Respiratory Failure Part III: Asthma
J. Mark Madison
Richard S. Irwin
Asthma is an inflammatory disease of the airways characterized by reversible airway obstruction [1,2]. Inflammation causes airway obstruction by making airway smooth muscle more sensitive to contractile stimuli [3], by thickening the airway wall with edema and inflammatory cell infiltration, by stimulating glands to secrete mucus into the airway lumen, by damaging the airway epithelium [4], and by remodeling the architecture of the airways [5]. Typically, intermittent worsening or exacerbation of asthma is triggered by exposure to environmental factors such as inhaled allergens, irritants, or viral infections of the respiratory tract. These exacerbations represent acute or subacute episodes of increased airflow obstruction that may be mild to life threatening in severity. Assessment, management, and prevention of exacerbations of asthma, especially those leading to respiratory failure, are the critical challenges of caring for adult patients with asthma [6,7], the focus of this chapter.
Epidemiology
Worldwide, asthma ranks among the most common chronic diseases, with a prevalence ranging from a low of 0.7% in Macau, 6.7% in Japan, 10.9% in the United States, and a high of 18.4% in Scotland [8]. In general, asthma prevalence increases with urbanization and westernization of societies. In the United States, from 1980 to 1996, self-reported asthma prevalence increased 73.9% but then stabilized from 1997 to 2004 [9].
Asthma exacerbation rates vary by season with peaks in emergency room visits and hospitalizations coinciding with respiratory viral infections, especially rhinovirus, in late summer and early autumn [10]. In 2002, annual rates of hospitalization for asthma in the United States were 27 per 10,000 population-age 0–17 years and 13 per 10,000 population-age 18 and over. Although there remain important racial and gender differences in the rates of hospitalization, this represents an overall decline in hospitalizations from 1995 to 2002 and this suggests the possibility of better management and prevention of asthma exacerbations in ambulatory settings over these years [11].
In 2002 there were 4,261 deaths due to asthma in the United States indicating a death rate of 1.5 per 100,000 population of all ages [9]. Asthma mortality rates also have an annual cycle, but do not strictly parallel the cycle for exacerbations. In children, mortality peaks in the summer months, but, with increasing age, asthma mortality becomes more common in winter months [10]. In 2002, the death rate for ages 18 years and older was 1.9 deaths per 100,000 population, but it is notable that there are very large racial differences in the risk of death due to asthma. Blacks aged 25 to 34 years are six times more likely to die from asthma than whites of the same age group [9]. Deaths among patients hospitalized for asthma do account for one third of asthma related mortality, but potential differences in hospital care do not appear to account for the striking racial disparities and this suggests that prehospitalization factors are more important [12].
Pathophysiology
Pathology
Bronchial biopsy specimens of patients with asthma are pathologically abnormal [13,14,15], with collagen deposition beneath the epithelial basement membranes, mucosal infiltration by eosinophils and neutrophils, mast cell degranulation, and epithelial damage. These findings occur in both severe and mild asthma, suggesting that airway inflammation is of primary importance in the pathogenesis of asthma.
Asthma exacerbations show variable pathology, reflecting at least two recognized subtypes of exacerbation—slow onset and rapid onset. Slow onset exacerbations are the most common (approximately 80% of exacerbations) and the patient presents with more than 2 to 6 hours of symptoms—often days or weeks of symptoms [16,17,18]. This suggests that most such patients should have sufficient time to seek medical attention for worsening shortness of breath [19]. At autopsy, the lungs of patients who die of “slow-onset” asthma exacerbations are hyperinflated with thick tenacious mucus filling and obstructing the lumens of the airways [4]. Microscopically, there is an eosinophilic bronchitis, with pronounced areas of mucosal edema and desquamation of the epithelium. Typically, hypertrophy and hyperplasia of smooth muscle are present and the muscle appears contracted [4].
