There are subtle physiologic and pharmacologic principles that should be understood for patients with neurologic injuries. These principles are especially true for managing patients with traumatic brain injuries. Prevention of hypotension and hypoxemia are major goals in the management of these patients. This article discusses the physiology, pitfalls, and pharmacology necessary to skillfully care for this subset of patients with trauma. The principles endorsed in this article are applicable both for patients with traumatic brain injury and those with spinal cord injuries.
Key points
- •
Airway management should be carefully planned, reviewed, and executed.
- •
Traumatic brain injury (TBI) and a Glasgow Coma Scale less than 9 have an almost 50% higher mortality than patients without TBI.
- •
Normocarbia should be preserved with a goal Pa co 2 between 35 and 45 mm Hg.
- •
Oxygen saturation less than 90% is associated with an increase in mortality in TBI.
- •
Hypotensive episodes can result in increased mortality even if short (<10 minutes).
Introduction
Patients with neurologic injury are prone to physiologic derangements, specifically during the period of initial stabilization, which includes establishing a definitive airway. In this article, the selection of sedatives that may help to optimize cerebral perfusion and ensure favorable outcomes is discussed. A focus on traumatic brain injury (TBI) is maintained throughout, but many of the principles discussed are applicable to any patient with a neurologic disease whereby cerebral or spinal cord perfusion may be compromised.
Background/epidemiology
TBI contributes to a growing and significant morbidity and mortality burden in the United States. In 2007, there were 1.9 million TBI-related emergency department visits, hospitalizations, and deaths; this increased almost 120% in 2013 to 2.4 million. In a study that examined TBI incidence and outcomes in California, a 57.7% increase in the number of TBI emergency department visits was observed, representing a 40.5% increase in visit rates over a 10-year period (346–487 per 100,000 residents) with 40.5% representing a revisit during the first year. As the number of TBI-related deaths has continued to increase annually, the epidemiology of various injury patterns has changed. Before 2006, most TBIs were associated with motor vehicle crashes ( Fig. 1 ). Overall, TBI-related deaths make up 2.2% of all deaths in the United States. Mortality largely comprises patients with a low Glasgow Coma Scale (GCS), especially those with a GCS less than or equal to 6 ( Fig. 2 ), with some studies citing up to a 76% mortality in patients with GCS 3.


Given the remarkable disease burden associated with TBI, prehospital, emergency, and critical care clinicians must understand the nuances of airway management. Appropriate selection of sedatives during airway management may help optimize physiology and prevent unfavorable outcomes.
Physiologic considerations in patients with traumatic brain injury
The path to intubate a patient with TBI is fraught with peril and each step should not be undertaken without expert knowledge of the physiology. From the decision to intubate through the postintubation period, the airway management of the patients with TBI with TBI should be carefully planned and skillfully executed. Similar to TBI, patients with spinal cord injury are equally at risk for complications, and many of the same physiologic principles are applicable. The current literature suggests that the possibility for harm can occur when intubating patients with TBI. Denninghoff and colleagues showed there was an increase in death in patients with TBI, with an odds ratio (OR) of 14 compared with nonintubated patients with TBI, and this increased OR persisted when adjusting for injury severity. It has also been shown that, of patients with a similar GCS, those who have a TBI and GCS less than 9 have an almost 50% higher mortality than those without TBI. Underpinning the harm of intubation in this population is the concept of secondary injury. A secondary insult, initiated by the primary injury, occurs and is characterized by a cascade of biochemical events responsible for clinical deterioration. The pathophysiology from the primary insult induces an ischemic environment in the brain via microvascular and neuronal injury. This ischemic environment precipitates a vicious cycle characterized by an impaired cerebral metabolic rate, impaired cerebral perfusion and blood flow, increased cerebral edema, and increased intracranial pressure (ICP) ( Fig. 3 ). Additional mechanisms resulting in injury include the initiation of glutamate-induced excitotoxicity and calcium channel injury leading to formation of free radicals. Secondary clinical insults include hypotension, hypoxia, hyperoxia, hypocarbia, hypercarbia, and acidosis. Hence, when selecting sedatives to facilitate airway management, this pathophysiology must be understood.

