Abstract
Cannabis has been used in medicine for centuries. Modern understanding of the clinical effects of cannabis and cannabinoids in the treatment of pain is evolving. Cannabinoid compounds include phytocannabinoids, endocannabinoids, and synthetics. The two main phytocannabinoids are delta-9-tetrahydrocannabinol (THC) and cannabidiol (CBD). The endocannabinoid system is comprised of endogenous cannabinoids and cannabinoid receptors including CB1 receptors located primarily in the brain and peripheral tissue and CB2 receptors in the immune and hematopoetic systems. Cannabis bioavailability and metabolism varies significantly for inhaled versus oral/sublingual routes of delivery. Large, well-designed clinical trials are limited; however the studies available provide evidence to support the use of cannabinoids for some cancer, neuropathic, and chronic pain conditions.
Keywords
cannabinoids, cannabis, dispensaries, endocannabinoid system, medical marijuana
Cannabinoids are a diverse group of herbal, endogenous, and synthetic compounds that bind to specific inhibitory receptors in the body. The most common source of cannabinoids is the Cannabis plant, which has been used recreationally, industrially, and medicinally for thousands of years. In modern times, few substances have carried more stigma or ignited more debate. However, over the past 20 years, the public and medical community’s attitude towards cannabinoids has been shifting. While it remains illegal federally, multiple US states have loosened state restrictions, including decriminalizing possession, legalizing medicinal use, and approving recreational use. With this changing landscape come many challenging questions from providers and patients: What role do cannabinoids play in alleviating pain? Should physicians recommend cannabinoids for the treatment of pain? What are the risks and side effects? How do patients obtain and use a cannabinoid drug? How should physicians who choose to recommend cannabinoids select appropriate patients and monitor them? What are the legal and regulatory issues that providers and researchers face in dealing with cannabinoids?
History of Cannabinoids in Medicine
Cannabis is one of the oldest documented herbal medicines, used for at least 5000 years. It was initially utilized for its industrial properties to produce paper, textiles, and clothing, and as an important food source of hemp milk, oil, and seed. Around the 1st century, the Chinese pharmacopoeia recommended its use for rheumatic pain, constipation, disorders of the female reproductive system, and malaria. The ancient Indians used it to treat headaches, insomnia, gastrointestinal disorders, and pain during childbirth. The West remained isolated from cannabis until the mid-19th century, when an Irish physician serving in the British military force in India documented cannabis’s medicinal properties, igniting its spread into Europe and the Americas. In 1845, it was first listed in the United States Dispensatory as a sedative, analgesic, and anticonvulsant. Popularity for this herbal remedy continued, and its indications expanded to include: headaches, migraine, eye-strain, menopause, brain tumors, neuralgia, gastric ulcer, uterine disturbances, dysmenorrhea, chronic inflammation, acute rheumatism, tingling, and relief of dental pain. As the production of medicines became more commercial in the late 1800s, pharmaceutical companies marketed tinctures and extracts of cannabis leading to increased public consumption.
In the early 20th century, there was an overall decline in herbal remedies—including cannabis—as pharmaceutically synthesized compounds like barbiturates, aspirin, and opioids became the accepted norm. Additionally, cannabis’s psychotropic effects, criminal associations, and lack of regulation led to further decrease in public consumption. In 1937, Congress passed the Marihuana Tax Act restricting and taxing the medical and nonmedical use of the drug. This led to the removal of cannabis from the American Pharmacopeia. In 1970, the Controlled Substances Act (CSA) classified cannabis as a Schedule I drug, cementing its status as an illegal substance without acceptable medical use. This increasingly restrictive environment contrasted with emerging research into cannabis’s pharmacological properties. The 1940s marked the first experiments performed using single cannabinoids. Tetrahydro-cannabinol (THC), the major psychoactive ingredient in cannabis, was isolated in the 1960s by Mechoulam and Gaoni, ushering in a new understanding into the mechanisms and effects of cannabinoids on the central nervous system (CNS). This was further solidified in the 1980s and 1990s by the discovery of endogenous cannabinoids (endocannabinoids) and their specific receptor targets, cannabinoid receptor type 1 and 2 (CB1 and CB2).