The patient with the rapid-onset type of exacerbation presents with severe symptoms that have rapidly progressed over 2 to 6 hours [16,17,18]. These rapid-onset exacerbations may represent 8% to 14% of asthma exacerbations in general and can be fatal, leading to death in only a few hours after symptom onset [16,18]. Pathologically, airway obstruction by mucus is not prominent, and there is a neutrophil, rather than eosinophil, predominance of inflammatory cells in the airway submucosa [20]. There are no specific clinical characteristics that reliably predict which patients are prone to these rapid-onset asthma exacerbations. However, patients with rapid onset asthma exacerbations may more commonly report sensitivity to nonsteroidal anti-inflammatory drugs (NSAIDs) [18].
Pathogenesis
Asthma is a disease or group of diseases with complex underlying genetics [21]. Why airway inflammation develops in the asthmatic patient is not understood entirely, but much evidence suggests an important role for Th2 cytokines [22]. Inhaled allergens, pollutants, smoke, and viral infections all may play a role in augmenting the baseline airway inflammation present in the asthmatic airway [1,23]. When these environmental
triggers interact with the asthmatic airway, the inflammation is intensified and the released mediators have potent effects on smooth muscle cell function, epithelium and microvascular integrity, neural function, and mucus gland secretion. All these factors contribute to increased narrowing of the asthmatic airway with smooth muscle contraction, mucus secretion, epithelial cell sloughing into the lumen, and edema and inflammatory cell infiltration of the airway wall. The resulting acute increase in airway obstruction is commonly referred to as an acute exacerbation of asthma.
triggers interact with the asthmatic airway, the inflammation is intensified and the released mediators have potent effects on smooth muscle cell function, epithelium and microvascular integrity, neural function, and mucus gland secretion. All these factors contribute to increased narrowing of the asthmatic airway with smooth muscle contraction, mucus secretion, epithelial cell sloughing into the lumen, and edema and inflammatory cell infiltration of the airway wall. The resulting acute increase in airway obstruction is commonly referred to as an acute exacerbation of asthma.
Physiology
The major physiologic consequences of airway obstruction are hypoxemia and increased work of breathing. Understanding these physiologic disturbances is important for management of severe exacerbations of asthma.
Narrowing the caliber of airway lumens causes hypoxemia by two mechanisms. First, increases in the resistance to flow in the conducting airways result in uneven distribution of ventilation to the alveoli. Hypoxic vasoconstriction of vessels that supply underventilated alveoli partially compensates for this uneven ventilation, but overall ventilation–perfusion ([V with dot above]/[Q with dot above]) ratios remain abnormal and are the principal cause of hypoxemia in asthma [24]. Consequently, even patients with severe exacerbations of asthma usually respond well to supplemental oxygen. A second, less common cause of hypoxemia in asthma is right-to-left shunt due to atelectasis of lung distal to airways that are completely occluded by mucus or due to interatrial shunt [25,26,27].
The second physiologic consequence of severe airway obstruction is increased work of breathing. During acute exacerbations, respiratory muscles must expend increased energy, generating large changes in pleural pressure to overcome high airway resistance [28]. The resulting discordance between respiratory effort and the change in thoracic volume also plays a role in the patient’s sensation of dyspnea and central drive to increase minute ventilation. The ensuing rapid respirations further increase the work of breathing and worsen air trapping behind narrowed airways that prematurely close during expiration. The dynamic hyperinflation of the lung itself leads to increased respiratory muscle energy costs because it restricts vital capacity to high thoracic volumes where alveolar dead space is increased, the respiratory muscles are at suboptimal mechanical advantage, and the lung is less compliant. All of these factors contribute to the enormous increase in the work of breathing. Thus, the respiratory muscles must expend more energy to achieve the same alveolar ventilation. Initially, the respiratory muscles may be able to exert the force needed to maintain alveolar ventilation but the muscles may fatigue if airway resistance increases rapidly, is sustained, or if there is inadequate oxygen delivery to theses muscles [29,30]. Dynamic hyperinflation due to severe airway obstruction also may impair cardiac performance by increasing afterload, decreasing venous return to the heart, and causing diastolic dysfunction [7,27].
Differential Diagnosis
Not all wheezing is due to asthma (Table 48.1). Obstruction of the airway at any level can produce wheezing and dyspnea that can be confused with asthma. For example, vocal cord dysfunction syndrome [31,32,33,34,35] is an extrathoracic cause of upper airway obstruction that can be confused with acute asthma. This diagnosis is suggested by the presence of stridor and wheeze in the absence of increased alveolar-arterial oxygen tension difference, extrathoracic variable obstruction on flow-volume loop, and observing paradoxic closure of vocal cords during inspiration on laryngoscopy.
