (ICU).9
INVASIVE HAEMODYNAMIC MEASUREMENTS
Whilst the therapeutic efficacy of the pulmonary artery catheter (PAC) remains controversial,10 the measurements obtained from it are undoubtedly extremely useful in differentiating between the four major types of shock (Table 11.2).
Pulmonary artery catheterisation is rarely required to aid in the diagnosis of uncomplicated hypovolaemic shock. The clinical picture and presence of a low CVP are usually sufficient. However, the additional information obtained from a PAC may be invaluable in differentiating cardiogenic shock due to acute myocardial infarction (pulmonary artery occlusion pressure (PAOP) elevated, CVP normal or elevated, pulmonary artery pressure (PAP) normal), from pulmonary embolus (PAOP normal, CVP and PAP elevated) and cardiac tamponade (PAOP and CVP identical and elevated). It may also be helpful in cases of septic shock that are not clinically hyperdynamic, particularly in relation to optimising fluid, inotrope and vasopressor therapy. Alternatively, optimisation of cardiac preload and index in all forms of shock may be guided by measurement of stroke volume variation and cardiac index using lithium dilution (LiDCO) or intrathoracic blood volume index, extravascular lung water, stroke volume variation and cardiac index by transpulmonary thermodilution (PiCCO).11–13
PRINCIPLES OF MANAGEMENT
GENERAL MEASURES
FLUID THERAPY
Optimising cardiac preload and restoring circulating volume are fundamental aspects of correcting tissue hypoxia in patients with shock. In cases of hypovolaemic and septic shock several litres of fluid are usually needed to achieve this, but occasionally even some patients with cardiogenic shock may benefit from a judicious volume challenge. In patients with severe sepsis, aggressive volume replacement within 6 hours of presentation in conjunction with targeting a central venous oxygen saturation > 70% (ScvO2 > 70) and haemoglobin level > 10 g/dl can reduce hospital mortality by up to 16%.14
CVP is often used as a surrogate for myocardial preload in uncomplicated hypovolaemic shock. However, in patients with ischaemic heart disease PAOP is usually preferred. Intrathoracic blood volume, an alternative measure of cardiac preload, offers theoretical advantages over both CVP and PAOP, particularly in mechanically ventilated patients, and is obtained using either double (COLD) or single (PiCCO) indicator dilution.12,13 More recently, stroke volume variation (SVV) with respiration has been shown to be the best predictor of volume responsiveness, and has the advantage of being relatively easy to obtain through analysis of the arterial waveform trace.15 When SVV is 9.5% or greater a 100 ml volume load will increase stroke volume by at least 5%.16 It is less useful in patients with atrial fibrillation or frequent ventricular ectopics, where wide fluctuations in baseline stroke volume are present.
Logically, the fluid used to correct any deficit should reflect the type of fluid lost, and patients who have significant bleeding will clearly require blood. In intensive care patients without acute coronary syndromes, it appears safe to aim for a haemoglobin level of 7–9 g/dl once the acute resuscitation phase has passed. Indeed, targeting this level is associated with a lower mortality than 10–12 g/dl.17 At present, the use of blood substitutes such as diasprin cross-linked haemoglobin is not recommended in haemorrhagic shock, as they are linked to a higher death rate.18
Given that blood is usually supplied in the form of red cell concentrates, the clinician must decide whether to combine it with a crystalloid, human albumin or a synthetic colloid in order to restore circulating volume. The same dilemma, over whether to use a crystalloid or a colloid during resuscitation, also arises in patients with septic shock. One property in favour of colloids is that they restore circulating volume more efficiently than crystalloids, since approximately 1.5 times as much crystalloid must be infused to achieve the same haemodynamic end-point.19 It is therefore common to begin resuscitation with a colloid to restore intravascular volume, and to continue with crystalloid to correct interstitial and intracellular losses. A recent, large placebo-controlled trial of normal saline versus 4% albumin for resuscitation in ICU may challenge this practice, however, since no differences in mortality or morbidity could be demonstrated between the groups, and crystalloids are undoubtedly much cheaper to use.19
In addition, several papers have raised concerns regarding the safety of colloids. A systematic review of randomised studies comparing the use of crystalloids and colloids in the resuscitation of critically ill patients concluded that the use of colloid was associated with a 4% increase in mortality.20 The specific issue of renal failure was highlighted in a randomised controlled study comparing the use of the synthetic colloid hydroxyethyl starch (MMW-HES 200 kDa, 0.6–0.66 substitution) with 3% gelatine in patients with sepsis and septic shock. In this study the incidence of renal failure was significantly higher in the HES group.21 The use of high-molecular-weight hydroxyethylstarch (HMW-HES 450 kDa, 0.5 substitution) has also been associated with abnormal clotting and increased bleeding.22
A number of recent publications have focused on the use of hypertonic crystalloids, such as 7.5% sodium chloride alone or in conjunction with dextran 70, for initial resuscitation. While most of the large studies have failed to show a clear survival benefit in the general trauma population, hypertonic solutions may be useful in certain subgroups, such as those with severe head injuries, in whom the administration of large volumes of isotonic crystalloid may worsen cerebral oedema.23,24 Most studies also show that the use of hypertonic solutions is associated with a reduction in fluid and blood transfusion requirements.25

Full access? Get Clinical Tree
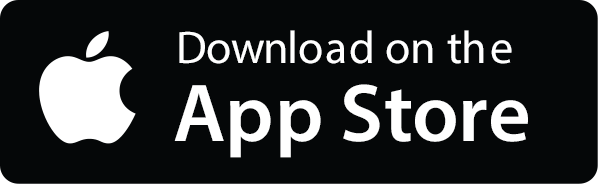
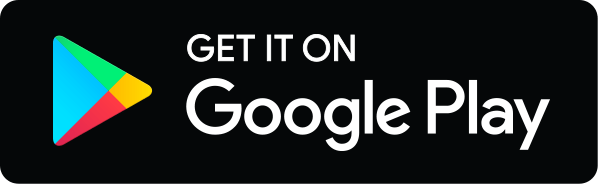