Chapter 6 Respiratory assessment and disease
David Lynes
SUMMARY
This chapter will describe:
• basic anatomy and physiology of the respiratory system
• applied physiology of common respiratory disease
• blood gas analysis and implication for perioperative optimisation
• non-pharma and pharmacological approach to respiratory optimisation.
INTRODUCTION
Patients with chronic respiratory problems frequently have co-morbidities that may increase the likelihood of their needing surgery. Moreover, due to the prevalence of respiratory disorders such as COPD it is often necessary to anaesthetise patients with respiratory problems and this involves a high risk of intra- and postoperative respiratory complications.1 The perioperative clinician should be aware that, although the effects of general anaesthesia may be minor, they may nevertheless tip a patient with a respiratory condition and hence limited respiratory reserve into respiratory failure. Problems might include laryngeal or bronchial spasm due to intubation and a reduction in the ability to clear secretions due to anaesthetic agents. Excess intravenous fluids can cause pulmonary oedema in patients with cardiac failure, and some anaesthetics and drugs may depress respiratory drive.2
Care of the patient with respiratory conditions is therefore an essential aspect of perioperative care and this chapter describes common respiratory diseases such as COPD and asthma and discusses salient aspects of current management regimes. Pivotal aspects of perioperative management, including preoperative assessment and postoperative care, are also considered.
Respiratory anatomy and physiology: an introduction
The respiratory system can be divided into a number of sections. These include the upper respiratory tract and the lower respiratory tract; the chest wall and respiratory muscles such as intercostal and diaphragm, and the cardiopulmonary circulation. A succinct description of respiratory anatomy and physiology is provided here and in other parts of this chapter.
Figure 6.1 The respiratory system
The upper respiratory tract comprises the nose, the pharynx and the larynx. Its functions include warming, filtering and moistening inhaled air.
The lower respiratory tract comprises conducting airways such as the larger trachea and bronchi, and smaller bronchioles. It also includes respiratory airways such as alveolar ducts, and the alveoli. Gaseous exchange, which involves oxygen passing from the lungs into the blood and carbon dioxide passing from the blood to the lungs, occurs at the respiratory airways and the alveoli (see Figure 6.1).
The conducting airways are made from smooth muscle and cartilage and they are lined with ciliated epithelium. Ciliated epithelium is a layer of cells with a brush border of cilia which are microscopic hair-like structures. These are interspersed with goblet cells which secrete mucus. If particles of dust or other airborne pollutants get past the upper respiratory tract into the lower respiratory tract, the conducting airways can constrict, which means that airflow through the airways becomes spiral rather than laminar and the dust is deposited onto the ciliated epithelium. Mucus traps the particles, and movement of the cilia ensures that trapped particles are removed from the lungs via the ‘muco-ciliary escalator’. The airways therefore play an important role in preventing particles reaching the respiratory airways and alveoli, which do not have goblet cells or cilia. If particles do reach the alveoli, cells called macrophages detect them. Macrophages engulf and destroy micro-organisms, and can also trigger a reaction in the immune system which helps protect the lungs from future invasions of micro-organisms.
The smaller respiratory airways do not have cartilage and therefore rely on attachments to the alveoli to keep them open. This is a bit like having guy ropes on a tent to keep it up; the attachments create a radial traction on the smaller airways that keep them open. Loss of attachments, such as that which occurs in emphysema, leads to the collapse of the small airways.
The alveoli are composed primarily of a single layer of cells called type 1 pneumocytes. Type 2 pneumocytes also form part of the alveolar walls. These produce a substance called surfactant which reduces the risk of alveolar collapse during expiration. The function of the alveoli is to enable gas exchange between the air and pulmonary circulation. There are a large number of microscopic spherical alveoli, which means that there is a total surface area of between 50 and 100 square metres of membrane available for gaseous exchange in normal adults. Pulmonary venules are wrapped around the alveoli, which permit transfer of gas to and from the blood.
The chest wall is composed of twelve pairs of ribs separated by intercostal muscles, and the diaphragm below. A layer of membrane called the parietal pleura lines the chest wall inner surface, and the outer surface of the lung is lined by the visceral pleura. These membranes are in contact with each other but are not attached. There is a thin layer of pleural fluid between the two membranes.
Inspiration of air is achieved by contraction of the diaphragm, which increases the size of the thoracic cavity. Contraction of intercostal muscles also increases thoracic volume by moving the ribs upwards and outwards. This causes a fall in pressure within the lungs, and this means that air travels from the mouth to the alveoli.
Expiration is largely a passive process. It usually occurs when the intercostal muscles and the diaphragm are relaxed, although expiration can be forced with the help of muscles such as abdominal muscles.
Common respiratory disorders: asthma and COPD
Both asthma and COPD are ‘obstructive’ disorders, which means that the diameters of airways become smaller so that it becomes difficult or even impossible to breathe. A simple analogy is that of breathing through a drinking straw rather than a hosepipe; it takes longer to breathe in and out. This may mean that it is possible to sit comfortably, but when walking a distance or up stairs it may be necessary to stop to catch one’s breath because it is not possible to breathe in sufficient oxygen to meet the additional demand due to exercise. If a smaller diameter drinking straw is used, it is possible that one may be extremely uncomfortable at rest; indeed it may take so long to breathe out that there may not be enough time for the lungs to empty before it is time to breathe in, leading to ‘dynamic pulmonary hyperinflation’.