Table 48.1 Differential Diagnosis of Wheezing | |
---|---|
|
Furthermore, many disease processes other than asthma can obstruct the lower airways to produce wheezing and dyspnea (Table 48.1). Systemic anaphylaxis can cause wheezing and should be considered in the differential diagnosis especially when respiratory symptoms have been of rapid onset and progress [36]. A diagnosis of anaphylaxis is suggested by acute-onset wheezing, stridor, urticaria, nausea, diarrhea, and hypotension (especially after insect bites, drug administration, or intravenous contrast). Exacerbations of chronic obstructive pulmonary disease (COPD) present similarly to acute asthma, but chronic bronchitis or emphysema, or both, can usually be distinguished from asthma historically. Pulmonary thromboembolism can masquerade as an exacerbation of asthma because the mediators released by platelets in thromboemboli sometimes cause bronchoconstriction and wheezing. However, hemoptysis, pleuritic pain, and pleural effusions rarely are seen in acute exacerbations of asthma.
Pulmonary edema, either cardiogenic or noncardiogenic, can obstruct small airways with mucosal swelling to produce acute wheezing. However, in these cases the clinical history, physical examination, and chest radiograph changes that show
vascular redistribution of blood flow and alveolar filling help exclude asthma as a diagnosis. Notably, however, acute, reversible left ventricular dysfunction has been described as a possible complication of severe exacerbations of asthma; the underlying mechanism for this is unclear [37]. Aspiration can present with acute dyspnea and wheezing. In this case, a history of impaired consciousness or inability to protect the airway suggests that the diagnosis and chest radiograph may show pulmonary infiltration.
vascular redistribution of blood flow and alveolar filling help exclude asthma as a diagnosis. Notably, however, acute, reversible left ventricular dysfunction has been described as a possible complication of severe exacerbations of asthma; the underlying mechanism for this is unclear [37]. Aspiration can present with acute dyspnea and wheezing. In this case, a history of impaired consciousness or inability to protect the airway suggests that the diagnosis and chest radiograph may show pulmonary infiltration.
Assessment
Physician failure to appreciate the severity of airway obstruction in acute asthma is not uncommon and contributes to mortality [1]. The cornerstone of evaluation of patients with asthma exacerbations is the objective measurement of airflow. However, because some patients, especially those with severe exacerbations, may be unable to perform the necessary testing maneuvers, the physician also must be adept at recognizing certain historical features and physical findings that strongly suggest high risk for severe airway obstruction.
History
Baseline pulmonary function tests that show persistent decreases in the forced expired volume of air in 1 second (FEV1), loss of lung elastic recoil, and hyperinflation at total lung capacity are associated with increased risk of near-fatal asthma [38]. A recent history of poorly controlled asthma (increases in dyspnea and wheezing, frequent nocturnal awakenings due to shortness of breath, increased use of beta-adrenergic rescue medications, increased diurnal variability in peak expiratory flow, and recent hospitalizations or emergency department visits) and any history of a prior near-fatal asthma exacerbation (prior admission to an intensive care unit or intubation for asthma) are the two most important predictors of a patient’s propensity for severe life-threatening asthma exacerbations [39,40,41,42,43,44]. Patient complaints of severe breathlessness or chest tightness or difficulty walking more than 100 feet (30.48 m) also suggest severe airway obstruction. Cigarette smoking also has been associated with higher in-hospital and posthospitalization mortality [43]. In general, patients are somewhat better judges of the severity of their airway obstruction during an attack of asthma than are physicians who elicit their history at the bedside [45]. However, the patient’s own assessment of airway obstruction should never be the exclusive means of assessing the severity of airway obstruction. Notably, patients with a history of severe asthma often have a blunted perception of dyspnea [46,47,48,49]. In assessing risk for fatal asthma, other important historical details include identification of current medications and coexisting illnesses, such as psychiatric disease, that interfere with medical follow-up and cardiopulmonary disease. A history of known coronary artery disease is important because the patient may be more sensitive to the stimulatory effects of β2-adrenergic agonists and to the cardiac complications of hypoxemia [50]. These patients may also be receiving β2-adrenergic antagonists that are making control of their asthma worse.
