Shock is a profound state of circulatory failure that leads to a reduction in delivery and impaired utilization of oxygen and other nutrients at the end-organ level. At first, these changes cause reversible injury and, if prolonged, lead to irreversible cellular damage.1,2 Shock is a common syndrome encountered in the intensive care unit (ICU) and is associated with a high mortality rate.1-3 Consequently, early recognition, prompt exploration of its etiology, and effective management of shock can greatly influence clinical outcomes.
Fundamentally, shock is a state of circulatory collapse relating to an aberration in preload (hypovolemic shock), pump function (cardiogenic and obstructive shock), or afterload (distributive shock). Early signs of shock include alterations in vital signs (tachycardia, tachypnea, and hypotension) and clinical evidence of end-organ hypoperfusion. Specifically, end-organ hypoperfusion can manifest as an acute alteration in mental status, a decrease in capillary refill, and a decrease in urine output (< 0.5 mL per kg of body weight per hour). Laboratory abnormalities may include alterations in lactate levels and mixed venous oxygen saturations.
The initial approach to a patient in shock involves an assessment of the cause as well as simultaneous management of the shock (Tables 4-1 and 4-2). As resuscitative efforts are initiated, a close examination of the available history, clinical examination, laboratory findings, and radiographs is warranted. As noted above, shock is characterized by a hypoperfused state, manifested by low blood pressure (BP). Both cardiac output (CO) and systemic vascular resistance (SVR) contribute to BP, as demonstrated by the equation BP = CO × SVR. The various types of shock are characterized by distinct alterations in either or both parameters. A thorough understanding of this relationship is advantageous when managing a patient in shock.
Type of Shock | Description | Prevalence |
---|---|---|
Hypovolemic shock | Hemorrhage (trauma, variceal bleed, coagulopathy, post-partum hemorrhage), severe diarrhea or vomiting | 16% |
Cardiogenic shock | Myocardial ischemia, acute decompensated heart failure, acute valve rupture | 16% |
Obstructive shock | Cardiac tamponade, tension pneumothorax, massive pulmonary embolism | 2% |
Distributive shock | Sepsis, anaphylaxis, acute spinal cord transection, acute adrenal insufficiency | 66% |
Shock type | CO/CI | PCWP | SVR | SvO2 | Comments |
---|---|---|---|---|---|
Hypovolemic | Low | Low | Normal/high | Low | |
Cardiogenic | Low | High | High | Low | |
Acute MR | Low forward CO | Giant V waves on PAOP tracing | |||
Acute VSD | Low forward CO | Step up in oxygen saturation between RA and RV | |||
RV failure | Normal/low | Elevated RA and RV pressures with normal PAOP | |||
Obstructive | Low | High | High | Low | |
Massive PE | PAD > PCWP by > 5 mmHg, increase in PVR | ||||
Tamponade | Equalization of pressures (RA, PA diastolic, and wedge) within 5 mmHg | ||||
Distributive | Normal/ high | Low/normal | Low | High | Decreased oxygen extraction by peripheral tissues |
Late Septic | Normal/low | Can be high |
Patients with decreased cardiac output and increased systemic vascular resistance will often have cool extremities, delayed capillary refill, and a narrow pulse pressure, often referred to as cold shock. The underlying etiology in this situation is either hypovolemic, cardiogenic, or obstructive. Hypovolemic shock is characterized by a low intravascular volume, which can be seen via low or flat jugular venous pressure (JVP), and low cardiac filling pressures. Also, an etiology for volume loss usually is apparent. Cardiogenic shock, on the other hand, is often associated with elevated intravascular volume, manifesting as an elevated JVP, elevated cardiac filling pressures, extremity edema, or crackles on lung exam. Myocardial ischemia on an electrocardiogram (ECG), elevated cardiac biomarkers, or evidence of structural impairment on echocardiography also may be seen. Obstructive shock is often more difficult to recognize and requires a high index of suspicion. Adjunct investigations such as an echocardiogram, computed tomography (CT) scan, or a chest x-ray are often needed to diagnose obstructive shock. Conversely, distributive shock (septic or otherwise) is characterized by a normal to high cardiac output state with a low SVR, or impaired oxygen extraction in the periphery. However, it is important to recognize that these classical descriptors of shock are not mutually exclusive and, can in fact, coexist.