The 1990s brought about a more permissive view of cannabinoids as US states, like California and Arizona, passed the first medicinal marijuana legislation (Arizona’s referendum was invalidated 5 months later, but has since been reinstated by voters). Currently, 23 US states (plus the District of Columbia) have approved the medicinal use of cannabis in various forms. Four states have legalized its recreational use, while several others have decriminalized its possession. This legalization trend is likely to continue, with increasing calls for the Federal government to reevaluate its classification of cannabis as a Schedule I drug.
The medicinal cannabinoid debate, specifically relating to marijuana, remains quite polarized. Its supporters argue that the herb contains numerous active constituents making it potentially more clinically effective than any synthetic or single constituent pharmaceutical. Moreover, it has a superior safety profile compared to analgesics like opioids because of its lack of lethal dose or risk of respiratory depression, substantiated by millennia of use. Opponents, on the other hand, argue that because it is a plant, its contents are nonstandardized and thus highly variable. Additionally, cannabis is addictive, impairs cognitive function, and has potential long-term risks, particularly in children and adolescents. Finally, removing the Schedule I classification of cannabis would lead to more widespread availability and recreational use, leading to untold public harm.
Classification of Cannabinoids
The term cannabinoid refers to both endogenous and exogenous compounds that bind to specific inhibitory cannabinoid receptors, ultimately decreasing neurotransmitter release. Historically, it referred to compounds derived from the Cannabis plant. With the discovery of human cannabinoid receptors, endogenous cannabinoids, and the development of new synthetic nonherbal compounds with similar effects, the term now applies more broadly to any compound having an interaction with these cannabinoid receptors. Cannabinoids can be divided into three types: phytocannabinoids (compounds derived from cannabis), synthetics (manufactured compounds), and endocannabinoids (compounds produced by the body, which interact with cannabinoid receptors) ( Fig. 56.1 ).

Phytocannabinoids (Cannabis and its Derivatives)
Cannabis is the genus of the family, cannabaceae (hemp). Cannabis is an herb that grows in temperate and tropical climates and has three major subspecies: Cannabis sativa, Cannabis indica, and Cannabis ruderalis. Although there is ongoing taxonomical controversy, it is generally thought that these subspecies are distinct in morphology and origin. Anecdotally, Cannabis sativa has more psychoactive features compared to indica, though this is a matter of ongoing debate as both have significant levels of THC. These two subspecies are commonly referred to as “marijuana.” Cannabis sativa is also the most widespread of the species, growing in almost all climates. There are wide varieties of cannabis hybrid plants that combine desired features of each of these subspecies. This has led to a plethora of cannabis strains with unique cannabinoid profiles based on the cultivation conditions, as well as selection and breeding. Cannabis ruderalis usually has very low amounts of THC (<1%) but may be a good source of cannabidiol (CBD) extract.
The cannabis plant contains over 400 chemical compounds, of which at least 80 are cannabinoids. Delta-9-tetrahydrocannabinol (Δ 9-THC) is the most well-known and the primary psychoactive compound in cannabis. It mimics the action of anandamide (AEA), an endogenous cannabinoid in humans, having approximately equal affinity for the CB1 and CB2 receptors. Other cannabinoids include CBD, delta-8-tetrahydrocannabinol (Δ 8-THC), cannabigerol (CBG), and cannabinol (CBN). The concentration of cannabinoids in each cannabis strain can vary tremendously. Due to selective breeding, the concentration of THC in recreational cannabis seized by the Drug Enforcement Administration (DEA) has increased from an average of 3% in the 1980s to 13% in 2009. More recently in medical marijuana dispensaries and recreational cannabis shops, the THC concentrations advertised range from the mid-teens to as high as 34%. The majority of these strains contain CBD in very low concentrations, usually under 1%. There are strains with lower THC concentrations available, as well as “high CBD” strains with concentrations generally in the range of 5% to 15%.
