91 Resuscitation from Circulatory Shock
Understanding Underlying Pathophysiology of Global Flow and Oxygen Delivery
Addressing Global Adequacy of Tissue Oxygenation
Adequacy of tissue oxygenation is defined as an adapted oxygen supply (or DO2) to oxygen demand.1 Oxygen demand varies according to tissue type and according to time. Although oxygen demand cannot be measured or calculated, oxygen uptake or consumption () and DO2 both can be quantified; they are linked by a simple relationship:
where ERO2 represents oxygen extraction ratio (ERO2 in %; and DO2 in mL O2/kg/min). DO2 represents the total flow of oxygen in the arterial blood and is given as the product of cardiac output (
) by arterial oxygen content (CaO2):
with CaO2 being the product of hemoglobin (Hb, g/100 mL) by arterial oxygen saturation (SaO2, %) and Hb O2 capacity (1.39 mL O2/g Hb): CaO2 = Hb × SaO2 × 1.39.
During circulatory shock and/or severe hypoxemia, as DO2 declines secondary to a decrease in and/or a decrease in CaO2,
can be maintained by a compensatory increase in ERO2,
and DO2 remaining therefore independent. But as DO2 falls further, a critical point (DO2 crit) is reached; ERO2 can no longer compensate for this fall in DO2, and at this critical level,
becomes DO2 dependent (Figure 91-1). At this DO2 crit (4 mL/kg/min), for a
of about 2.4 mL/kg/min, ERO2 reaches its critical point (ERO2 crit) of 60%. When
is higher, DO2 crit is higher as well. Increase in oxygen extraction occurs via two fundamental adaptive mechanisms2: (1) redistribution of blood flow among organs via an increase in sympathetic adrenergic tone and central vascular contraction (this is responsible for a decreased perfusion in organs with low ERO2, such as the skin and splanchnic area, and a maintained perfusion in organs with high ERO2, such as heart and brain); and (2) capillary recruitment within organs responsible for peripheral vasodilation (opposite to central vasoconstriction).
Using Mixed Venous Oxygen Saturation to Assess Adequacy of Global Tissue Oxygenation
where CvO2 is mixed venous blood oxygen content. To some extent, is approximately equal to cardiac output × (SaO2 − SvO2) × Hb × 1.39, and SvO2 is approximately equal to SaO2 −
/(
× Hb × 1.39).
Four situations can be responsible for a decrease in SvO2: a decrease in SaO2 (hypoxemia), in Hb (anemia) or in cardiac output, or an increase in (like in exercise). At DO2 crit, SvO2 is about 40% (SvO2 crit) with an ERO2 of 60% and a SaO2 of 100%. This SvO2 crit has been identified in humans.3 It is important to emphasize that for the same decrease in CaO2 (induced by a decrease of Hb or SaO2), the decrease in SvO2 will be more pronounced if cardiac output cannot adapt. Hence, SvO2 represents adequacy of global flow to CaO2 decrease. A 40% SvO2 can be taken as an imbalance between arterial blood oxygen supply and tissue oxygen demand with evident risk of dysoxia. In the clinical setting, a decrease of SvO2 of 5% from its normal value (77%-65%) is representative of a significant fall in DO2 and/or an increase in oxygen demand (Figure 91-2). If initial probabilistic treatment (fluid resuscitation and/or low-dose inotropes and/or red blood cell transfusion) does not allow SvO2 to be restored to a minimal 65%, Hb, SaO2, and cardiac output should then be individually measured to introduce the appropriate treatment.
Assessing Global Flow
During circulatory shock, -to-DO2 dependency with a rise in blood lactate levels implies oxygen debt. Several authors have reported that oxygen debt is related to the likelihood of multiple organ failure and mortality in postoperative or polytrauma patients.4,5 Patients who survive multiple organ failure have been shown to have higher cardiac index, lower SVR, higher
, and higher SvO2 than nonsurvivors.6,7 Rixen and Siegel5 demonstrated that the degree of tissue oxygen debt is related to an enhanced inflammatory response, associated with an increased risk of acute respiratory distress syndrome and higher mortality rates.