Systemic hypotension, notorious for inciting secondary injury, has long been known to correlate with increased morbidity and mortality. Hypotension can result in increased mortality even if these episodes are short (<10 minutes). However, the numerical target for blood pressure control has been widely debated. Guidelines recommend maintaining a mean arterial pressure (MAP) greater than 80 mm Hg and a systolic blood pressure (SBP) greater than 100 mm Hg, while not allowing the SBP to decrease to less than 90 mm Hg. It is also recommended to increase the threshold to an SBP of 110 mm Hg for patients aged greater than 70 years. Some investigators have argued that this target may still be too low and that an SBP closer 120 mm Hg is optimal. What seems clear in the literature is that hypotension, whether occurring early or late in a patient’s clinical course, is associated with increased mortality. In one of the most widely cited studies on this topic, by Chestnut and colleagues, a 28% increase in mortality in early (within 8 hours) shock and a 49% increase in mortality in those with late (median 31 hours) shock was reported.
Low oxygen levels also may contribute to secondary brain injury. Hypoxemia (low Pa o 2 ) leads to hypoxia (inadequate oxygen supply to neurologic structures). In the prehospital setting, 1 study showed that hypoxemia (defined as an oxygen saturation [Sp o 2 ] of <90%) was associated with a 17% increase in mortality in patients with TBI. Other reports have shown similar deleterious effects of hypoxemia. , A combination of hypoxemia and hypotension is at least additive, if not synergistic. , , In contrast, hyperoxia, likely extreme hyperoxia (arterial oxygen pressure >300 mm Hg), may also be deleterious.
Hypocapnia is well established to decrease cerebral blood volume, which decreases the intracranial volume and thus has been used to decrease ICP. Arterial CO 2 pressure (Pa co 2 ) is a potent vasoconstrictor of the cerebral arteries, causing cerebral blood flow (CBF) to decrease by about 3% per millimeter of mercury change in Pa co 2 , thus a decrease from 40 to 30 mm Hg represents a 33% decrease in brain volume. Such a decrease can result in cerebral ischemia. It is now well accepted that hyperventilation to a Pa co 2 less than 25 mm Hg is harmful, , and prolonged periods are not recommended. Thus, maintenance of normocarbia (Pa co 2 between 35 and 45 mm Hg) is a primary goal during TBI management. However, several studies have shown that this goal is infrequently attained in the prehospital and emergency settings. , Hyperventilation to a low Pa co 2 should only be used for short periods of time in imminent brain herniation as a temporizing method while mobilizing neurosurgery for a possible decompressive craniectomy. Moreover, the effect of hypocarbia is short lived and the cerebrospinal fluid is able to normalize CBF quickly in the setting of hypocarbia, within about 4 hours.
Protocolized care that allows for proper clinical decision making has been shown to improve outcomes. The EPIC (Excellence in Prehospital Injury Care) study was a statewide public health initiative in Arizona designed to implement Brain Trauma Foundation guidelines into prehospital protocols to improve adherence and minimize causes of secondary brain injury. Adjusted survival doubled in patients with severe TBI and tripled in the intubated cohort after statewide guidelines were effected. In addition, the incidence of hypoxemia and hypotension among patients with TBI was decreased.
Establishing an airway
Understanding the deleterious consequences of altered oxygen, carbon dioxide, and blood pressure is fundamental when planning an approach for airway management of a patient with TBI. The goal of airway management is to establish a definitive airway while simultaneously optimizing physiologic parameters. Rapid sequence induction and intubation (RSII) is the technique of choice for TBI. Once the decision is made to secure the airway with RSII, decisions regarding selection of induction and neuromuscular blocking agents are critical. Compounding the difficulty in selection of appropriate agents is the fact that neuronal injury is frequently associated with cardiac dysfunction. Although this is well known in nontraumatic aneurysmal subarachnoid hemorrhage, it has also been observed in cases of TBI where decompressive hemicraniectomy was required. Both cardiac and neurologic function should be considered before the administration of induction agents. Although the usual reflex responses to stimulation by intubation include glottic closure, hypertension, tachycardia, and reflex bronchoconstriction, any of a myriad of pathophysiologic derangements can occur, including hypoxia, hypotension, apnea with hypercarbia, bradycardia, and even cardiac arrest, with a nonnegligible incidence.