CBD is the second most abundant compound in cannabis after THC. It is thought to have wider medical applications than THC, which has fueled the demand for medical marijuana and pharmaceuticals with higher CBD concentrations compared to recreational marijuana. CBD is generally considered to have no psychoactive effects, but clinically it has been reported to reduce seizures, improve muscle spasm, and reduce inflammation. It has a very low affinity for the CB1 and CB2 receptors, and may act as an inverse agonist/antagonist. These interactions with the CB receptors may attenuate some of the psychotropic effects of THC. The majority of the marijuana strains available in medical marijuana dispensaries and recreational shops contain predominately THC, with CBD in very low concentrations. However, there are low THC strains available, which have higher CBD concentrations ranging from 5% to 15%. CBN, a metabolite of THC, is minimally psychoactive and found in only trace amounts in the plant. Δ 8-THC is also minimally psychoactive and present at low concentrations.
In addition to whole-leaf cannabis plants, there is another class of phytocannabinoids collectively referred to as cannabis-based medicine extracts (CBME). These are derived by extracting compounds directly from cannabis plants. There are two subtypes of CBMEs. The first are those produced by pharmaceutical companies under well-regulated, controlled conditions, and undergoing rigorous clinical trials. The second are products produced and sold in medical marijuana dispensaries without any regulatory oversight or clinical trials. There is increasing interest in CBD oil extracts for its wide range of medicinal uses. Industrial hemp, which is used in manufacturing thousands of commercial products, has a high CBD content and is used for CBD extracts in other countries. Although industrial hemp contains very low levels of THC (0.3%), legally grown hemp in the United States still falls under Schedule I drug policy so CBD extraction is not allowed. However, CBD extracts from hemp in other countries can legally be sold in the United States as found on websites, health food stores, and dispensaries.
There are three pharmaceutically manufactured CBMEs that have undergone clinical trials. Nabiximols (Sativex) is a sublingual spray with a 1:1 ratio of THC to CBD. Cannador is an oral capsule with a 2:1 ratio of THC to CBD. Epidiolex is a solution of purified CBD. Nabiximols is currently approved in Canada and Europe for the treatment of spasticity in multiple sclerosis (MS). In Canada, it also has a conditional license as an adjunctive medication for MS-related neuropathic pain and cancer-related pain. In the United States it has been studied in clinical trials, which initially showed promising results for advanced cancer pain. However, the most recent phase III trial failed to show superiority over placebo, and thus far it does not have Food and Drug Administration (FDA) approval. Cannador has been used in several research studies in patients with MS spasticity and parkinsonian dyskinesia, but is not clinically available. The CBD-based extract Epidiolex is being studied to treat certain rare forms of epilepsy and is currently undergoing phase III trials.
The category of nonpharmaceutically derived CBMEs contains a large variety of products made from the cannabis plant and sold in medical marijuana dispensaries. These include extracts, oils, tinctures, patches, topical creams, and pills. The delivery routes of these cannabinoid products include oral administration, vaporizer inhalation, transmucosal absorption, transdermal delivery, and rectal suppository. Additionally, most dispensaries sell a variety of foods, drinks, and candies infused with cannabinoids. There is wide variation in cannabinoid concentrations in these products, and there are no clinical studies on their effects for pain or other conditions.
Synthetic Cannabinoids
There are two FDA-approved synthetic cannabinoid medications, dronabinol (Marinol) and nabilone (Cesamet). Dronabinol is a Schedule III synthetic form of Δ 9-THC, which is marketed in the United States for the treatment of nausea associated with chemotherapy, and as an appetite stimulant for HIV-related wasting syndrome. Nabilone is a Schedule II synthetic analogue of Δ 9-THC, which is FDA approved to treat chemotherapy-induced nausea. Both dronabinol and nabilone have been studied for the treatment of chronic pain and spasticity. Ajulemic acid is a newer synthetic analogue of the nonpsychoactive terminal metabolite of Δ 8-THC. It is currently being investigated as an antiinflammatory and analgesic agent. A newer highly purified version of this molecule (Resunab) is currently under clinical investigation for a variety of inflammatory diseases.