Hypovolemic shock is characterized by a low preload. The decreased pressure in the capacitance system creates a weaker gradient to the right atrium, clinically characterized by low venous pressures. The initial compensatory mechanism activates a sympathetic response that causes peripheral vasoconstriction in order to maintain adequate systemic arterial pressures and thereby perfuse distal tissues. A shift in the left ventricular end systolic pressure-volume relationship also occurs as a part of this response. The left ventricle (LV) tries to generate a higher pressure for a lower left ventricular end diastolic volume (positive inotropy) and there is also an increase in heart rate (positive chronotropy). These changes augment the cardiac output. Initially, the body is able to compensate and maintain perfusion to vital organs such as the brain and the heart. However, if the initial insult is not reversed and there is continued volume loss beyond 40% of the total body volume,3 there is a left shift in the diastolic pressure-volume relationship with increased diastolic stiffening of the LV. Poor filling of the LV ensues, resulting in a lower stroke volume, which further decreases preload. Ultimately, diminished end organ tissue perfusion activates the release of systemic inflammatory mediators that can directly suppress the myocardium and perpetuate refractory shock.
Treatment of hypovolemic shock relies on early identification of and control of the underlying source with adequate fluid resuscitation to maintain cardiac output. There have been numerous studies that have examined what the optimal fluid is for resuscitation in hypovolemic shock. The Saline versus Albumin Fluid Evaluation (SAFE) investigators4 reported no significant difference in outcomes, including 28-day mortality, in patients receiving 4% albumin versus normal saline in medical and surgical ICU patients. The higher cost of albumin and lack of clear benefit have made colloids a second-line agent. Other investigators5,6 have also found no significant difference in outcomes such as 28-day mortality, as well as ICU and hospital length of stay when comparing patients treated with colloids versus crystalloids. Use of hydroxyethyl starch for resuscitation also has been discredited due to increased risk of acute kidney injury, renal replacement therapy, and mortality.7
Resuscitative strategies for hemorrhagic shock are somewhat different from those strategies described above. The underlying premise of resuscitation in this setting is to utilize blood products over crystalloids. In the recently published progmatic, randomized optimal platelet and plasma ratios (PROPRR) study, a 1:1:1 (plasma to platelets to packed red blood cells) transfusion strategy did not show a significant difference in mortality at 24 hours or 30 days when compared to a 1:1:2 ratio. However, the 1:1:1 group achieved better hemostasis and had fewer deaths attributed to exsanguination. In addition, there were no significant differences in safety with respect to the development of acute respiratory distress, venous thromboembolic events, coagulation abnormalities, or transfusion-associated complications.8 The literature also suggests that the use of tranexamic acid leads to favorable outcomes in both civilian9 and military trauma settings10 in the management of hemorrhagic shock. The end point of resuscitation, again, has not been clearly defined but essentially entails achieving hemostasis while maintaining tissue perfusion.
Cardiogenic shock is characterized by inadequate tissue and/or end-organ perfusion due to cardiac dysfunction. It is defined via persistent hypotension (systolic blood pressure < 80 to 90) with a severe reduction in the cardiac index (< 1.8 L/min per m2 without support or < 2 to 2.2 L/min per m2 with support) in the setting of adequate filling pressures (left ventricle end-diastolic pressure > 18 mmHg or right ventricle end-diastolic pressure of 10–15 mmHg). The incidence is estimated at 5% to 8% of ST elevation myocardial infarcts and 2.5% of non–ST elevation myocardial infarcts.11 The most common underlying etiology remains acute myocardial dysfunction with associated left ventricular failure but can also be caused by right ventricular dysfunction, wall rupture, tamponade, or valvular pathology.