Adjunctive medications
A variety of medications have historically been used to attenuate increases in ICP and to attenuate other effects associated with endotracheal intubation. However, the data to support the use of adjunctive medications are sparse and often extrapolated from small case series and heterogeneous patient populations.
Lidocaine has been used before RSII to prevent hypertension, tachycardia, and increased ICP based largely on data extrapolated from patients with hydrocephalus and brain tumors. A Vaughan Williams class Ib antiarrhythmic, lidocaine, suppresses automaticity of conduction tissue and blocks both the initiation and conduction of nerve impulses by decreasing the neuronal membrane’s permeability to sodium ions. At least 2 well-conducted reviews do not support the routine use of lidocaine as a pretreatment of RSII in patients with TBI. , If used, a dose of 1 to 2 mg/kg may be considered, but the drug must be given several minutes before RSII for maximal effect. Putative advantages include blunting of fasciculations associated with succinylcholine, a relatively safe side effect profile, and variable blockade of sympathetic response during RSII. , Disadvantages include the burden of drawing up an additional medication during an emergency airway scenario, arterial hypotension, minimal ICP protection, inconsistent protection against hypertension or tachycardia, and no direct evidence of benefit. ,
A Cochrane Review examined the role of multiple different adjunctive medications, including lidocaine, β-blockers, calcium channel blockers, alpha-agonists, and opioids. The review found a decrease in the OR of arrhythmias (OR, 0.1; 95% confidence interval [CI], 0.14–0.26) but a much larger OR of adverse events, including hypotension and bradycardia for local anesthetics (OR, 12.27; 95% CI, 4.03–37.36) and adrenergic blockers (OR, 24.17; 95% CI, 8.88–65.79). For opiates, the adverse events OR was much higher (OR, 149.5; 95% CI, 35.07–637.65), including at least 1 case of chest rigidity (alfentanil). Thus, the use of adjuncts should be considered on a case-by-case basis, balancing the harm and benefit.
Induction agents
There are no firm recommendations favoring one induction agent rather than another in terms of neuroprotective effects. Ultimately, the ideal drug is the one with which the clinician is most comfortable. Table 1 lists properties and doses of commonly used induction agents. For hemodynamically unstable patients, one technique that may help with dosing involves the use of the shock index (heart rate/blood pressure). As a composite measure for hemodynamic instability (ie, a shock index >0.9), shock index–based dosing may prevent the exacerbation of hypotension, including populations of patients at risk for this complication ( Table 2 ). Midazolam has not been extensively studied as either a sole or adjunctive medication for RSII, but, in a retrospective review of 219 patients who had prehospital RSII, use of this agent as an induction agent was associated with a dose-related incidence of hypotension. Similarly, use of fentanyl as a single-drug induction agent in patients with trauma produced worse neurologic variables in a small retrospective study. Hence, the use of opioids and benzodiazepines as sole induction agents is not discussed here; this article emphasizes the 3 principal sedative agents used for RSII in TBI and patients with neurologic injuries: propofol, etomidate, and ketamine.