Endocannabinoid System
The endocannabinoid system (ECS) refers to the human body’s network of cannabinoid neuromodulators (endocannabinoids), enzymes, and receptors. This intricate system is influenced by the phytocannabinoids in cannabis. The ECS is thought to regulate myriad behavioral processes, including pain, mood, memory, learning, addiction, and appetite. Until the cannabinoid receptors were discovered, it was thought that cannabinoid effects in the body were nonspecific, due to lipophilic disruption of the phospholipid membrane. However, discovery of CB1 and CB2 receptors and advances in genotyping and pharmacology in the 1990s led to the understanding of receptor-specific processes.
The two primary endocannabinoids are AEA and 2-arachidonoylglycerol (2-AG). These eicosanoids are synthesized in postsynaptic neurons and act as retrograde signaling messengers. During neurotransmission between a presynaptic and postsynaptic neuron, there are a multitude of excitatory, inhibitory, and secondary messenger systems at play. Endocannabinoids are thought to be produced on-site/on-demand from cell membrane lipid precursors to inhibit the presynaptic release of a variety of neurotransmitters, including acetylcholine, dopamine, gamma amino butyric acid (GABA), glutamate, serotonin, norepinephrine, and endorphins ( Fig. 56.2 ).

Anandamide (AEA), named from the Sanskrit word Ananda , meaning bliss, is an amide of ethanolamine and arachidonic acid. It is generated from its membrane precursor, N -arachidonoyl phosphatidylethanolamine (NAPE) via a multitude of pathways including phospholipase A2, phospholipase C, and phospholipase D. 2-AG, on the other hand, is synthesized from diacylglycerol through Ca-dependent pathways including diacylglycerol lipase and phosphatidycholine. AEA is degraded in the postsynaptic terminal primarily by fatty acid amino hydrolase (FAAH), whereas 2-AG is mostly degraded presynaptically by monoacylglycerol lipase (MAG). Both of these endocannabinoids are released into the presynaptic space by some combination of simple diffusion and facilitated, carrier-mediated transport.
Pharmacodynamically, AEA and 2-AG bind to the G-protein coupled receptors (GPCRs), CB1 and CB2, which are located in the CNS and the peripheral nervous system. The analgesic properties of AEA likely involve its partial agonism (moderate affinity, low efficacy) of the CB1 receptor, which, in turn, decreases the release of excitatory neurotransmitters. It has also been shown to act as a weak agonist at the transient receptor potential vanilloid 1 (TRPV-1) receptors. The TRPV-1 receptors are expressed in nociceptive sensory neurons and respond to noxious mechanical, thermal, and chemical stimuli. AEA and capsaicin share the same TRPV-1 binding site, but higher concentrations of AEA are required in order to activate TRPV-1. Unlike AEA, 2-AG is found in much higher concentrations in the brain (up to 170-fold) and is thought to be a full agonist (low affinity, high efficacy) of the CB1 receptor.
Pharmacology of Cannabinoids
The precise pharmacology of most cannabinoids remains unknown. However, researchers have elicited major mechanisms underlying the active compounds in cannabis including THC, CBD, and CBN. Complicating this is the highly variable absorption due to the plethora of delivery forms and routes. These include inhalation and ingestion, as well as absorption via oral, sublingual, topical, or rectal application. For centuries, the primary means of delivering cannabinoids has been via the inhaled smoke of cannabis or hashish. The variable concentration of THC and other cannabinoids in cannabis, the lack of controlled production and testing in most medical marijuana products, and the diversity of delivery routes makes the prediction of pharmacologic effects difficult.
Activation Delivery and Absorption of Cannabinoids
Patients who prefer not to smoke are increasingly using cannabis in a multitude of other forms. Cannabis vaporizers, similar to tobacco vaporizers or e-cigarettes, heat the plant material and vaporize the active ingredients (due to the low volatility of cannabinoids) without resulting in combustion or smoke. The use of cannabis-infused foods, candies, and drinks, or “edibles,” has surged in popularity due to their slower onset of action and longer-lasting effects. Many of these food products are produced with butter or oil that has been infused with cannabinoids. The buds of the cannabis plant are heated in oil to extract the cannabinoids and decarboxylate the THC into its active form. The ingestion of the raw cannabis plant, without first heating and decarboxylating the THC, has little pharmacologic effect. The delayed onset and variable absorption of edibles often prolongs the clinical effects but increases the risk of overdose. Additionally, many of the commercially produced edibles are sweets and candies, and pose a temptation and risk of children being exposed. Tinctures, oils, and tonics are available in medical marijuana dispensaries, which can be administered sublingually or added to food and drink items. Cannabis topicals, including lotions, sprays, creams, and balms, are marketed to provide local analgesic and antiinflammatory effects. Finally, products such as hash and wax contain very potent concentrations of cannabinoids that can be eaten, smoked, or ingested.