Based on data collected from several national registries, both the incidence and mortality from cardiogenic shock seem to be decreasing. Presumably, this decrease is a result of early reperfusion strategies now employed for ischemic heart disease.11 Despite these improvements, increasing age, prior myocardial infarction (MI), oliguria, and physical exam findings suggestive of poor perfusion have been identified as independent predictors of increased mortality.12
From a pathophysiologic perspective, cardiogenic shock as a result of myocardial ischemia has been described as a “downward spiral.” Myocardial perfusion depends on the pressure gradient between the coronary arterial system and the left ventricle, and on the duration of diastole. When stroke volume and cardiac output decrease after an initial ischemic insult, hypotension leads to a further decrease in myocardial perfusion. This decrease in perfusion leads to worsening ischemia and progressive myocardial dysfunction. Ultimately, without intervention, this downward spiral leads to death.13
As noted above, most patients with cardiogenic shock have evidence of significant coronary artery disease, particularly either left main disease or triple vessel disease. This fact is important because compensatory hyperkinesis normally develops in unaffected segments to help maintain cardiac output. The inability to augment CO, because of previous infarction or high-grade coronary stenosis, is an important risk factor for cardiogenic shock and death.14 Consequently, early revascularization strategies have been shown to improve long-term outcomes, as evidenced in the SHOCK trial. More specifically, primary percutaneous coronary intervention (PCI), when available, is preferred as compared to fibrinolytic therapy.15
Although the data supporting early PCI is convincing, the data in support of routine intra-aortic balloon pump (IABP) insertion is less robust. No clear-cut mortality benefit has been seen to date.16 Consequently, the American College of Cardiology/American Heart Association (ACC/AHA) guidelines on ST-elevation myocardial infarction (STEMI) recommended an IABP as a stabilizing measure in patients with acute MI and cardiogenic shock that is not quickly reversed with pharmacologic therapy.17
Percutaneous mechanical circulatory devices are continuous flow pumps designed to augment end-organ perfusion, reduce intracardiac filling pressures, reduce myocardial oxygen consumption, and augment coronary perfusion. As of yet, they have not been shown to improve clinical outcomes but are being used in a variety of situations, including cardiogenic shock, as recommended by the ACC.18
Extracorporeal membrane oxygenation (ECMO) is a cardiopulmonary support device that can be used for respiratory failure and/or shock. With venovenous bypass, ECMO primarily helps in respiratory failure by bypassing the pulmonary circulation and uses an artificial membrane to remove carbon dioxide and provide oxygen. With venoarterial bypass, an extracorporeal pump is used to support systemic circulation. There is a growing amount of data, albeit from observational and case control studies, that ECMO may improve outcomes for those in cardiogenic shock.19,20
Both ventricular septal defects and acute mitral regurgitation can be seen in the days following acute myocardial infarction. Classically, these complications occur in the range of 2 to 7 days after a myocardial infarction. An increased risk is seen in patients with disease involving the left anterior descending artery for ventricular septal defects and the posterior descending artery for acute mitral regurgitation. However, diffuse disease with poor collateral circulation can also be the underlying cause. Prompt action is necessary as clinical deterioration can be sudden. Both of these diagnoses need to be differentiated from rupture of the left ventricular free wall. In fact, ventricular septal rupture can be difficult to distinguish from acute mitral regurgitation on a pulmonary arterial catheter because both can produce dramatic V waves. However, the classic finding is a left-to-right intracardiac shunt, which manifests as an increase in oxygen saturation from the right atrium to the right ventricle (step-up; see Chapter 3, “Hemodynamic Monitoring”). Bedside echocardiography again helps to distinguish between the 2, and surgical repair usually is necessary.15
Obstructive shock can often be classified as either mechanical or secondary to pulmonary vascular diseases. The mechanical etiologies often present with hypotension due to a decreased preload rather than right ventricle (RV) or left ventricle (LV) failure. In contrast, the physiology seen in the pulmonary vascular etiologies is consistent with RV failure. Three important types of obstructive shock are pericardial tamponade, tension pneumothorax, and massive pulmonary embolism.
Cardiac tamponade is a clinical syndrome that is caused by the rapid accumulation of fluid, pus, blood, clots, or gas within the pericardial space. This process results in impaired diastolic filling and reduced cardiac output. Classically, the clinical picture includes distended jugular veins, an exaggerated inspiratory decrease in arterial systolic pressure (pulsus paradoxus), arterial hypotension, and diminished heart sounds on cardiac auscultation.21 Bedside ECG plays a major role in the identification of cardiac tamponade and in the assessment of its hemodynamic significance.22 Hemodynamic significance is present when intrapericardial pressure exceeds intracardiac pressure. On echocardiography, this can be visualized either by the diastolic collapse of the right atrium or the right ventricle. In general, the right-sided structures are more prone to collapse than the left-sided structures due to their thinner walls. Other echocardiographic findings include inferior vena cava (IVC) dilatation associated with less than a 50% reduction in its diameter during inspiration due to impaired filling of the right-sided structures. Finally, from a Doppler perspective, augmented variation in the flow across both the mitral and tricuspid valves during respiration can be measured. Specifically, a 30% inspiratory decrease in flow across the mitral valve is considered diagnostic.23 This change in flow is reflective of the right ventricle’s inability to outwardly expand during inspiration with subsequent underfilling of left-sided structures and bulging of the septum into the LV. These respiratory changes ultimately can lead to a decrease in stroke volume and form the basis of the pathophysiology behind pulsus paradox. However, these changes in flow should not be used in isolation to diagnose tamponade. When a diagnosis of pericardial tamponade is confirmed, either percutaneous or surgical drainage is necessary.