Agent | MAP | ICP | CBF | CPP | CMR | Dose (mg/kg) |
---|---|---|---|---|---|---|
Propofol | <SPAN role=presentation tabIndex=0 id=MathJax-Element-1-Frame class=MathJax style="POSITION: relative" data-mathml='↓’>↓↓ ↓ | <SPAN role=presentation tabIndex=0 id=MathJax-Element-2-Frame class=MathJax style="POSITION: relative" data-mathml='↓’>↓↓ ↓ | <SPAN role=presentation tabIndex=0 id=MathJax-Element-3-Frame class=MathJax style="POSITION: relative" data-mathml='↓’>↓↓ ↓ | <SPAN role=presentation tabIndex=0 id=MathJax-Element-4-Frame class=MathJax style="POSITION: relative" data-mathml='↓’>↓↓ ↓ | <SPAN role=presentation tabIndex=0 id=MathJax-Element-5-Frame class=MathJax style="POSITION: relative" data-mathml='↓’>↓↓ ↓ | 1–2 |
Propofol (in shock) a | — | <SPAN role=presentation tabIndex=0 id=MathJax-Element-6-Frame class=MathJax style="POSITION: relative" data-mathml='↓’>↓↓ ↓ | <SPAN role=presentation tabIndex=0 id=MathJax-Element-7-Frame class=MathJax style="POSITION: relative" data-mathml='↓’>↓↓ ↓ | <SPAN role=presentation tabIndex=0 id=MathJax-Element-8-Frame class=MathJax style="POSITION: relative" data-mathml='↓’>↓↓ ↓ | <SPAN role=presentation tabIndex=0 id=MathJax-Element-9-Frame class=MathJax style="POSITION: relative" data-mathml='↓’>↓↓ ↓ | 0.5–1 |
Etomidate | <SPAN role=presentation tabIndex=0 id=MathJax-Element-10-Frame class=MathJax style="POSITION: relative" data-mathml='↔’>↔↔ ↔ | <SPAN role=presentation tabIndex=0 id=MathJax-Element-11-Frame class=MathJax style="POSITION: relative" data-mathml='↓’>↓↓ ↓ | <SPAN role=presentation tabIndex=0 id=MathJax-Element-12-Frame class=MathJax style="POSITION: relative" data-mathml='↓’>↓↓ ↓ | <SPAN role=presentation tabIndex=0 id=MathJax-Element-13-Frame class=MathJax style="POSITION: relative" data-mathml='↑/↓/↔’>↑/↓/↔↑/↓/↔ ↑ / ↓ / ↔ | <SPAN role=presentation tabIndex=0 id=MathJax-Element-14-Frame class=MathJax style="POSITION: relative" data-mathml='↓’>↓↓ ↓ | 0.15–0.3 |
Ketamine | <SPAN role=presentation tabIndex=0 id=MathJax-Element-15-Frame class=MathJax style="POSITION: relative" data-mathml='↑’>↑↑ ↑ | <SPAN role=presentation tabIndex=0 id=MathJax-Element-16-Frame class=MathJax style="POSITION: relative" data-mathml='↓’>↓↓ ↓ b | <SPAN role=presentation tabIndex=0 id=MathJax-Element-17-Frame class=MathJax style="POSITION: relative" data-mathml='↑’>↑↑ ↑ | <SPAN role=presentation tabIndex=0 id=MathJax-Element-18-Frame class=MathJax style="POSITION: relative" data-mathml='↑’>↑↑ ↑ | <SPAN role=presentation tabIndex=0 id=MathJax-Element-19-Frame class=MathJax style="POSITION: relative" data-mathml='↑/↓’>↑/↓↑/↓ ↑ / ↓ c | 1–2 |

Full access? Get Clinical Tree
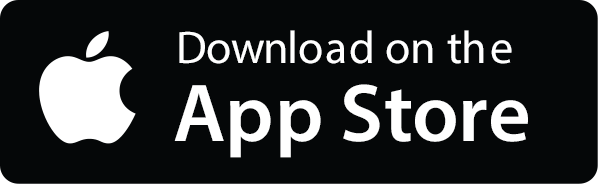
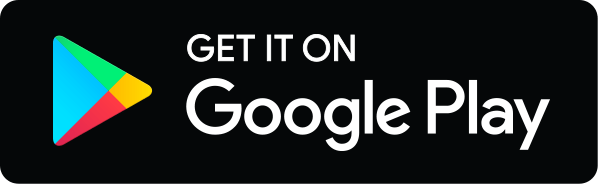