Obstructive disorders are just one category of respiratory diseases; another is ‘restrictive’. In restrictive disorders the diameter of airways may not be affected, but the volume of the lungs is reduced because the patient cannot breathe in completely. Restrictive disorders include fibrosing disorders such as silicosis and fibrosing alveolitis, and bony disorders such as kyphosis. They can also include muscular weakness.
The respiratory investigation ‘spirometry’ is essential when diagnosing an obstructive or restrictive disorder and it is also an important preoperative investigation. An understanding of spirometry is therefore prerequisite to understanding asthma, COPD, and perioperative care of the patient with a respiratory condition.
Spirometry involves a patient breathing in as deeply as they can, blowing into a spirometer via a mouthpiece as forcibly as they can, and exhaling completely. Spirometry measures a number of variables, but perhaps the most important of these are the Forced Vital Capacity (FVC), which is the volume of air that can be exhaled from the lungs following full inspiration during a forced manoeuvre, and the Forced Expiratory Volume at one second (FEV1), which is the volume of air expired in the first second of the FVC manoeuvre.
Results from spirometry can be displayed in a number of ways, but one of the most useful methods of displaying results is graphically on a Volume Time Curve (see Figure 6.2).
Figure 6.2 Volume Time Curve demonstrating a normal pattern
As the patient begins to exhale rapidly the graph rises sharply upwards and then curves smoothly and flattens as the exhalation slows until all air is exhaled from the lungs. FVC and the FEV1 can be read directly from the graph.
Clearly if an individual has an obstructive disorder it will take them longer to exhale during the FVC manoeuvre (imagine trying to exhale rapidly through a narrow drinking straw). Whilst the FVC may not be affected, the FEV1 will certainly be reduced. In order to diagnose an obstructive disorder, the FEV1 is considered as a percentage of the FVC. This FEV1/FVC ratio is sometimes referred to as the ‘forced expiratory ratio’ or FEV1 %. This ratio defines the presence or absence of obstruction in the airways. A ratio of less than 70% is deemed to indicate obstructive lung disease.3
Figure 6.3 Volume Time Curve demonstrating an obstructive pattern in comparison to a normal curve
Figure 6.4 Volume Time Curve demonstrating a restrictive pattern in comparison to a normal curve
Figure 6.3 shows a typical trace of an obstructive pattern, which one might expect with either asthma or COPD. This presents as a reduction in FEV1 from normal. Clearly the ratio of the FEV1 to FVC is also reduced, demonstrating airflow obstruction.
Spirometry will also detect restrictive lung disorders, as shown by Figure 6.4. Restrictive disorders prevent full expansion of the lungs and may be due to changes in the chest wall, lung tissue and pleura. It is important to note that, unlike obstructive disorders, restrictive lung disorders will not necessarily reduce the diameter of airways, and the shape of the curve is therefore similar to a ‘normal’ curve.
The reduction in lung volume caused by restrictive disorders will mean that the FVC and FEV1 are reduced compared to normal (see Figure 6.4). The forced expiratory ratio, however, is normal (above 70%) or high. This is because, although the vital capacity is low, the airflow is not reduced because the size of the airways remains normal. Clearly it is important to calculate the FEV1/FVC ratio when diagnosing an obstructive disorder, and not simply rely on an FEV1 measurement.
An example of the interpretation of spirometry: COPD
COPD involves progressive airflow obstruction. The NICE guidelines3 indicate that COPD disease is characterised by airflow obstruction and that the airflow obstruction is usually progressive, not fully reversible and does not change markedly over several months. Unlike asthma, once COPD is established, the changes are irreversible and the disease continues to progress so long as the patient continues to smoke. In healthy non-smoking adults, FEV1 will decline by an average of 30ml per year. However, in the subgroup of smokers who develop COPD, FEV1 decline is an average of 70ml per year. Some smokers will decline faster than this.4
Fig 6.5 FEV1 decline Based on the work of Fletcher and Peto et al (1976)
It is vital to ensure that the spirometry recording is accurate prior to interpretation of results. There must be a minimum of three technically acceptable recordings. The curve should be smooth, convex and free from irregularities that may, for example, be caused by coughing during the forced manoeuvre. It should rise steeply upwards and plateau for at least 2 seconds. Reproducibility is demonstrated by comparing each FVC attempt. At least two should be within 100ml and 5% of each other.
Spirometry interpretation
Step 1: is obstruction present?
As discussed, this information is acquired from the FEV1/FVC ratio, which is less than 70% in obstructive lung diseases, such as COPD. If the ratio is greater than 70%, no obstruction has been demonstrated and the individual may not have COPD.
The calculation for this ratio is as follows: | ![]() |
Step 2: What is the level of FVC and FEV1?
The values obtained from spirometry can be compared to predicted values for the individual. These are based on the patient’s height, age, gender and ethnicity. Some spirometers will do this following input of appropriate data prior to the test. The measured values are compared to the predicted value and are expressed as a percentage.
According to NICE guidelines,3 a normal FVC is defined as being greater than 80% of the predicted value. If the FVC is low, that is less than 80% of the predicted value, this indicates small lung volumes which may be due to a restrictive lung disease. If detected, this would indicate the need for further investigation.