A pneumothorax can occur if there is a breach through the visceral or parietal pleura. This breach creates a 1-way valve where air enters but cannot escape24-37 (Table 4-3). In a healthy, spontaneously breathing patient, the normal intrapleural pressure (IPP) is approximately –5 to –8 cm H2O, with the potential to reach –80 cm H2O at forced maximal inspiratory effort. In a ventilated patient, the IPP is often greater than +20 cm H2O due to the application of positive pressure. The literature has used several different definitions for tension pneumothorax, which have included mediastinal shift, the hiss of air on thoracic needle decompression, and hemodynamic compromise. Ultimately, the diagnosis of a tension pneumothorax is mostly clinical and a delay in treatment can be catastrophic.
Clinical Signs of Pneumothorax in Spontaneously Breathing Patients | Clinical Signs of Pneumothorax in Ventilated Patients |
---|---|
|
|
Chest radiographs may be used to confirm the presence of a pneumothorax in stable patients. However, waiting for a radiograph should not precede emergent decompression in patients with a high suspicion of tension pneumothorax and the following scenarios: hypoxia less than 92%; hypotension (systolic blood pressure [SBP] < 90%); respiratory rate (RR) less than 10; altered mental status; or cardiac arrest.24
Decompression can be initially achieved via needle thoracostomy, followed by tube thoracostomy. Needle decompression involves insertion of a 14-gauge (4.5-cm) needle in the second/third intercostal space (ICS) at the midclavicular line (MCL). Failure to decompress the pleural space has been attributed to increases in chest wall thickness up to 5 cm in that location, needle obstruction, loculated pneumothoraces, or large air leaks.24,38 The fourth/fifth ICS at the midaxillary line has less muscle tissue and fat and is another potential location.24,39 After needle thoracostomy has been inserted, tube thoracostomy is placed and usually suction is applied.
The distinction between submassive pulmonary embolism (PE) and massive PE is predominantly dependent on hypotension, either an SBP less than 90 mmHg or a 40-mmHg drop in BP for at least 15 minutes.40 Fibrinolysis has been advocated as the initial mode of treatment for those in shock, as it has been shown to reduce mortality by up to 55% in some studies.40-45 While alteplase 100 mg intravenously (IV) for 2 hours is the drug of choice, there have been reports that use 50 mg IV for those patients at higher perceived risk of bleeding.40-45 In general, a partial thromboplastin time (PTT) should be obtained after the infusion finishes and systemic heparin initiated when the PTT is less than 80 seconds or twice the upper limit of normal. If the PTT is greater than 80 seconds, a repeat PTT should be obtained after 4 hours. The presence of the McConnell sign on a transthoracic echo (TTE), hypokinesis of the RV free wall and base, with sparing of the apex, may help to differentiate RV failure secondary to PE from chronic RV failure.
There are controversies regarding fluid management in obstructive shock. Based on the pathophysiology of an overloaded right ventricle, with septal bowing and LV dysfunction, volume loading may further increase right ventricular overload.46 In contrast, for some patients, diuresis may be more beneficial than volume loading.47 Ultimately, the etiology of the obstructive shock state needs to be corrected and either fluids or diuretics should be used judiciously on a patient-by-patient basis.
Low peripheral vascular resistance and a normal to increased cardiac output characterize distributive shock. It is often described as warm shock because of the characteristic flushed skin, warm extremities, high respiratory rates, and bounding pulses with a normal capillary refill. If the shock state persists over the subsequent 72 hours, patients will often enter cold shock, which is characterized by cold and mottled extremities and associated with weak pulses, hypothermia, and vasoconstriction. This transition from a warm shock state to a cold shock state generally has been associated with a poorer prognosis and is considered a separate entity from sepsis-induced cardiomyopathy. The most common etiology of distributive shock is septic shock, which is discussed in Chapter 18, “Sepsis.” Other etiologies include anaphylaxis, neurogenic shock, some forms of endocrinologic conditions such as hypothyroidism (myxedema coma), and adrenal insufficiency (AI).

Full access? Get Clinical Tree
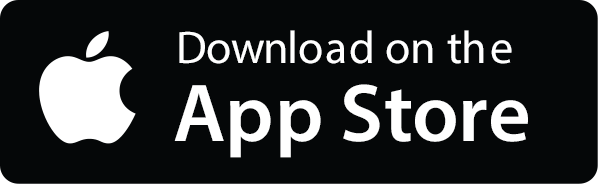
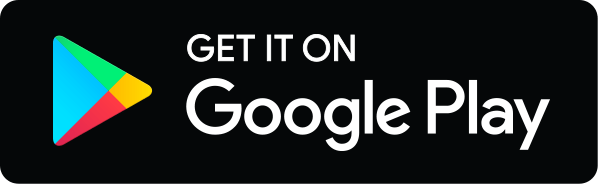