Physical Examination
Physical examination is important for excluding other causes of dyspnea (see Differential Diagnosis section) and assessing the degree of airway obstruction [44]. Tachycardia (greater than 120 beats per minute), tachypnea (greater than 30 breaths per minute), diaphoresis [51], bolt-upright posture in bed, pulsus paradoxus greater than 10 mm Hg, and accessory respiratory muscle use all should be regarded as signs of severe airway obstruction [52]. However, because the absence of these signs does not rule out severe obstruction, physical examination cannot be relied on exclusively to estimate the severity of airway obstruction. The amount of wheezing heard on auscultation of the chest is a notoriously poor method of assessing the severity of airway obstruction [53]. Cyanosis is a late, insensitive finding of severe hypoxemia. Abnormal thoracoabdominal motion (e.g., respiratory muscle alternans, abdominal paradox) and depressed mental status due to hypoxemia and hypercapnia are ominous indicators and can herald the necessity for mechanical ventilation [54].
Pulmonary Function Tests
To evaluate patients who are having an acute exacerbation of asthma, an objective measure of maximal expiratory airflow should be performed. An exception to this is the patient who is unable to perform a testing maneuver due to a severe, life-threatening exacerbation with obvious airway compromise and cyanosis [44]. Peak expiratory airflow rate (PEFR) and FEV1 are equally good bedside measures to quantify the degree of airway obstruction [55]. These tests are invaluable for the initial assessment and for following responses to therapy [44,56]. In general, a PEFR or FEV1 of less than 40% of baseline (either the predicted value or the patient’s best-known value) indicates severe obstruction and a severe exacerbation of asthma (Table 48.2).
Arterial Blood Gas Analysis
Analysis of arterial blood gases (ABGs) have a role in assessing and managing severe asthma exacerbations (see Chapter 11) and should be performed for suspected hypoventilation, severe respiratory distress, or when spirometric test results are less than 25% predicted [44]. Also, any patient who fails to respond to the first 30 to 60 minutes of intensive bronchodilator therapy should have an ABG analysis performed. Although ABG values are not predictive of overall patient outcome [55], there is some correlation between hypoxemia and hypercapnia and the degree of airway obstruction measured by FEV1 [57]. A partial pressure of arterial oxygen (PaO2) less than 60 mm Hg or a pulse oximeter oxygen saturation value less than 90% on room air should be regarded as additional evidence of severe airway obstruction. Therefore, although ABG analysis is not recommended as routine in the initial evaluation of asthma, it should be done for the evaluation of severe cases. One study found that the frequency of ABG analysis in cases of severe asthma actually decreased from 1997 to 2000, a trend needing improvement [58].
Table 48.2 Objective Assessment of Airway Obstruction After Initial Intensive Therapy | ||||||||||
---|---|---|---|---|---|---|---|---|---|---|
|
Understanding the expected changes in the partial pressure of arterial carbon dioxide (PaCO2) during an asthma exacerbation is important for recognition of a rapidly deteriorating course. With modest airway obstruction, the patient’s mild dyspnea stimulates an increase in minute ventilation that meets or exceeds the level required to maintain normal alveolar ventilation. Thus, patients with modest obstruction have a normal or slightly below normal PaCO2. As airway obstruction worsens, dyspnea becomes more severe and the central nervous system drive to increase minute ventilation becomes intense. Typically, the increase in minute ventilation exceeds the level required to maintain constant alveolar ventilation; consequently, patients with moderate-to-severe obstruction have lower than normal PaCO2 and respiratory alkalosis. As the airway obstruction becomes more severe and prolonged, high minute ventilation can no longer be maintained by the respiratory musculature and alveolar ventilation decreases. As a result, the PaCO2 rises toward normal and then continues to climb, resulting in hypercapnia and respiratory acidosis. Thus, a normal or high PaCO2 (greater than 40 mm Hg) during a severe exacerbation of asthma is a potentially ominous finding, often signifying the impending need for mechanical ventilation. Any coexisting conditions (malnutrition, advanced age) or medications (sedatives) that weaken respiratory muscle function or depress respiratory drive should be expected to accelerate the onset of hypercapnic ventilatory failure during acute exacerbations of asthma.