The calculation for percentage of predicted for FVC is as follows: | ![]() |
Once it has been established that a patient has an obstructive curve, defined as an FEV1 /FVC ratio below 70%, FEV1 can be compared to predicted values in order to calculate the severity of COPD. A normal FEV1, is defined as being greater than 80% of the predicted value3 although some guidelines suggest that a patient can still have COPD if FEV1 is above 80% predicted, if the FEV1/FVC ratio is also reduced.5
The calculation for percentage of predicted for FEV1 is: | ![]() |
For patients with COPD, the severity of the disease is determined by comparing the FEV1 to the predicted value. Guidelines for the diagnosis and classification of COPD exist and vary; for example, severity classified by NICE3 is outlined in Table 6.1.
Table 6.1 Classification of severity of COPD according to NICE (2004)
MILD COPD | FEV1 50–80% |
MODERATE COPD | FEV1 30–49% |
SEVERE COPD | FEV1 <30% |
It can be seen that spirometry is a pivotal investigation, and it will be discussed in relation to perioperative care later in this section.
Asthma and COPD have many similarities, including the fact that both diseases are obstructive and therefore limit expiratory flow; indeed asthma and COPD can coexist or overlap. However, it is important to understand that they are different disorders, and whilst they can coexist, their treatment, including aspects of pharmacological management, is different.
Whilst airway inflammation plays the central role in the pathogenesis of both diseases, the inflammation in COPD is different to that seen in asthma; in COPD it is driven by neutrophils whereas inflammation in asthma is driven by eosinophils.6 This has important implications for pharmacological management and prognosis, which are clearly different in asthma and COPD, not least in that COPD inevitably leads to death and no current treatment can halt disease progression, whereas deterioration in asthma can be prevented and the condition can be managed for life.
The asthma inflammatory process involves the airways becoming infiltrated with inflammatory cells, and these cells are activated, meaning that they produce and contain chemicals that cause inflammation. The airflow obstruction seen in asthma is usually at least partially and often fully reversible, but if a patient ignores the symptoms or does not treat the inflammation the airways may become damaged and some changes in airway structure and function may become irreversible. For example, the basement membrane, which is the layer of tissue immediately below the lining layer of the airway wall, can become thickened. This results in the airways becoming narrowed and stiff, which can mean that the airways become less responsive to bronchodilator drugs.
Most people with asthma develop inflamed airways due to an allergic response. This means that their airways become inflamed due to an external stimulus which triggers the asthmatic response, and indeed asthmatic attacks. These triggers include allergens such grass pollen, food and drink, dust, and animal fur. However, other triggers may not be allergens. For example, exercise, respiratory viruses, psychological stress and pollution may cause asthma attacks in some people.
The inflammatory process and pathological changes in COPD are quite different from those in asthma. A pollutant, usually smoking, irritates the bronchiolar wall. The body responds by producing additional mucus, and the patient can develop chronic bronchitis. Smoke can also damage the respiratory bronchioles and the alveoli by attracting neutrophils which release enzymes called proteases. These enzymes can damage respiratory bronchioles and alveoli in susceptible individuals, which in the long term can result in the destruction of alveoli seen in emphysema. However, some smokers will not develop COPD, and it is probable that this is because they have an efficient protective mechanism against the enzymes released by white cells. Alpha 1 antitrypsin is an ‘antiprotease’ which is an example of a protective mechanism. A deficiency in alpha 1 antitrypsin will result in the early development of COPD, and may result in the development of COPD in a non-smoker. Alpha 1 antitrypsin deficiency is a rare condition which is probably responsible for about 1% of cases of COPD.
COPD affects different people in different ways, but there are some pathological processes that are common to most patients. In the large airways the mucous glands increase in size and number, which results in increased mucus production. The epithelium becomes chronically inflamed, and breakdown of the integrity of the epithelium is often seen. The viscosity of mucus increases and the cilia that line the airways become destroyed. Because of this the lungs’ ability to remove mucus is impaired, as the mucociliary escalator becomes inefficient. This is called chronic bronchitis.
In the medium airways the airway smooth muscle becomes thickened and excessively contracted, which reduces the diameter of the airway. The small airways can become inflamed, oedematous, and infiltrated with cells such as macrophages and neutrophils. Smoking causes the neutrophils to release various enzymes such as proteases, and these digest and damage the alveoli. Inflammation also occurs within the walls of the distal bronchi and bronchioles, and this, together with the impact of repeated infections, leads to irreversible structural damage to the walls and sub-mucosa of the small airways.
Fig 6.6 Pathological changes in the airways
This process can result in the destruction of alveoli and when alveolar walls are damaged they can coalesce; this means that some of the smaller alveolar sacs merge to become larger ones. Instead of small alveolar sacs that are highly elastic, the alveoli coalesce into large inelastic sacs. This is called emphysema and is seen in Figure 6.7 (page 110).
If alveoli continue to merge they can form sacs that are larger than 1cm in diameter. These larger sacs are called bullae. This coalescence results in a reduction of the surface area of alveolar membrane, which results in impaired gaseous exchange. The smaller airways that supply air to alveoli have microscopically thin walls, and their shape and patency is maintained by attachments which act like guy ropes applying radial traction. Emphysema also causes a reduction in radial traction, which means that the small airways are not supported so they tend to close and collapse, especially during expiration. This will lead to air trapping, to increased work of breathing that is less efficient, and to areas of the lung not being ventilated. Hence gas exchange is further impaired. This can cause a disturbance in the matching of ventilation to perfusion.