Pharmacokinetics
The metabolism of cannabinoids occurs in the liver via the cytochrome P450 (CYP450) systems CYP2C subfamily of isoenzymes, particularly CYP2C9; therefore, this can result in decreased metabolism of other drugs that use the CYP450 system. Some metabolism occurs in the lungs as well as other tissues. Elimination is primarily in the form of acid metabolites both renally and through biliary excretion with only 5% eliminated unchanged. Extensive tissue storage of metabolites results in prolonged elimination, which may explain the lack of or reduced withdrawal symptoms with chronic use and abrupt cessation. The detection of metabolites in urine will fluctuate between positive and negative results for days after last use. For infrequent users, the average time to the first negative urine test is 8.5 days with 12.9 days to the last positive test. For frequent users, the average time to first negative test is 19.1 days with 31.5 days to the last positive test.
Inhaled cannabis, whether smoked or vaporized, is the most popular delivery mechanism. It is easier to titrate than ingestion, producing fast and relatively predictable results. Smoking cannabis results in rapid absorption, delivering into the lungs approximately 50% of its THC content in the inhaled smoke. Fifty percent or more of this inhaled THC is then lost to exhalation and local metabolism in the lung, leaving a bioavailability for the active drug between 10% and 35%. Bioavailability varies depending on depth of inhalation, puff duration, and breath-holding with regular users being more efficient. Vaporization is another means of inhaled delivery without burning the cannabis and results in higher bioavailability. The volatile cannabinoids are vaporized when heated air is drawn through the cannabis. Alveolar absorption results in maximal plasma concentration within minutes, and psychotropic effects starting within a few seconds and reaching maximal effect within 15 to 30 minutes. Psychotropic effects with inhalation will last 2 to 3 hours.
The oral administration of cannabis produces slow, but relatively high, absorption with peak plasma concentrations at 60 to 120 minutes. However, first-pass metabolism results in a low bioavailability of 6% to 20% with significant variability. The delay in peak blood levels of THC from oral administration makes self-titration more difficult. Psychotropic effects begin within 30 to 90 minutes after oral ingestion, reaching maximal effect after 2 to 3 hours and may last 4 to 12 hours. Absorption of the cannabinoids can be increased with coingestion of a fatty meal.
Pharmacodynamics
The location of the cannabinoid receptors and their respective interactions with these ligands is a field of extensive study. CB1 receptors are located mainly in the CNS at nerve terminals where they mediate inhibition of transmitter release. In the brain, they are located in the cerebral cortex, hippocampus, caudate-putamen, substantia nigra, pars reticulata, cerebellum, the mesolimbic system, as well as the brainstem and spinal cord. The CB2 receptor is found mainly on immune cells including microglia, monocytes, macrophages, and B and T lymphocytes where they modulate cytokine release. Both CB1 and CB2 receptors are coupled through G proteins, negatively to adenylate cyclase (inhibiting the production of cyclic adenosine monophosphate [AMP]), and positively to mitogen active protein kinase (MAPK). Additionally, CB1 is coupled positively to inwardly rectifying and A-type outward potassium channels, and negatively to N-type and P/Q-type calcium channels. Within the spinal cord, CB1 receptors have been localized to multiple areas involved in nociceptive processing including the superficial dorsal horn, dorsolateral funiculus, and lamina X. The activation of CB1 receptors in central nociceptive processing regions and primary afferents inhibits the release of neurotransmitters via decreased calcium conductance and increasing potassium conductance, which forms the anatomical basis for the analgesic action of cannabinoid agonists (see Fig. 56.2 ). Additionally, there are CB1 receptors located in peripheral tissues in adipocytes, liver, lung, reproductive organs, smooth muscle, gastrointestinal tract, immune system, and peripheral sensory nerves.