Other Laboratory Studies
For acute exacerbations of asthma, routine chest radiographs reveal few abnormalities other than hyperinflation [59]. However, although not recommended for routine assessment, for severe exacerbations chest radiography can be helpful when there is clinical suspicion of other causes of dyspnea and wheezing (see Differential Diagnosis section) or complications of severe airway obstruction [44]. Chest radiographs should be examined for evidence of enlarged cardiac silhouette, upper lung zone redistribution of blood flow, pleural effusions, and alveolar or interstitial infiltrates because any one of these findings suggests a diagnosis other than or in addition to acute asthma. In addition, chest radiography allows the early detection of common complications of severe airway obstruction, including pneumothorax, pneumomediastinum, and atelectasis. Also, lung infiltrates on chest radiographs can be compatible with a diagnosis of asthma complicated by either allergic bronchopulmonary aspergillosis or Churg–Strauss syndrome.
In the elderly, in patients with severe hypoxemia, and in individuals with suspected cardiac ischemia or arrhythmia, an electrocardiogram should be performed. Sinus tachycardia is common during acute exacerbations of asthma, but less common and transient findings include right-axis deviation, right ventricular hypertrophy and strain, P pulmonale, ST- and T-wave abnormalities, right bundle-branch block, and ventricular ectopic beats [60].
Therapeutic Agents
Optimal management of an acute exacerbation of asthma begins with a careful assessment of the degree of airway obstruction. This initial assessment and repeated objective measures of airway obstruction guide treatment that combines supportive measures, bronchodilator therapy, and anti-inflammatory therapy (Table 48.3).
Because the dominant causes of airway obstruction during an acute exacerbation of asthma are the result of airway inflammation, the cornerstone of treatment is anti-inflammatory therapy with systemic corticosteroids [61]. Because corticosteroids take at least 4 to 6 hours to begin to have a beneficial effect and the inflammatory causes of airway obstruction may take days to resolve, the medical challenge is to support patients until the inflammatory processes have responded to corticosteroids.
Table 48.3 Treatment of Severe Acute Exacerbations of Asthma | |
---|---|
|
β2-Adrenergic agonists relieve airway obstruction due to airway smooth muscle contraction, and this is an important therapeutic maneuver in initial treatment. Although these bronchodilators relieve only one component of the airway obstruction during severe exacerbations of asthma, even small improvements in airflow can lead to important clinical benefits in the acute setting. Of the available bronchodilators, β2-adrenergic agonists are the most effective and rapidly acting and, therefore, most useful during that critical time before the onset of corticosteroid action [62]. Other measures that support the patient until the inflammatory processes in the airways have resolved include supplemental oxygen, judicious fluid administration, and, when indicated, mechanical ventilation.
β2-Adrenergic Agonists
β2-Adrenergic agonists bind to β2-adrenergic receptors on airway smooth muscle cells and cause relaxation of the muscle cell. Although the primary cellular target of β2-adrenergic agonists is airway smooth muscle, other cell types in the airways also express β2-adrenergic receptors that may regulate mediator release by mast cells, epithelial cells, and nerves.
There are two general classes of β2-adrenergic agonists. Short-acting β2-adrenergic agonists (SABA) have bronchodilatory effects that last for 3 to 5 hours. They include
epinephrine, isoproterenol, terbutaline, metaproterenol, albuterol, and fenoterol. These short-acting agents have an onset of action less than 5 minutes and are the mainstay of bronchodilator therapy for acute asthma. These agents differ in their selectivity for β2-adrenergic receptors, the rank order of selectivity being epinephrine < isoproterenol < metaproterenol < fenoterol, terbutaline, and albuterol [63]. However, all of these agents have approximately equal efficacy in the treatment of asthma. Another class of drugs, the long-acting β2-adrenergic agonists (LABA), have bronchodilatory effects for at least 12 hours, but these agents are not currently recommended in the treatment of acute exacerbations [1,2,44]. There has been significant controversy on whether chronic use of these long-acting agents predisposes patients to increased severe, life-threatening, or fatal asthma exacerbations [64].