Fig 6.7 Pathological changes in the alveoli and small airways
COPD is a disorder that affects the whole body including the heart, kidneys and muscles. It also has cognitive and emotional aspects such as panic and anxiety which may directly contribute to the sensation of dyspnoea.
Importantly, as far as perioperative management is concerned, significant airflow obstruction may have developed before the COPD patient is aware of it. Indeed a substantial degree of lung damage can take place before any clinical symptoms become apparent. Many patients with COPD may have a 50% reduction in FEV1 before they present to a doctor. This is probably because we have more alveoli than are needed for gas exchange; therefore we can afford to lose a substantial percentage before dyspnoea becomes apparent.
Fig 6.8 Lung damage can occur before symptoms become obvious
This means that a patient may present ‘normally’ and may not believe that they have a respiratory disease, but their FEV1 may be significantly reduced, which has implications for surgery.
With increased disease severity, alveoli become hypoventilated and the patient becomes hypoxic. The patient will develop respiratory failure, and will need long-term oxygen therapy (LTOT). LTOT can double survival if given appropriately.7,8
Pharmacological management of asthma and COPD
The evidence base for the pharmacological management of asthma and COPD is rapidly changing, and today’s good practice soon becomes dated. Up-to-date guidelines can be downloaded from the British Thoracic Society (http://www.brit-thoracic.org.uk).
Drug therapy for asthma includes inhaled bronchodilators and anti-inflammatory agents. Bronchodilators relieve the symptoms of asthma, thus patients sometimes call them relievers. Inhaled bronchodilators can be short or long acting; short acting typically lasting from 4 to 6 hours, and long acting 12 hours. Anti-inflammatory drugs do not directly cause bronchodilation so do not give immediate relief, but they can modify the underlying inflammation. Patients sometimes call anti-inflammatory drugs ‘preventers’ or ‘controllers’. Anti-inflammatory drugs include inhaled and oral corticosteroids, and other drugs such as leukotriene receptor antagonists.
Asthma is variable, and an asthmatic can have severe symptoms at times, yet at other times can have normal respiratory function. Pharmacological therapy in asthma is therefore ‘stepped up’ or ‘stepped down’ depending on the severity of the patient’s asthma, which can change. Asthmatic patients therefore need regular review, the aim being to control the disorder and prevent deterioration. Pharmacological therapy is adjunct to other aspects of management, such as allergen avoidance.
The management of COPD is different. Unfortunately treatment does not slow the underlying disease progression or slow decline in lung function. Bronchodilators can help increase exercise capacity and reduce breathlessness. The classes of bronchodilators used for COPD include inhaled beta-2 agonists, inhaled anti-muscarinics, and oral xanthine derivatives. These can be taken in short or long acting forms, they can be combined with each other, and in some circumstances can be combined with other drugs such as inhaled corticosteroids. Generally patients start off with the ‘weaker’ and less expensive bronchodilators, and progress to the stronger drugs in isolation and in combination with others as their disease progresses.
Pharmacological therapy can also reduce the frequency of exacerbations that a patient is likely to experience. Inhaled corticosteroids are particularly effective in this respect,9,10,11 and exacerbation frequency can be reduced even further if these are combined with long-acting bronchodilators.12,13,14 Systematic reviews suggest that mucolytics can also reduce exacerbations in patients with chronic bronchitis and COPD.15 NICE3 therefore recommend mucolytic therapy be considered in patients with a chronic cough productive of sputum.
Management of exacerbations
A patient with asthma may occasionally exacerbate, and patients with COPD exacerbate with increasing frequency and severity as their condition deteriorates.
When the asthmatic patient is in hospital, the treatment of an uncontrolled attack usually involves the use of a beta-2 agonist given by nebuliser, and if the peak flow reading is less than 75% of the predicted value then a course of oral corticosteroids is usually prescribed for at least one week. High flow oxygen is administered (usually 40% via a facemask).
The features of an acute severe attack of asthma include the inability to complete a sentence without having to draw a second breath, a pulse rate greater than 110 per minute, a respiratory rate of greater than 25 breaths per minute, and a peak flow of less than 50% predicted or best value. Thus if a patient has a silent chest, cyanosis, bradycardia and an FEV1 of less than 33% predicted the attack is deemed to be life threatening and management should be in a critical care or specialised respiratory unit. The use of high flow oxygen therapy and intravenous hydrocortisone may be required along with other treatments including intravenous magnesium and intravenous aminophylline along with local guidelines in discussion with senior medical staff.
Acute exacerbations of COPD which require hospitalisation can be difficult to treat, and require a skilled and knowledgeable practitioner. For example, it is not sufficient to give a fixed percentage of oxygen; the patient needs enough to correct hypoxia, yet too much can cause death by respiratory failure so careful titration of oxygen is necessary.
The signs of an exacerbation of COPD include a high respiratory rate, shallow breathing, use of accessory muscles and tachycardia. The patient may be centrally cyanosed, and may show signs of carbon dioxide retention such as confusion and agitation, a bounding pulse, vasodilation (warm, flushed peripheries) and a flapping tremor of the hands.