CB2 receptors play a role in mediating analgesia through reducing inflammation in the periphery. The CB2 receptors are found in the spleen, tonsils, thymus, and other tissues responsible for immune cell production and regulation. Activation of CB2 receptors down-modulates mast cell function with evidence that it can trigger microglial cell migration and regulate cytokine release. The effect of CB2 on mast cells may play a role in modulating nerve growth factor (NGF)–driven sensitization of nerve terminals during inflammation, which has been implicated in the development of inflammatory hyperalgesia. There is also evidence that CB2 receptors are located on and play a role in nociceptive regulation of primary sensory neurons.
Emerging research suggests that there might be a third cannabinoid receptor. One possible candidate is the orphan receptor GPR55, based on its sequence homology to CB1 and CB2. This GPCR expressed in the putamen and caudate nucleus responds to cannabinoid ligands particularly the endogenous lysophosphatidylinositol. Further research is likely to yield more cannabinoid and cannabinoid-like receptors and ligands as advanced genetic and pharmacological technologies emerge.
Historically, the study of cannabinoids has focused mainly on THC. Recently, there has been an interest in coadministration of CBD and THC due to evolving research highlighting CBD’s neuroprotective and medicinal effects. CBD as a sole agent does not produce any of the psychoactive effects associated with THC, and has a multitude of analgesic, anxiolytic, antiepileptic, antinausea, and antipsychotic actions. Moreover, it is thought to antagonize many of THC’s psychotropic and other adverse reactions; however, the associated mechanism remains unclear. This effect of CBD has been shown in a few animal and human studies where it attenuated some of the anxiety, paranoia, mental slowing, and tachycardic effects produced by THC. Findings like these have supported the surge in low-THC/high-CBD cannabis products that might have more targeted roles for pain and inflammation without some of the adverse psychomimetic features. Even more recently, there has been a shift in drug development to focus on peripheral cannabinoid targets, thereby eliminating the central psychotropic effects completely.
Cannabinoids as Analgesics
There are a limited number of randomized, controlled, clinical trials (RCTs) involving cannabinoids for the treatment of pain. Stimulated by the burden of chronic pain globally, and the need to find safer, nonopioid therapeutic targets, the number of studies has been rising. Complicating this area of research, however, are complex federal regulatory issues due to the Schedule I status of cannabinoids, and the lack of standards for cannabinoid form and administration across various studies. The studies differ in the type of cannabinoid (plant, extract, synthetic), route of administration (inhalation, ingestion, mucosal absorption), and dosing that create unique challenges in interpretation. All of the current studies have focused on THC. There are no studies focusing on CBD, although there is increasing interest given its lack of psychoactive properties. To date, all studies that used cannabis supplied by the National Institutes of Health (NIH) had CBD levels of less than 1%. A summary of select RCTs across several pain conditions is highlighted in Table 56.1 .