epinephrine, isoproterenol, terbutaline, metaproterenol, albuterol, and fenoterol. These short-acting agents have an onset of action less than 5 minutes and are the mainstay of bronchodilator therapy for acute asthma. These agents differ in their selectivity for β2-adrenergic receptors, the rank order of selectivity being epinephrine < isoproterenol < metaproterenol < fenoterol, terbutaline, and albuterol [63]. However, all of these agents have approximately equal efficacy in the treatment of asthma. Another class of drugs, the long-acting β2-adrenergic agonists (LABA), have bronchodilatory effects for at least 12 hours, but these agents are not currently recommended in the treatment of acute exacerbations [1,2,44]. There has been significant controversy on whether chronic use of these long-acting agents predisposes patients to increased severe, life-threatening, or fatal asthma exacerbations [64].
Among the short-acting β2-adrenergic agonists, a single-isomer preparation (i.e., R-albuterol or levalbuterol) is available. The potential advantage of this preparation is that the S-enantiomer present in racemic albuterol, does not contribute to bronchodilation and might have deleterious effects in the airways. However, although some studies of levalbuterol (R-albuterol) in the emergency department setting have suggested that levalbuterol is a more efficacious bronchodilator than racemic preparations, there have been no large, randomized, double-blind and controlled trials in adults to confirm these findings [65,66,67].
The major side effects of β2-adrenergic agonists during the treatment of severe asthma exacerbations are tremor, cardiac stimulation, and hypokalemia [68]. Case reports have associated lactic acidosis with the use of β2-adrenergic agonists as well [69]. These side effects are potentially serious, especially in the elderly, who frequently have underlying cardiac disease. Cardiac toxicity can be minimized by using agonists with high β2-adrenergic receptor selectivity, by avoiding systemic administration of β2-adrenergic agonists, and by maintaining adequate oxygenation [50,70]. β2-Adrenergic agonists can be administered to patients by inhaled, subcutaneous, or intravenous routes. Numerous studies have shown that the bronchodilator effects of inhaled β2-adrenergic agonists are rapid in onset and equal to the effect achieved by systemic delivery [71]. Because the inhaled route allows administration of comparatively small doses directly to the airways with minimal systemic toxicity, this route is almost always preferable to systemic delivery [1,2].
Several options exist for the delivery of inhaled β2-adrenergic agonists (see Chapter 62). A small-volume nebulizer is widely used. However, studies have shown that metered-dose inhalers (MDIs) equipped with spacer devices are as effective as small-volume nebulizers in the treatment of acute asthma, although some patients may have difficulty coordinating MDI use, especially during an acute exacerbation with severe respiratory distress [1,72,73]. Frequent, multiple inhalations of the medication may allow for progressively deeper penetration of the drug into peripheral airways [74]. In fact, continuous administration by nebulizer may be more effective in severely obstructed patients [75,76]. For administration of inhaled albuterol in the treatment of severe exacerbations of asthma, National Institutes of Health guidelines recommend treatment with MDI (90 μg per puff; four to eight puffs every 20 minutes up to 4 hours, then every 1 to 4 hours as needed) or nebulizer treatments, either intermittent (2.5 to 5.0 mg every 20 minutes for 3 doses, then every 1 to 4 hours as needed) or continuous (10 to 15 mg per hour) [1] (see Management section).
Intermittent positive-pressure breathing devices to deliver aerosols were once popular but are not used today because many patients with severe asthma cannot tolerate the device and because the devices are no more effective than small-volume nebulizers [77]. Furthermore, the risk of barotrauma is significantly increased with intermittent positive-pressure breathing devices, and pneumothorax resulting in death has been reported [78].
Because of its lower density than oxygen, heliox-powered nebulizer treatments have the potential to improve penetration of aerosols into the lungs. Adult patients with severe asthma exacerbations had greater improvements in peak expiratory flow rates and dyspnea scores when albuterol was delivered using heliox, rather than oxygen, driven nebulization [79,80]. Current National Institute of Health Guidelines suggest that heliox-driven albuterol nebulization be considered for patients with life-threatening exacerbations or for those with severe exacerbations even after 1 hour of intensive conventional therapy [1].