The treatment of an exacerbation of COPD includes antibiotics, oral corticosteroids, bronchodilators and oxygen therapy. It may also include non-invasive ventilation. The use of antibiotics is contentious, as many exacerbations have viral or non-infective causes. Ram et al.16 concluded that antibiotics should be used for those who have increased cough and sputum purulence and are moderately or severely ill. Oral corticosteroids can shorten the duration of an exacerbation and NICE3 advise giving 30mg prednisolone for 7 to 14 days.
Perhaps the most difficult aspect of managing an exacerbation of COPD is oxygen therapy. If a COPD patient is hypoxic it is important that they are given sufficient oxygen to correct their hypoxia. Yet giving too little oxygen will not correct hypoxia whilst giving oxygen in excessive concentrations can be dangerous because it can result in some patients retaining carbon dioxide leading to the development of respiratory acidosis, which can be fatal. Careful monitoring of pulse oximetry and arterial blood gas analysis is therefore essential.
If it is not possible to maintain an acceptable arterial oxygen level without causing an increase in carbon dioxide and a drop in arterial pH, non-invasive ventilation (NIV) should be considered. NIV is safer than invasive ventilation, and patients do not need to be managed in an intensive care setting.17 Indeed NICE3 recommend that NIV should be used as treatment of choice for chronic hypercapnic patients during acute respiratory failure despite optimal medical therapy.
Bi-level Positive Airway Pressure or BiPAP is probably the most important mode where management of acute on chronic hypercapnic respiratory failure in COPD is concerned. This Bi-level ventilation delivers a set pressure of air as the patient breathes in, called Inspiratory Positive Airways Pressure (IPAP) and on expiration a lower volume of pressure is delivered called Expiratory Positive Airways Pressure (EPAP). Bi-level therefore alternates between IPAP and EPAP and in doing so it provides assistance during the inspiratory phase of respiration in the form of pressure support, but why would a patient also need EPAP, which effectively means that they breathe out against a pressure that is generated by the ventilator? The answer is that a COPD patient’s airways can collapse, and the delivery of EPAP effectively means that a positive pressure is maintained within the airways during the expiratory phase, helping to inflate them and keep them and the alveoli open.
NIV has transformed the management of an exacerbation of COPD, but it requires specialist knowledge and skills, and it is important that practitioners are competent and appropriately educated.
Blood gas analysis
Blood gas analysis is an essential aspect of the management of an exacerbation of a respiratory condition, and it is an important aspect of preoperative screening, preparation, and perioperative care. A discussion is merited at this juncture.
Arterial blood gases are the most sensitive indicator of respiratory function, particularly the levels of oxygen and carbon dioxide, and can be used to initiate effective treatment. In order to interpret blood gases it is important to understand acid–base balance and the role the lungs and kidneys play in maintaining that balance.
Acids and bases
The pH is a measure of a solution’s acidity or alkalinity and can have a value from 0 to 14. Pure water has a pH of 7 which is neutral, whereas a solution below 7 is acidic, the lower pH value being more acidic. Likewise, a pH of over 7 is alkali with higher pH value being more alkaline.
An acid is a chemical that can release hydrogen ions (H+). The more H+ is in a solution, the more acidic it becomes. A base or alkali can receive or absorb H+. Bicarbonate (HCO3–) is an example of a base and the more that is present in a solution, the more alkaline it becomes.
When carbon dioxide is carried in the blood, some of it combines with water to produce carbonic acid. Carbon dioxide + water = carbonic acid. Expressed as an equation this is:
CO2 + H20 = H2C03
The carbonic acid then dissociates (releases its hydrogen ion into the blood) which causes blood pH to drop. It follows that the more carbon dioxide, in the blood, the more acidic the blood is. If a patient has respiratory problems and retains carbon dioxide they can develop respiratory acidosis. The pH of blood is normally between 7.35 and 7.45 so if a patient has too much carbon dioxide in the blood and the pH is below 7.35 they have developed respiratory acidosis.
It also follows that the less carbon dioxide is in the blood, the less acidic the blood is. So if a patient hyperventilates and there is too little carbon dioxide and therefore too little carbonic acid in the blood the patient will have developed respiratory alkalosis (pH above 7.45).
If a patient has too much carbon dioxide in the blood and therefore too much acid, the body will respond by increasing the respiratory rate and will exhale the carbon dioxide. However, if the patient has a respiratory or other problem, such as a head injury, they may not be able to do this. If this persists over hours or days the kidneys can produce bicarbonate (HCO3–). The bicarbonate stabilises the carbonic acid in the blood; in other words it stops the carbonic acid dissociating and releasing its hydrogen ion. This means that although the patient has too much carbon dioxide in the blood and therefore too much carbonic acid the kidneys have produced bicarbonate which will ensure that the pH will be within normal limits (7.35–7.45) but will not be as high as the upper limit. This situation is called ‘compensated’ respiratory acidosis, because the kidneys have compensated by producing bicarbonate. It takes a number of days for the kidneys to compensate in this way.
Acute respiratory acidosis is characterised by arterial blood gas analysis showing a high PaCO2, a low pH and normal bicarbonate. On the other hand in chronic hypercapnic respiratory failure the arterial blood gas analysis will show a high PaCO2, and unlike acute failure the pH will be within normal limits and the bicarbonate will be high. The pH of blood will have returned to normal limits due to this ‘renal compensation’. Therefore a high bicarbonate level will be evident on blood gas analysis. This is sometimes called ‘compensated respiratory acidosis’. Patients with compensated respiratory acidosis can be clinically stable but will have significant lung disease. Their arterial blood gases will always be abnormal.