Pain Model | Drug (Route) | Outcome | Side Effects | Author, Year |
---|---|---|---|---|
Healthy volunteers | Cannabis (smoked) | No effect (low dose) Decreased pain (moderate dose) Increased pain (high dose) | Mild | Wallace et al., 2007 |
Healthy volunteers | THC (oral) | No effect | Mild | Naef et al., 2003 |
Healthy volunteers | Cannabis (smoked) | Decreased pain | Mild | Greenwald and Stitzer, 2000 |
Healthy volunteers | Cannabis (smoked) | Decreased pain | Mild | Greenwald and Stitzer, 2000 |
Healthy volunteers | Cannabis (smoked) | Decreased pain | Mild | Milstein et al., 1975 |
Healthy volunteers | Cannabis (smoked) | Increased pain | Clark et al., 1981 | |
Healthy volunteers | Cannabis (smoked) | Increased pain | Hill et al., 1974 | |
Healthy volunteers | THC (oral) | No effect | Mild | Zeidenberg et al., 1973 |
Acute pain | Cannador (oral) | Decreased pain (dose dependent) | Mild | Holdcroft et al., 2006 |
Acute pain | THC analogue (oral) | Increased pain | Mild | Beaulieu, 2006 |
Acute pain | THC (oral) | No effect | Mild | Buggy et al., 2003 |
Acute pain | Levonantradol (IM) | Decreased pain | Mild | Jain et al., 1981 |
Acute pain | THC (IV) | Decreased pain (low dose) Increased pain (high dose) | Moderate | Raft et al., 1977 |
Chronic pain | Nabiximols (SL spray) | Decreased pain | Mild | Narang et al., 2008 |
Chronic pain | THC/cannabidiol (SL spray) | Decreased pain | Mild | Blake, 2006 |
Chronic pain | THC (SL spray) | Decreased pain | Mild | Notcutt et al., 2004 |
Chronic pain | THC/cannabidiol (SL spray) | Decreased pain | Mild | Notcutt et al., 2004 |
Chronic pain | Cannabidiol (SL spray) | No effect | Mild | Notcutt et al., 2004 |
Neuropathic pain | Cannabis (smoked) | Decreased pain (dose dependent) | Mild | Wallace et al., 2015 |
Neuropathic pain | Nabixomols (SL spray) | Decreased pain | Mild | Serpell et al., 2014 |
Neuropathic pain | Cannabis (smoked) | Decreased pain (high dose) | Mild | Ware et al., 2010 |
Neuropathic pain | Cannabis (smoked) | Decreased pain (high dose) | 2 cases of toxic psychosis | Ellis et al., 2009 |
Neuropathic pain | Cannabis (smoked) | Decreased pain (high dose) | Mild | Wilsey et al., 2008 |
Neuropathic pain | Cannabis (smoked) | Decreased pain | Mild | Abrams et al., 2007 |
Neuropathic pain | Nabiximols (SL spray) | Decreased pain | Mild | Nurmikko et al., 2007 |
Neuropathic pain | Nabiximols (SL spray) | Decreased pain | Mild | Rog et al., 2005 |
Neuropathic pain | Nabiximols (SL spray) | Decreased pain | Mild | Berman et al., 2004 |
Neuropathic pain | Synthetic analog of THC (oral) | Decreased pain | Mild | Karst et al., 2003 |
Neuropathic pain | Cannador (oral) | Decreased pain | Mild | Zajicek et al., 2003, 2005 |
Neuropathic pain | Nabiximols (SL spray) | Decreased pain | Mild | Wade et al., 2003 |
Cancer pain | Nabixomols (SL spray) | Decreased pain (low and middle dose) No effect (high dose) | Mild | Portenoy et al., 2012 |
Cancer pain | Nabixomols (SL spray) + THC extract | Decreased pain (nabiximols) Increased pain (THC extract) | Mild | Johnson et al., 2010 |
Cancer pain | Benzopyranoperidine (oral) | Increased pain | Yes (similar to codeine) | Jochimsen et al., 1978 |
Cancer pain | Benzopyranoperidine (oral) | Decreased pain | Yes (similar to codeine) | Staquet et al., 1978 |
Cancer pain | THC (oral) | Decreased pain | Yes | Noyes et al., 1975 |

Full access? Get Clinical Tree
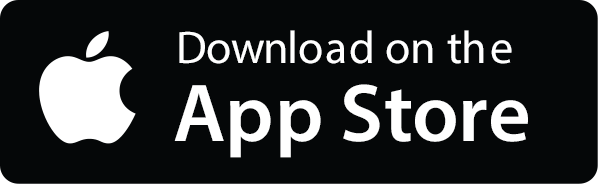
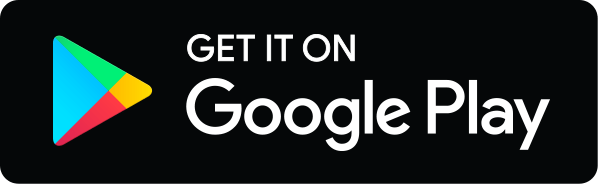