Theoretically, systemic administration of beta-adrenergic agonists could deliver drugs via the bloodstream to obstructed airways that are poorly accessible to inhaled aerosols. However, this theoretical advantage has not been supported by most studies [71]. Subcutaneous epinephrine (adults, 0.3 mL of a 1 to 1,000 solution every 20 minutes for three doses) was a traditional therapy for acute asthma in emergency departments, but it is not more effective than aerosol delivery of β2-adrenergic agonists [81]. A major concern with the use of subcutaneous epinephrine in adults has been cardiac toxicity [82]. More selective β2-adrenergic agonists, such as terbutaline, are available for subcutaneous use, but cardiac toxicity in elderly individuals is still a significant concern even with these more selective agents. Formerly, intravenous isoproterenol (0.05 to 1.50 μg per kg per minute) was often used to treat severe exacerbations of asthma [83]. However, intravenous delivery of β2-adrenergic agonists is no longer recommended for the routine treatment of even severe exacerbations of asthma [1,2]. No convincing evidence has shown intravenous administration to be superior to inhaled delivery of β2-adrenergic agonists. The lack of enhanced efficacy and the potential cardiac toxicity of intravenous β2-adrenergic agonists have led most authorities to reserve intravenous delivery for those rare patients who continue to deteriorate on mechanical ventilation despite maximal routine therapy with inhaled β2-adrenergic agonists [83]. Intravenous β2-adrenergic agonists should be used only in closely monitored adults because myocardial ischemia can occur [84]. It is important to emphasize again that intravenous β2-adrenergic agonists are not recommended in current NIH guidelines and are unlikely to be any more effective than inhaled β2-adrenergic agonists such as albuterol [1].
Cholinergic Antagonists
The muscarinic cholinergic antagonists (e.g., atropine, ipratropium and tiotropium) are less effective and more slowly acting bronchodilators than β2-adrenergic agonists [85,86,87]. In general, these agents should not be used as the sole bronchodilator therapy for acute asthma. Exceptions may be bronchospasm induced by acetylcholinesterase inhibitors or β2-adrenergic antagonists and patients with severe cardiac disease who are unable to tolerate β2-adrenergic agonists.
However, inhaled cholinergic antagonists have a low incidence of side effects and are a recommended adjunct to β2-adrenergic agonists in the initial emergency department treatment of severe exacerbations of asthma [1,88]. Because even small improvements in airflow could prove clinically significant in the severely obstructed and deteriorating patient, it is recommended that ipratropium be routinely added to β2-adrenergic agonist therapy during the initial treatment of severe asthma exacerbations in the emergency department [1] (see Management section). However, although comparable trials for adults do not exist, controlled trials in children have not shown a benefit of continuing ipratropium treatment once the patient is hospitalized [89,90]. Therefore, inhaled ipratropium bromide currently is not recommended for hospitalized
patients with severe exacerbations of asthma [1]. The long-acting anticholinergic, tiotropium, has a role in treating outpatients with difficult to control asthma, but whether it has a role in treating hospitalized patients with acute exacerbations of asthma is not yet known [91].
patients with severe exacerbations of asthma [1]. The long-acting anticholinergic, tiotropium, has a role in treating outpatients with difficult to control asthma, but whether it has a role in treating hospitalized patients with acute exacerbations of asthma is not yet known [91].

Full access? Get Clinical Tree
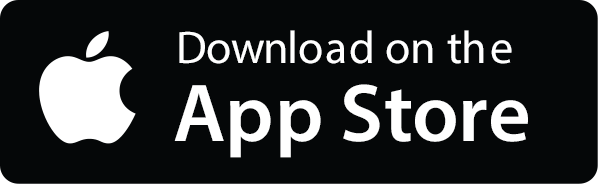
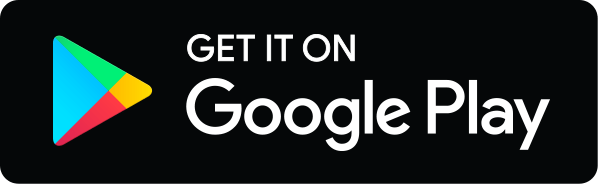