Sometimes patients with chronic type II respiratory failure can deteriorate, perhaps due to an exacerbation. When this happens it is called ‘acute on chronic respiratory failure’. This indicates an acute deterioration of a pre-existing chronic hypercapnic respiratory failure. Arterial blood gases show a high PaCO2, low pH and high bicarbonate. In this situation the pH is low despite the high bicarbonate levels. Whilst this may be due to an exacerbation of COPD, it may also be due to injudicious use of oxygen therapy.
Acidosis and alkalosis can also be caused by non-respiratory problems, such as diabetic keto-acidosis. In situations such as this, alterations in pH are not explained by levels of carbon dioxide. For example, in diabetic acidosis the pH will be low but this is not explained by a high carbon dioxide.
Interpretation of blood gases
Investigations only make sense in the context of clinical examination and blood gases may be misleading if relied upon in isolation. It is important to label the sample and complete any forms correctly. Details regarding the patient’s condition should be recorded, especially if they are receiving oxygen therapy. The percentage of oxygen being given needs to be stated, as this will have an effect on the results and subsequent treatment.
Normal values of blood gases vary from hospital to hospital, so it is important to familiarise yourself with the values used in your unit. The table below gives the values used in this chapter.
Table 6.2 Normal values of blood gases
kPa (kilopascals) | mm Hg | |
pH | 7.35–7.45 | |
PaCO2 | 4.7–6.0 | 35–45 |
PaO2 | 11.3–14.0 | 80–100 |
HCO3– | 22–28 mEq/L | 22–28 mEq/L |
Base excess | +/- 2.5 mEq/L | +/- 2.5 mEq/L |
In order to analyse and interpret blood gas results it is preferable to follow a systematic process which involves five steps.
1) Look at the PaO2 (oxygen). This indicates whether the patient is being oxygenated adequately. If below 8.0 kPa the patient is in respiratory failure, and if below 6.7 kPa the patient is dangerously hypoxic.
2) Look at the pH. Is it acid or alkali?
3) Look at the PaCO2 (carbon dioxide). Could this explain a low or raised pH? CO2 combines with H2O to form carbonic acid, so a high CO2 would explain a low pH, and a low CO2 would explain a high pH. If the PaCO2 explains why the pH is high or low, then the problem is likely to be respiratory in origin. If it does not, then the problem is likely to be ‘metabolic’, or non-respiratory.
4) Look at the HCO3– (bicarbonate). Could this explain a change in pH? A high bicarbonate may explain a high pH, and a low bicarbonate may explain a low pH. If changes in bicarbonate levels explain pH the problem is likely to be metabolic, or non-respiratory.
5) Assess for compensation. For example, the kidneys can compensate for respiratory acidosis by producing bicarbonate, which would mean that pH may be within normal limits despite a high PaCO2. Similarly, the lungs can compensate for metabolic acidosis by ‘blowing off’ CO2 resulting in a low PaCO2.
A COPD patient with compensated respiratory acidosis might have blood gas results similar to the example below.
pH | 7.37 |
PaCO2 | 8.29 kPa |
PaO2 | 7.9 kPa |
HCO3– | 34 mEq/L |
Using the steps to analyse the sample:
1. Look at the PaO2: it is low, but is there adequate oxygenation for a person with COPD?
2. Look at the pH: it is in the lower range of normal.
3. Look at the PaCO2: it is high, indicating a respiratory acidosis.
4. Look at the HCO3: it is high, so the acidosis is not due to metabolic problems.
5. Assess for compensation: the HCO3– is high and the pH is within normal range; therefore a compensated respiratory acidosis is indicated.
Obstructive sleep apnoea
Obstructive sleep apnoea is thought to result from decreased upper airway patency in sleep compared to wakefulness. The airway partially occludes; arousals from sleep can restore airway patency but this is followed by a repetitive cycle of airway collapse and arousal. Patients may be at risk of complications related to anaesthesia and postoperative analgesia. Indeed it may be a risk factor for anaesthetic morbidity and mortality.18 This may be because anaesthetic and narcotic agents can increase the tendency for airway collapse and they may also impair normal arousal, thus worsening apnoea severity.19
There is a positive correlation between obstructive sleep apnoea severity and complication rate, but even sleep apnoea which may not be otherwise significant may become dangerous under the influence of anaesthetic or analgesia, or with oedema of the airway from intubation.20
Unfortunately patients may not have a diagnosis of obstructive sleep apnoea prior to surgery, and clinical presentation alone may be a poor predictor. Nevertheless preoperative evaluation should include assessment of the likelihood of obstructive sleep apnoea. If suspected, preoperative consultation with a sleep specialist is appropriate but if surgery cannot be postponed patients may require CPAP postoperatively when the endotracheal tube is removed.20 The first 24 postoperative hours are probably the most critical time, but deaths can occur later. This should be considered when prescribing analgesia21 and consideration should be given to the potential synergistic effects of medications with regard to central nervous system depression.
The necessity of identifying patients with respiratory problems
Patients with respiratory disease have an increased chance of developing complications postoperatively.2 This may be due to specific respiratory disorders, or problems may be caused by shallow breathing, poor lung expansion, and infection.