Recent research has emphasized the potential interest of central venous oxygen saturation (ScvO2) for detecting global oxygenation impairment.7 Experimental studies reported that changes in SvO2 and ScvO2 closely reflect circulatory disturbances during periods of hypoxia, hemorrhage, and subsequent resuscitation (ScvO2 being approximately 5% higher than SvO2 in the critically ill). Fluctuations in these two parameters correlated relatively well, although absolute values differed.8 Finally, observational data found ScvO2 to be a useful parameter in detecting occult tissue hypoperfusion in both sepsis and cardiac failure.9,10 An important feature with ScvO2 monitoring is that ScvO2 can be continuously provided by central venous catheters equipped with optic fibers (e.g., PreSep oximetry catheter [Edwards Lifesciences, Irvine, California]). In initial resuscitation of circulatory shock, insertion of a central venous catheter is a standard, rapid, and easy approach, much easier than any other invasive hemodynamic monitoring, especially in patients who are not yet sedated, intubated, and ventilated.
In a landmark trial by Rivers et al., patients with severe sepsis and septic shock admitted to the emergency department were randomized to standard therapy (n = 133) or to early goal-directed therapy (n = 130) targeted to achieve a central ScvO2 of greater than 70%.11 Standard therapy included antibiotics, fluid resuscitation, and vasoactive drugs to achieve a central venous pressure between 8 and 12 mm Hg, MAP greater than 65 mm Hg, and urine output greater than 0.5 mL/kg/h. Patients in the early goal-directed therapy group, in addition to the standard goals, had to reach an ScvO2 of greater than 70% by optimizing fluid administration, hematocrit above 30%, and/or prescription of an inotrope (dobutamine < 20 µg/kg/min). Initial ScvO2 in both groups was quite low (49 ± 12%), confirming that severe sepsis is hypodynamic before any fluid resuscitation has started. This study demonstrated a significant reduction in hospital mortality: 30.5% in the early goal-directed therapy group compared with 46.5% in the standard therapy group (P = .009). An important point in this study is that 99.2% of patients receiving early goal-directed therapy achieved their hemodynamic goals within the first 6 hours, compared with 86% of those receiving standard therapy. From the first to the 72nd hour, total fluid loading was not different between the two groups (approximately 13,400 mL); in contrast, from the first to the seventh hour, the amount of fluid received was significantly larger in the early goal-directed therapy patients (approximately 5000 mL versus 3500 mL). In the follow-up period between the seventh and the 72nd hour, in patients receiving early goal-directed therapy, mean ScvO2 was higher (70.6 ± 10.7% versus 65.3 ± 11.4%; P = .02), mean arterial pH was higher (7.40 ± 0.12 versus 7.36 ± 0.12; P = .02), and lactate plasma levels were lower (3.0 ± 4.4 mmol/L versus 3.9 ± 4.4 mmol/L; P = .02), as was base excess (2.0 ± 6.6 mmol/L versus 5.1 ± 6.7 mmol/L; P = .02). The multiple organ failure score was significantly altered in patients receiving standard therapy when compared with early goal-directed therapy patients. This was the first study demonstrating that early identification of patients with sepsis, associated with early initiation of goal-directed therapy to achieve adequate tissue oxygenation by O2 delivery (ScvO2 monitoring), significantly improves mortality rates.11 This study was then supported by more than 10 following trials,12 and further multicentric prospective studies are under way.

Full access? Get Clinical Tree
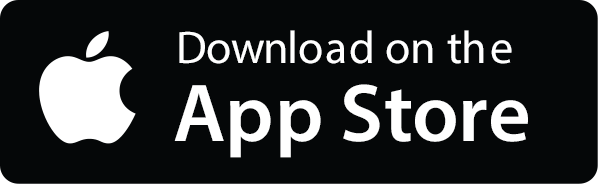
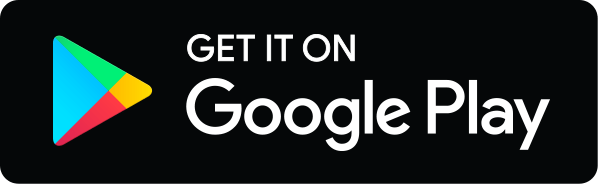