To minimise the risk of complications, respiratory patients should be identified preoperatively, and their lung function should be improved as much as possible by providing optimum treatment for their disorder, and interventions such as physiotherapy can help teach patients in the preoperative period to participate with techniques in the postoperative phase. This can help to mobilise secretions and increase lung volumes in the postoperative period, which can reduce pulmonary complications.2 Postoperative complications can be minimised by treating respiratory conditions prior to surgery. Respiratory infection should be treated and resolved, and asthma should be well controlled. Before surgery, patients should be free of wheeze and ideally have a peak flow reading of above 80% or the patient’s personal best value. Inhaled steroid dose may have to be increased or oral steroids commenced one week prior to surgery.2,22 Elective surgery should be postponed until the patient is ready.
Smoking cessation is also an important aspect of preoperative preparation. According to Sharma,22 patients who smoke have a two-fold increase in risk of postoperative complications even in the absence of a diagnosed disease; however, patients who quit smoking for 6 months have a risk similar to those who do not smoke. The risk of pulmonary complications following major surgery in smokers is estimated to be six times higher than that in the non-smoking population.23
The airways of smokers have poor muco-ciliary clearance of secretions, and they are at increased risk of postoperative complications such as pneumonia. Even abstinence for the 12 hours before anaesthesia will allow time for clearance of nicotine, a coronary vasoconstrictor, and a fall in the levels of carboxyhaemoglobin, thus improving oxygen carriage in the blood.2
Preoperative assessment is therefore essential, and full pulmonary function tests are sometimes warranted. Clearly this is very much dependent on the patient’s condition and the nature of the surgery, and there is no definitive guide that will give reliable ‘cut off’ points which would prevent patients from receiving surgery. This is definitely depends on the clinical judgement of the surgeon, anaesthetist and other specialists.
Lung cancer is an illustrative example. Clearly if a patient is to receive surgery which will remove part of their lungs and reduce their FEV1, preoperative FEV1 is important because if it is already low, any further reduction due to surgery could be significant. Because of this it is important to calculate an ‘Estimated Post Operative FEV1’ (EPOFEV1). Techniques to calculate this vary considerably, and include simply counting the number of lung segments to be removed, or inserting the number of lung segments that are obstructed (a) and the number of unobstructed segments (b) into a formula. The EPOFEV1 = pre FEV1 x [(19 – a) – b]/19 – c where c = the total number of segments in the lung. The right upper lobe has three segments, middle has two, right lower has five, left upper three, lingual two and left lower has four.
For general surgery, a low FEV1 might result in low postoperative survival. An FEV1 of FVC less than 70% predicted, or a ratio of less than 65%, is associated with an increased risk of pulmonary complications2 and COPD patients with an FEV1 of less than 40% predicted are six times more likely to have a major postoperative complication. Yet it is not possible to state that there is a prohibitive level of pulmonary function for an absolute contradiction to surgery, and the clinical decision will depend on a range of factors.22
Spirometry alone is not a good prognostic factor1,24 and other things are important, such as age, nutrition, hypoxia, general condition – therefore full assessment is needed. The procedure itself is of course important. For example, preoperative hypoxia or carbon dioxide retention indicates the possibility of postoperative respiratory failure which may require a period of assisted ventilation postoperatively2 and chest X-rays may identify collapse, consolidation, infection, pulmonary oedema, or hyperinflated lungs. With some respiratory conditions, such as COPD, pre-op arterial blood gas analysis is recommended to show severity of hypoxia and hypercapnia.23
Postoperative care is complicated, and much of this chapter can be applied to the postoperative management of any patient with a respiratory condition. Clearly it should be tailored to the individual patient and their situation; some patients may require high flow oxygen, but other patients may require carefully controlled, titrated oxygen which is adjusted depending on blood gas analysis. Patients should be closely monitored, and COPD patients will require postoperative oxygen therapy that should aim to maintain arterial oxygen level without inhibiting respiration, thereby preventing respiratory acidosis.23
Postoperatively the patient may develop bronchospasm. The patient may be breathless, have a rapid respiratory rate, and may be using their accessory muscles. An expiratory wheeze may be audible on auscultation, but a quiet chest may be ominous as it may mean that very little airflow is taking place. Treatment may be similar to that discussed in this chapter, including nebulised bronchodilators, titrated oxygen and intravenous steroids.
Above all it is important to appreciate that there are no rigid rules that can be applied to the management of all respiratory patients perioperatively. Each patient’s situation is unique and perioperative management should be agreed in consultation with the anaesthetist and the specialist team.
REFERENCES
1. R. Larsen (2003). Anestezjojogia. Wroclaw: Urban and Partner. MRC (1981). Long term domiciliary oxygen therapy in chronic hypoxic cor pulmonale complicating chronic bronchitis and emphysema.
2. M. Mercer (2000). Anaesthesia for the patient with respiratory disease. Practical Procedures 12: 1–17.
3. NICE (2004). Chronic Obstructive Pulmonary Disease. National clinical guideline on management of chronic obstructive pulmonary disease in adults in primary and secondary care. Thorax 59 (Suppl 1): 1–232.
4. C.M. Fletcher and R. Peto (1976). The natural history of chronic airflow obstruction. British Medical Journal 1: 1645–8.
5. GOLD (2004). Global Initiative for Chronic Obstructive Pulmonary Disease. http://www.goldcopd.com/ (last accessed February 2010).
6. P.J. Barnes (2000). Mechanisms in COPD: differences from asthma. Chest 117: 10S–14S.
7. MRC (1981). Long term domiciliary oxygen therapy in chronic hypoxic cor pulmonale complicating chronic bronchitis and emphysema. Lancet 1: 681–6.
8. NOTT (1980). Continuous or nocturnal oxygen therapy in hypoxaemic chronic obstructive lung disease. Annals of Internal Medicine 93: 391–8.
9. P.S. Burge, P.M.A. Calverley and P.W. Jones (2000). Randomised, double-blind, placebo controlled study of fluticasone propionate in patients with moderate to severe chronic obstructive pulmonary disease: the ISOLDE trial. British Medical Journal 320: 1297–1303.
10. P.M.A. Calverley, R. Pauwels, J. Vestbo, P. Jones, N. Pride and A. Gulsvik (2003). Combined salmeterol and fluticasone in the treatment of chronic obstructive pulmonary disease: a randomised controlled trial. Lancet 361: 449–56.
11. D.D. Sin, F.A. McAlister, P. Man and N.R. Anthonisen (2003). Contemporary management of chronic obstructive pulmonary disease: Scientific review. Journal of the American Medical Association 290: 2301–12.
12. W. Szafranski, A. Cukier, A. Ramirez, G. Menga, R. Sansores, S. Nahabedian, S. Peterson and H. Olsson (2003). Efficacy and safety of budesonide/formoterol in the management of chronic obstructive pulmonary disease. European Respiratory Journal 21: 74–81.
13. P. Kardos, M. Wencker, T. Glaab and C. Vogelmeier (2007). Impact of salmeterol/fluticasone versus salmeterol on exacerbations in severe chronic obstructive pulmonary disease. American Journal of Critical Care Medicine 175: 144–9.
14. P.M.A. Calverley, J.A. Anderson, B. Celli, J. Vestbo et al (2007). Salmeterol and fluticasone propionate and survival in chronic obstructive pulmonary disease. New England Journal of Medicine 356: 775–89.
15. P.J. Poole and P.N. Black (2006). Mucolytic agents for chronic bronchitis or chronic obstructive pulmonary disease. Cochrane Database of Systematic Reviews, Issue 3. Art no.: 001287. DOI: 10.1002/14651858.CD001287.pub2.
16. F.S.F. Ram, P.W. Jones, A.A. Castro, J.R. De Brito Jardim, A.N. Atallah, Y. Lacasse, R. Mazzini, R. Goldstein and S. Cendon (2002). Oral theophylline for chronic obstructive pulmonary disease. Cochrane Database of Systematic Reviews, Issue 3. Art. No.: CD003902. DOI: 10.1002/14651858.CD003902.
17. British Thoracic Society (2001). Guidelines on the selection of patients with lung cancer for surgery. Thorax 56: 89–108.
18. J.A. Loadsman and D.R. Hillman (2001). Anaesthesia and sleep apnoea. British Journal of Anaesthesia 86: 254–66.
19. R.W. Robinson, C.W. Zwillich, E.O. Bixler, R.J. Cadieux, A. Kales and D.P. Whide (1987). Effects of oral narcotics on sleep disordered breathing in healthy adults. Chest 91: 197–203.
20. A.M. Meoli, C.L. Rosen, D. Kristo, M. Kohrman, N. Gooneratne, R. Aguillard, R. Fayle, R. Troell, R. Kramer, K. Casey and J. Coleman (2003). Upper airway management of the adult patient with obstructive sleep apnea in the perioperative period – avoiding complications. Sleep 26(8): 1060–5.
21. D.J. Cullen (2001). Obstructive sleep apnea and postoperative analgesia – a potentially dangerous combination. Journal of Clinical Analgesia 13: 83–5.
22.S. Sharma (2006). Perioperative pulmonary management. www.emedicine.com/med/topic3169.htm (last accessed February 2010).
23. K. Rutkowska, H. Misiolek, H. Kucia and P. Knapic (2006). Perioperative management of COPD patients undergoing nonpulmonary surgery. Anaesthesiology Intensive Therapy 38: 153–7.
24. D.H. Wong, E.C. Weber, M.J. Schell and A.B. Wong (1995). Factors associated with postoperative pulmonary complications in patients with severe COPD. Anesthesia and Analgesia 80: 276–84.

Full access? Get Clinical Tree
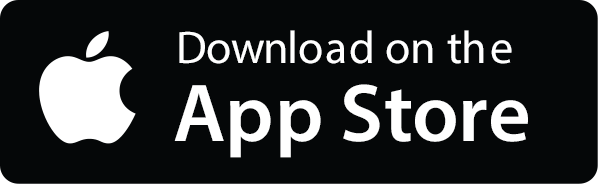
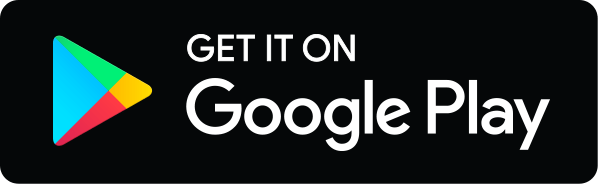